and Peter J. Millett2
(1)
Steadman Philippon Research Institute, Vail, CO, USA
(2)
The Steadman Clinic, Steadman Philippon Research Institute, Vail, CO, USA
Keywords
ShoulderAcromioclavicularAC jointCoracoclavicularSeparationDislocationPhysical examClinical exam7.1 Introduction
When compared to other areas of the shoulder, physical examination of the AC joint is more straightforward given its subcutaneous location—a factor that facilitates inspection, palpation and, in some cases, pain localization. In addition, many of the provocative maneuvers used to diagnose AC joint pathologies are more reliable due to the limited potential for confounding variables. However, even if the AC joint is the most likely source for the patient’s pain, the inciting etiology is not always clear-cut. A thorough understanding of the anatomy, biomechanics, radiographic features, and relevant physical examination findings related to the AC joint will ultimately contribute to the generation of an accurate diagnosis, an effective treatment plan, and a successful clinical outcome.
7.2 Anatomy and Biomechanics
The AC joint represents a complex articulation between the distal clavicle and the anteromedial aspect of the acromion and plays an important role in the coordination shoulder motion and force transmission between the shoulder girdle and the axial skeleton (Fig. 7.1) [1, 2]. Because the clavicle is linked to the acromion, three-dimensional scapular motion requires that the clavicle also be capable of three-dimensional motion (Fig. 7.2) [3]. Therefore, the AC joint must be flexible enough to allow for acromioclavicular motion while also being stiff enough to confer stability. Unconstrained osseous anatomy, capsular and extra-capsular ligamentous structures, and dynamic muscle contraction function in harmony to maintain the balance between mobility and stability across the AC joint throughout the entire range of shoulder motion.
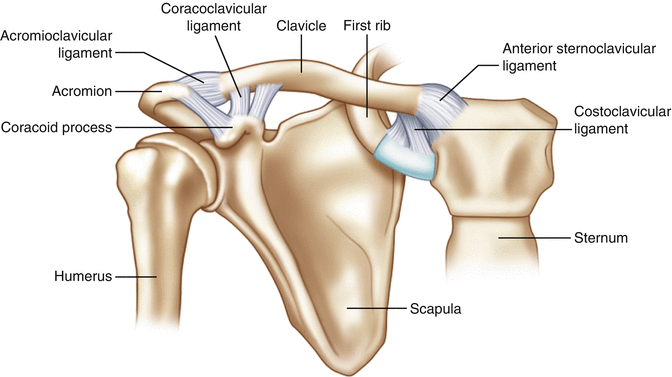
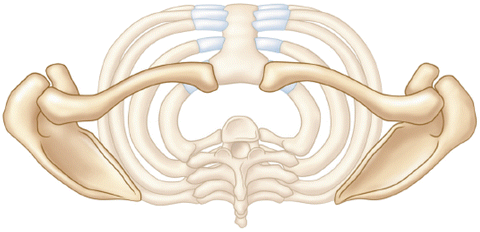
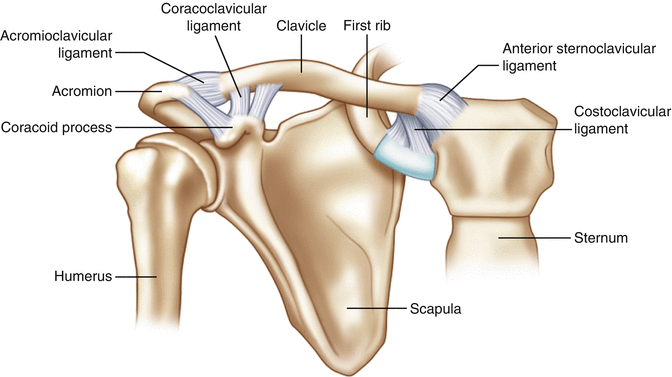
Fig. 7.1
Illustration showing the basic osseoligamentous anatomy of the shoulder girdle. The AC joint is the central coordinator of three-dimensional shoulder motion and is responsible for the transmission of forces between the axial skeleton and the glenohumeral joint.
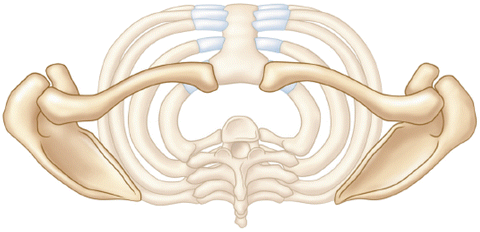
Fig. 7.2
Illustration highlighting the spatial relationship between the scapula, clavicle, and sternum. Note that scapular motion in any plane requires a coordinated motion to occur across both the AC and SC joints.
7.2.1 Osseous Anatomy
The clavicle is an S-shaped bone that develops from three separate ossification centers via an intramembranous ossification mechanism beginning during the fifth gestational week [4]. Complete ossification of the clavicle does not occur until at least 25 years of age and physeal fusion may be delayed until 31 years of age [5–7]. Similarly, the acromion has four centers of ossification which fuse together by approximately 18 years of age [8]. The fusion sites of these ossification centers are referred to as the preacromion, mesoacromion, and meta-acromion (Fig. 7.3). In approximately 7 % of individuals, one or more of these ossifications centers may fail to fuse, producing a defect known as os acromiale which is primarily diagnosed with radiographs (Fig. 7.4) [9]. Bone scans can also be used to detect increased metabolism of the bony fragment which is closely correlated with the presence of pain (Fig. 7.5). Because the symptoms related to os acromiale can mimic those of other painful AC joint pathologies, patients who present with pain at the top of the shoulder should always be evaluated for the possibility of os acromiale [8]. Os acromiale is also frequently associated with rotator cuff impingement since the deltoid muscle can pull the loosely attached bone downward with arm elevation, thus decreasing the volume of the subacromial space (Fig. 7.6) [8].
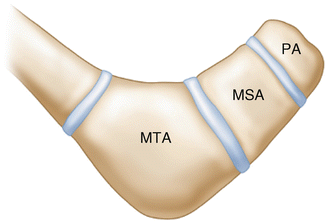
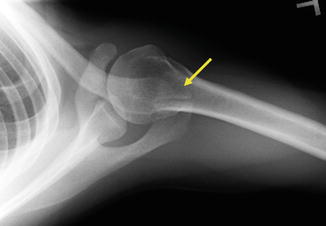
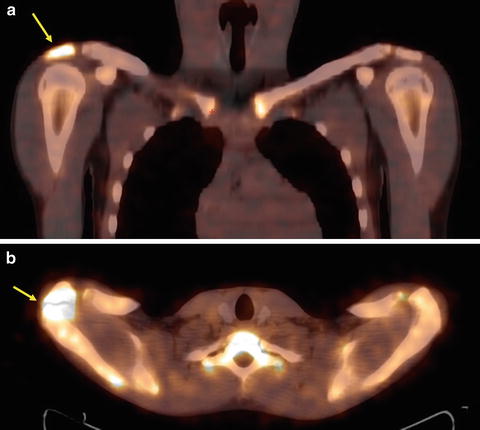
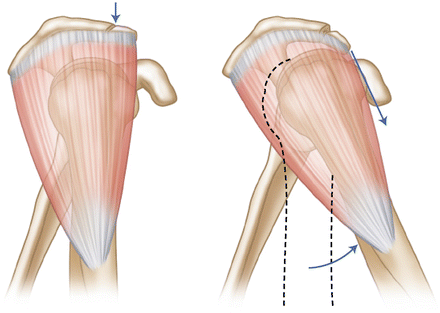
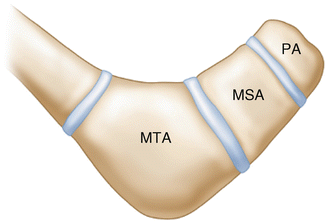
Fig. 7.3
Illustration showing the fusion sites for each ossification center of the acromion. The preacromion, mesoacromion, and meta-acromion are shown. Os acromiale occurs when two of the ossification centers fail to fuse together, sometimes leading to a pseudarthrosis.
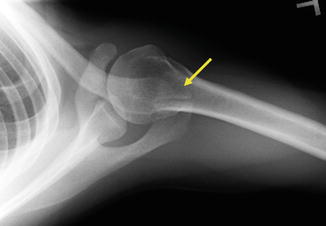
Fig. 7.4
Axillary radiograph of a left shoulder in a patient with symptomatic os acromiale.
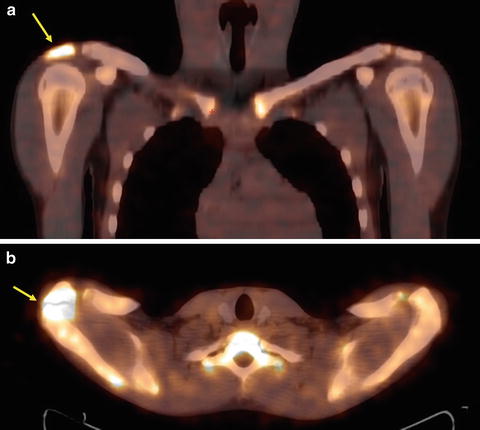
Fig. 7.5
Positron emission tomography (PET) scan in a patient with symptomatic os acromiale of the right shoulder. (a) The coronal sequence demonstrates increased uptake of the radioactive tracer at the site of the os acromiale, suggesting that the patient’s pain was most likely related to the os acromiale itself instead of another underlying pathology. (b) The axial sequence confirms that the increased uptake did, in fact, involve the mesoacromion.
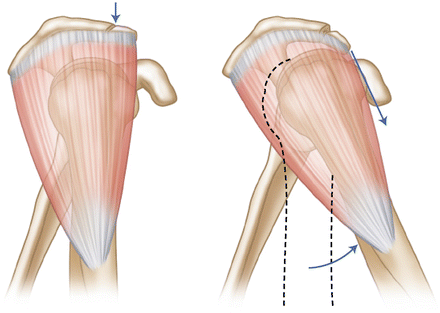
Fig. 7.6
Illustration depicting a possible mechanism of rotator cuff impingement in patients with os acromiale. The downward pull that the deltoid imparts upon the acromion displaces the unfused bony fragment downward, thus decreasing the space available for the rotator cuff tendons to pass beneath the acromion as the humerus is elevated.
Overall, the mean size of the AC joint is approximately 9 mm × 19 mm [10]. However, the size and shape of the distal clavicle and acromion can vary widely across the population [11, 12]. The inclination of the joint surfaces may also be highly variable [13]. When viewed from anteriorly, the joint line can range from a vertical orientation to nearly 50° of angulation where the articular surface of the distal clavicle overrides that of the acromion (Fig. 7.7) [14, 15]. Some AC joints may have an “ellipsoid” shape that may limit internal and external scapular rotation, thus elevating the risk for subacromial impingement [16]. In most cases, the articular surface of the acromion is concave whereas the articular surface of the distal clavicle is convex [13]; both of these surfaces are initially covered in hyaline cartilage (either completely or partially [17]) followed by a slow transformation to fibrocartilage beginning in adolescence [18]. Rockwood et al. [19] stated that this transition to fibrocartilage is completed by ages 17 and 24 on the acromial and clavicular joint surfaces, respectively.
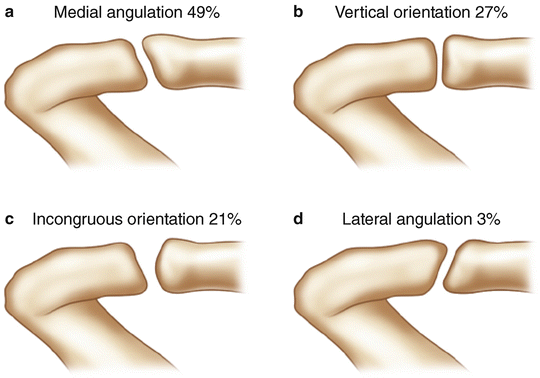
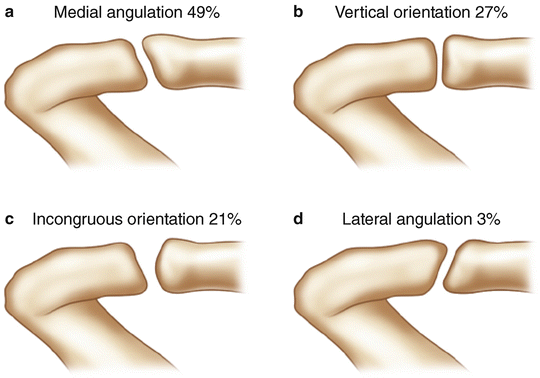
Fig. 7.7
Illustrations showing the variable angulation of the AC joint surfaces when viewed from anteriorly. (a) Medial angulation occurs in approximately 50 % of cases, (b) a vertical joint line occurs in approximately 25 % of cases, (c) an incongruent joint occurs in approximately 25 % of cases, and (d) lateral angulation occurs in approximately 5 % of cases.
7.2.2 Intra-Articular Disk
An intra-articular disk (i.e., meniscal homologue) of widely variable shapes and sizes attaches firmly to the acromial side of the joint (Fig. 7.8). The disk functions to disperse the forces associated with joint loading and corrects for any joint incongruency. The upper portion of the disk is made up of fibrocartilage whereas the lower portion of the disk is primarily made up of dense fibrous tissue, possibly reflecting differential load-bearing across the AC joint [16, 20]. Normal degeneration of the intra-articular disk begins during adolescence and progresses through the fourth decade, at which point the disk has become a vestigial structure [21–23]. Degeneration of the intra-articular disk has been associated with osteoarthritis of the AC joint; however, this finding is inevitable since disk degeneration appears to be nearly universal in normal adults.
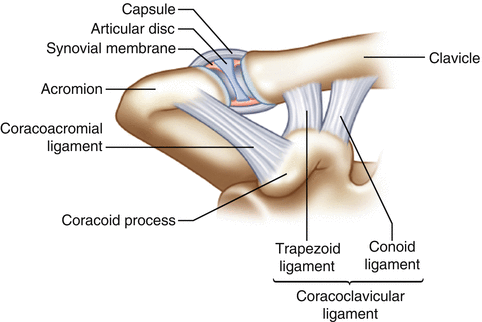
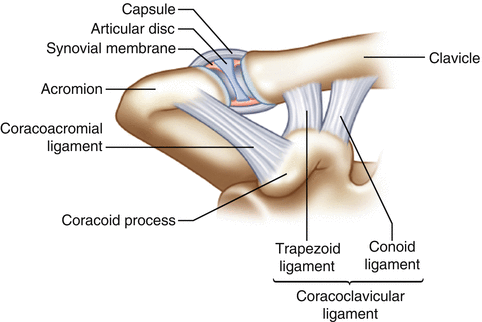
Fig. 7.8
Illustration highlighting the intra-articular structure of the AC joint. The intra-articular disk typically adheres to the acromial side of the joint. The AC joint capsule inserts between 3 and 5 mm lateral to the acromial articular surface and between 3 and 6 mm medial to the clavicular articular surface [24].
7.2.3 Capsular and Extra-Capsular Ligaments
The AC joint is surrounded by a fibrous joint capsule with inward-facing synovial tissue that facilitates joint mobility by providing adequate lubrication (see Figs. 7.1 and 7.8). The joint capsule inserts between approximately 3 and 5 mm lateral to the acromial articular surface and between approximately 3 and 6 mm medial to the clavicular articular surface [24]. This capsule is reinforced by closely integrated and confluent AC joint ligaments (superior, inferior, anterior, and posterior), the combined actions of which primarily resist horizontal displacement, accounting for up to 50 and 90 % of anterior and posterior stability of the distal clavicle, respectively [25–27]. More specifically, the superior AC ligament is thought to contribute up to 90 % of the overall strength of the AC joint capsule [28]. In addition, the fibers of the deltotrapezial fascia merge and coalesce with those of the superior AC ligament, thus providing even more structural reinforcement. In contrast, the anterior, posterior, and inferior AC ligaments have significantly less tensile strength [28]. In general, the AC joint capsular ligaments are responsible for resisting relatively small horizontal translations of the distal clavicle relative to the acromion [26].
On the other hand, the coracoclavicular (CC) ligaments are primarily responsible for preventing large translations of the distal clavicle relative to the acromion [26]. The CC ligaments include the conoid (centromedial) and the trapezoid (anterolateral) ligaments which travel between the inferior surface of the distal clavicle and the base of the coracoid process (Fig. 7.9). The conoid and trapezoid ligaments insert approximately 32.1 and 14.7 mm medial to the articular surface of the distal clavicle, respectively [24]. The conoid ligament is the primary restraint to excessive superior translation and rotation of the distal clavicle whereas the trapezoid ligament helps prevent excessive anterior–posterior translation of the distal clavicle and compression of the AC joint [17, 26, 27]. The CC ligaments assume complete responsibility for both horizontal and vertical stability when the AC joint capsule has been disrupted [26, 29]. Therefore, dislocation of the AC joint is not possible unless disruption of the CC ligaments has occurred [18].
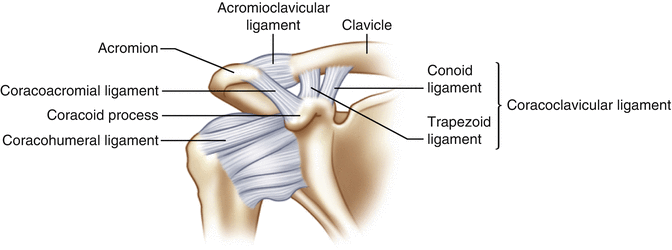
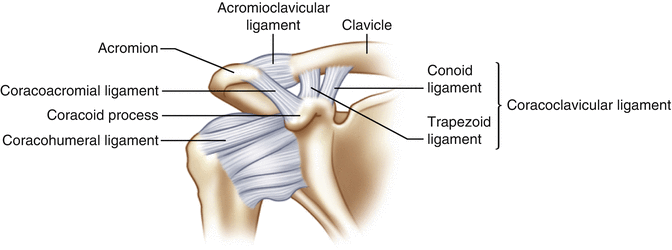
Fig. 7.9
The coracoclavicular (CC) ligaments are composed of the conoid and trapezoid ligaments. The conoid ligament travels between the coracoid base and the conoid tubercle which is centrally located on the inferior aspect of the clavicle. The trapezoid ligament runs anterolateral to the conoid ligament and inserts approximately 15 mm medial to the articular surface of the distal clavicle. The CC ligaments are responsible for preventing large displacements of the distal clavicle relative to the acromion.
7.2.4 Dynamic Stability
Dynamic stability of the distal clavicle is provided by the serratus anterior, upper trapezius, and anterior deltoid (see Chap. 3 for a more detailed anatomic description of these muscles). Of note, the upper trapezius inserts into the posterosuperior aspect of the distal clavicle and the medial acromion and its fibers blend into those of the superior AC joint ligament. The serratus anterior and upper trapezius function in synchrony to optimize the three-dimensional position of the scapula which facilitates glenohumeral stability and supports a large arc of motion. The deltoid primarily functions to support the weight of the arm, thus reducing articular shear forces and reducing tension across the AC joint capsuloligamentous structures [30].
7.2.5 Neurovascular Anatomy
The AC joint capsule receives its blood supply from small branches derived from the suprascapular and thoracoacromial arteries. The joint is innervated by the suprascapular nerve just before it passes beneath the transverse scapular ligament (the suprascapular artery travels with the nerve and passes above the transverse scapular ligament). Branches of the lateral pectoral nerve which travel with the thoracoacromial artery also provide some joint innervation (Fig. 7.10).
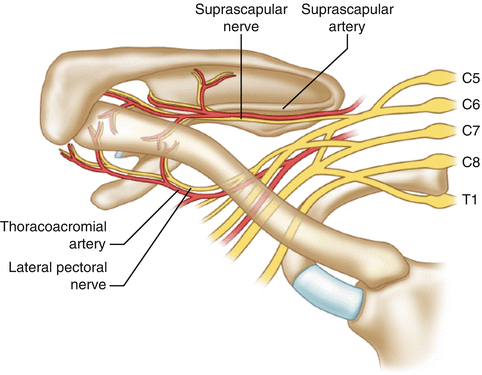
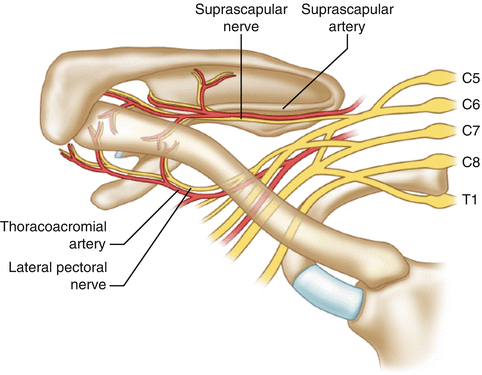
Fig. 7.10
Illustration of the neurovascular anatomy that supplies the AC joint. The suprascapular nerve and lateral pectoral nerve provide the innervation while the suprascapular artery and thoracoacromial artery provide the blood supply.
7.2.6 Joint Motion
To achieve normal shoulder motion, the AC joint must be able of coordinating the unique three-dimensional motion planes of both the scapula and the clavicle (Fig. 7.11) [1, 2]. Shoulder elevation requires long-axis rotation (up to 50° [31–34]), elevation (up to 15° [35]), and posterior angulation (i.e., retraction.; up to 30° [35]) of the clavicle relative to its resting position; however, the AC joint itself may only be responsible for a portion of this motion where sternoclavicular (SC) joint motion makes up the majority of this difference [35]. More specifically, Ludewig et al. [35] found that approximately 31° of posterior longitudinal rotation of the clavicle occurred at the SC joint whereas about 19° of posterior scapular tilt was allowed across the AC joint. Disruption of the AC joint following an acute injury can therefore result in uncoordinated scapular motion and global shoulder dysfunction (scapular dyskinesis following an AC joint injury is discussed in further detail in Chap. 9).
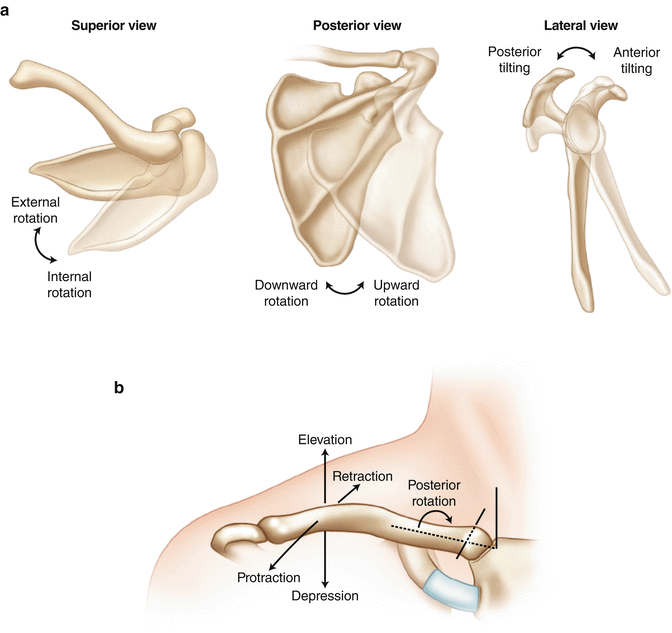
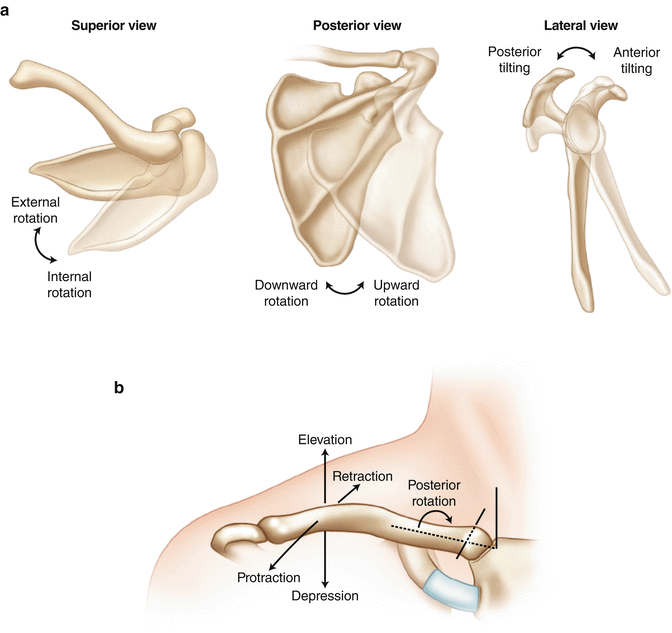
Fig. 7.11
Illustrations highlighting the three-dimensional movements of the clavicle and the scapula. Note that any scapular motion requires force transmission through the AC joint, leading to clavicular motion and, therefore, SC joint motion. (a) The three-dimensional motion planes of the scapula. The kinetic energy from these motions travels through the AC joint thus resulting in clavicular motion. (b) The three-dimensional motion planes of the clavicle that are closely coordinated with scapular motion through both mechanical and neuromuscular stimuli.
Relative positional changes of the scapula and the clavicle also require a small amount of joint translation—up to 6 mm of translation in any direction [29, 36]. Given the small articular surface area and the high compressive and shear forces that are applied to the joint with daily activities, the AC joint is susceptible to locally elevated contact stresses which may favor the development of osteoarthritis [13].
7.3 Instability of the Acromioclavicular Joint
7.3.1 Pathogenesis
The vast majority of injuries to the AC joint occur during contact sports following an impact to the lateral shoulder with the arm in an adducted position (Fig. 7.12). Although much less common, other types of injury mechanisms such as a direct blow to the distal clavicle or a fall onto an outstretched hand (driving the humeral head into the acromion and producing an inferior dislocation) are also possible.
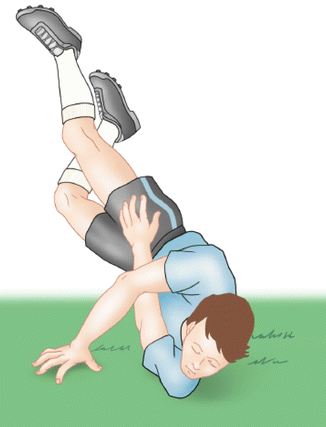
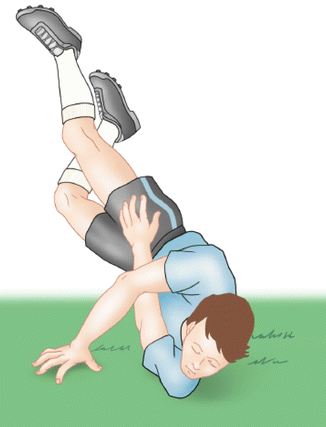
Fig. 7.12
Illustration of the most common injury mechanism resulting in acute AC joint dislocations. Direct impact to the superolateral aspect of the shoulder forces the acromion inferiorly leading to rupture of the AC joint capsule and CC ligaments, thus allowing the distal clavicle to translate superiorly.
7.3.2 Physical Examination
7.3.2.1 Acute AC Joint Injuries
Patients with acute AC joint injuries will complain of pain at the top of the shoulder following a significant impact-type injury to the lateral shoulder. Examination of the patient in the sitting or standing position allows the weight of the arm to pull the scapula downward, thus exaggerating the deformity (if present). Inspection of the shoulder usually reveals swelling surrounding the area of the AC joint. In cases of higher-grade injuries, an obvious step-off deformity may be present (Fig. 7.13). Patency of the deltotrapezial fascia can be assessed by having the patient shrug their shoulders—spontaneous joint reduction with this maneuver indicates that the deltotrapezial fascia is intact (discussed below for grades III and V injuries). The clinician should assess for a concomitant clavicle fracture by palpating the entire length of the clavicle, beginning at the SC joint and moving towards the AC joint.
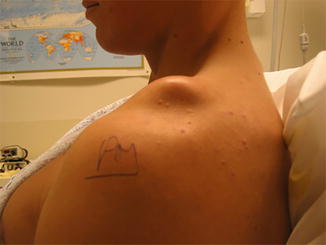
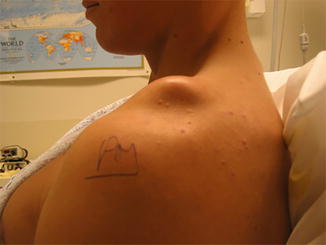
Fig. 7.13
Clinical photograph of a patient’s left shoulder following an acute AC joint dislocation. This patient had a grade V injury.
In most cases, these general physical findings lie along a spectrum of severity that are closely related to radiographic findings. The Zanca view is most often used to evaluate injuries to the AC due to its accuracy, excellent diagnostic utility, and its ability to identify concomitant clavicle fractures which can sometimes mimic an AC joint dislocation, especially in younger patients with open physes [37, 38]. To obtain this view, the X-ray beam is centered on the AC joint and tilted 10–15° cephalad [39]. It is recommended to decrease the X-ray penetrance by approximately 50 % to improve visibility of the coracoid, distal clavicle, and other surrounding structures [17]. Many practitioners prefer to obtain a Zanca view that includes both shoulders in order to compare the amount of distal clavicle displacement between the injured and non-injured shoulders (Fig. 7.14). To objectively assess the amount of distal clavicle displacement, the CC distance can be measured and compared between shoulders. Using the same Zanca radiograph, the CC distance is determined by the length of a vertical line that begins from the most superior point of the coracoid and ends at the most inferior point of the clavicle (Fig. 7.15). Although “normal” CC distances have been reported, these raw measurements are highly variable between individuals due to variations in anatomy. Therefore, comparisons should be made using a ratio in which the CC distance of the injured shoulder is compared to that of the non-injured shoulder. Bearden et al. [40] suggested that a 25 % increase in the CC distance of the injured shoulder probably represents complete disruption of the CC ligaments. Pre- and postoperative Zanca radiographs of the same patient are helpful to ensure maintenance of joint reduction following operative management of an AC joint injury. Of importance, a normal-appearing CC distance in a patient with signs, symptoms, and historical features characteristic of an AC joint dislocation should generate suspicion of a concomitant fracture of the coracoid with superior displacement. When a coracoid fracture is suspected, a Stryker notch view should also be obtained. In this radiographic view, the patient lies supine, places the hand of the affected extremity on top of the head, and the technician directs the X-ray beam towards the coracoid process with approximately 10° of cephalad angulation (Fig. 7.16).
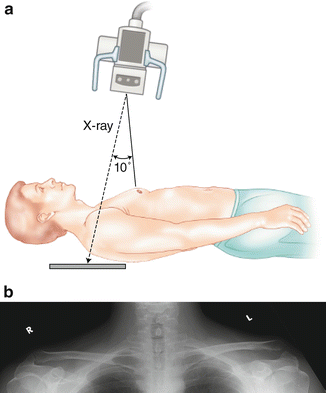
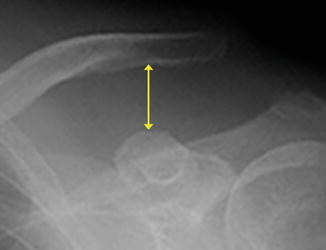
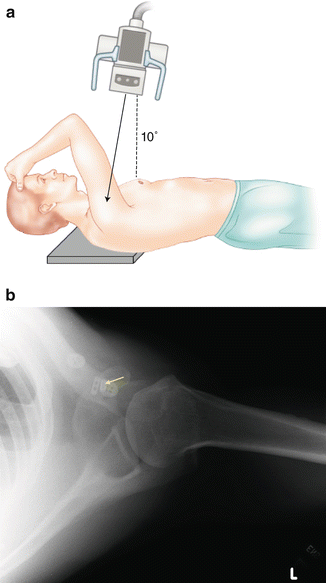
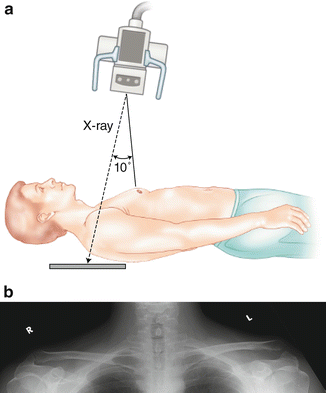
Fig. 7.14
(a) Illustration of the technique used to obtain a Zanca radiograph. The X-ray beam is aimed directly towards the AC joint with 10–15° of cephalad angulation. The X-ray beam can also be centered on the midline with cephalad angulation to obtain an image that includes the bilateral AC joints. (b) Example of a Zanca radiograph in a patient with an acute grade III AC joint dislocation of the left shoulder.
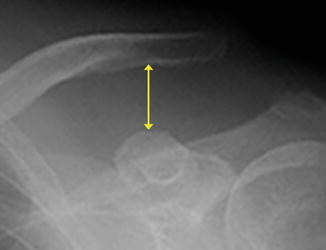
Fig. 7.15
Measurement of the CC distance using a Zanca radiograph. A vertical line is drawn connecting the most superior point of the coracoid to the most inferior point of the distal clavicle. The length of the vertical line represents the CC distance.
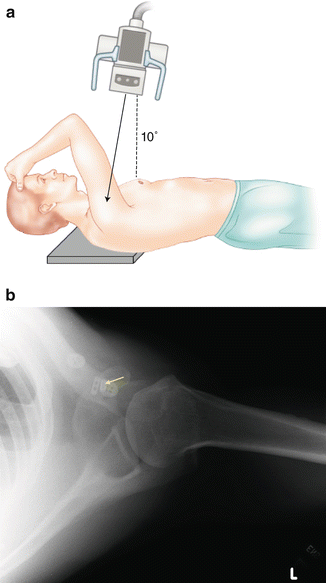
Fig. 7.16
(a) Illustration of the technique used to obtain a Stryker notch view. With the patient supine and the hand of the affected extremity placed on top of the head, the X-ray beam is aimed towards the coracoid process with approximately 10° of cephalad angulation. (b) Example of a Stryker notch view obtained in a patient with a suspected coracoid fracture following AC reconstruction. The patient presented with loss of reduction following a skiing fall. The image demonstrates displacement of a cortical fixation button that was placed through a drill hole in the coracoid to reduce the initial dislocation (arrow).
Although less common, posterior dislocations of the AC joint can also occur and may appear normal on standard Zanca radiographs in some cases. Therefore, in all cases of AC joint instability, clinicians should also obtain an axillary view (or Velpeau axillary view) of the shoulder to identify posterior displacement of the distal clavicle relative to the acromion (Figs. 7.17 and 7.18).
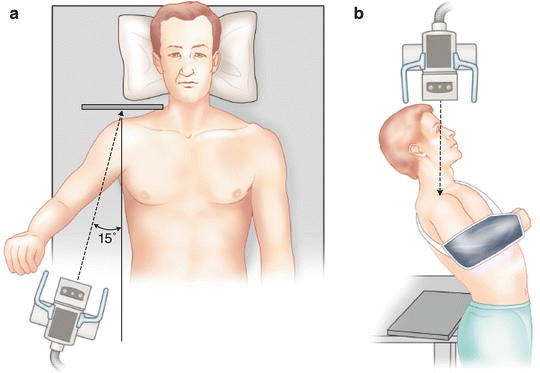
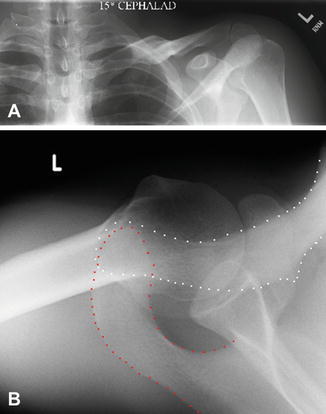
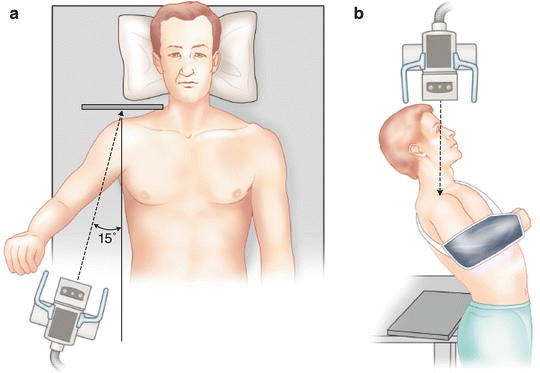
Fig. 7.17
(a) The axillary view is obtained with the patient supine (or standing) and the X-ray cassette positioned above the injured shoulder. The shoulder must be sufficiently abducted to allow the X-ray beam to pass between the humerus and the thorax. The X-ray tube is positioned inferior to the shoulder and aimed directly towards the glenohumeral joint at approximately half the angle of abduction (e.g., an abduction angle of 30° would require the X-ray tube to be positioned approximately 15° lateral to the midline). This method ensures that the axillary radiograph is obtained within the plane of the glenoid. (b) The Velpeau axillary view is used when the patient cannot adequately abduct the arm to obtain the standard axillary view. While wearing a sling (e.g., Velpeau dressing), the patient is asked to lean backwards to approximately 30° over the X-ray table and cassette. The X-ray beam is directed vertically downward towards the cassette.
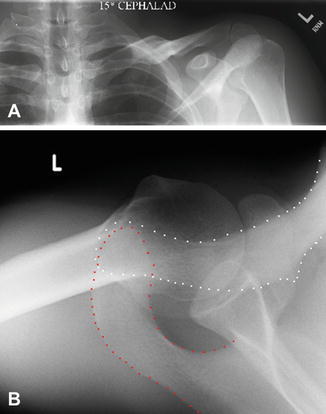
Fig. 7.18
(a) Normal-appearing Zanca radiograph of the left AC joint in a patient with pain at the top of the shoulder following an acute injury. Note the normal alignment between the distal clavicle and the acromion. (b) Axillary radiograph of the same shoulder demonstrating posterior displacement of the distal clavicle (outlined in white) relative to the acromion (outlined in red) that was not detectable on the Zanca view.
Tossy et al. [41] and Rockwood [42] developed a classification system for AC joint injuries based on anteroposterior (AP), Zanca or axillary radiographs (types I–VI, described below [Fig. 7.19]). This classification system is closely related to injury severity which, in turn, is closely related to physical examination findings. However, it should be noted that up to 30 % of patients with an acute AC joint injury are likely to have concomitant intra-articular injuries [43–45]. Therefore, the clinical examination should involve an evaluation of the entire shoulder girdle in all cases to identify potentially treatable concomitant injuries.
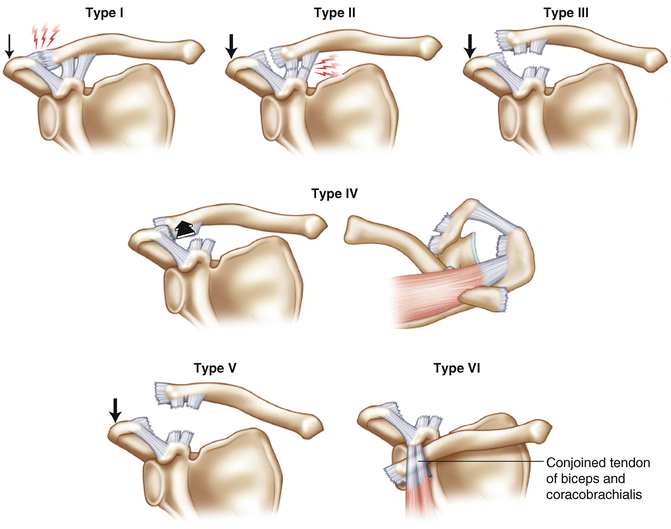
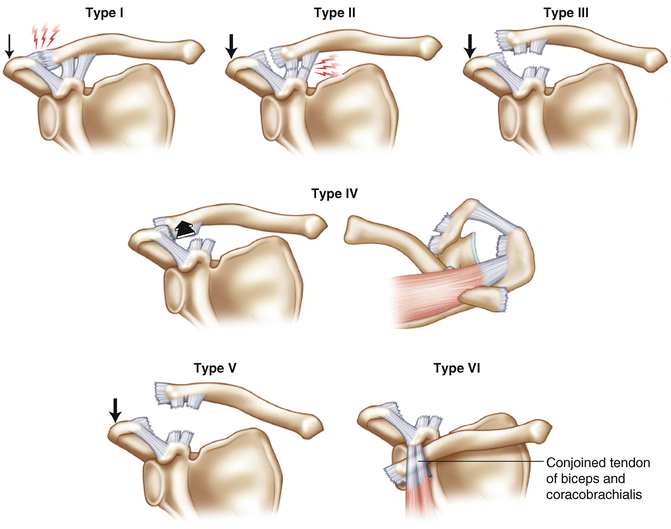
Fig. 7.19
Rockwood classification of AC joint injuries. Type I = sprain of the AC joint capsule; Type II = rupture of the AC joint capsule with possible sprain of the CC ligaments; Type III = rupture of the CC ligaments (i.e., dislocation) with superior displacement equal to approximately 100 % the width of the distal clavicle; Type IV = dislocation with posterior displacement that often punctures the trapezial fascia; Type V = dislocation with superior displacement of >100 % of the width of the distal clavicle; Type VI = subcoracoid dislocation.
Type I Injuries
Patients with type I injuries typically present with mild to moderate pain and swelling over the AC joint following a traumatic injury; however, there are no visible or palpable deformities of the AC joint on clinical examination. In these cases, shoulder motion does not consistently generate increased pain at the AC joint. Radiographically, although some soft-tissue swelling may be present, the distal clavicle appears aligned with the acromion without any significant increase in the measured CC distance when compared to the contralateral shoulder (Fig. 7.20). According to the original classification, type I injuries represent a sprain of the capsuloligamentous structures without disruption of any associated structural ligaments.
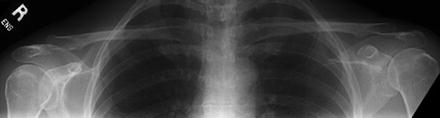
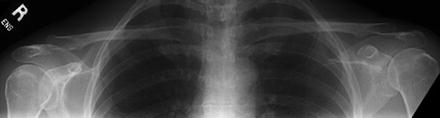
Fig. 7.20
Zanca radiograph demonstrating a right type I AC joint injury.
Type II Injuries
Type II injuries are characterized by moderate to severe pain over the AC joint which usually increases when shoulder motion is initiated. Palpation of the AC joint often reveals moderate swelling and slight superior migration of the distal clavicle relative to the acromion. Horizontal instability, which can be detected by manually grasping the clavicle and applying an anterior–posterior pressure, may be present in some type II injuries. AP or Zanca radiographs may show slight superior displacement of the distal clavicle; however, there is no significant difference in CC distances between the injured and non-injured shoulders (Fig. 7.21). In type II injuries, the AC capsuloligamentous structures are torn which allows the clavicle to migrate superiorly; however, the CC ligaments remain intact.
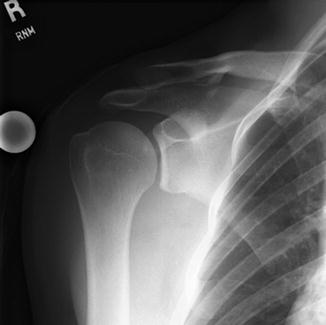
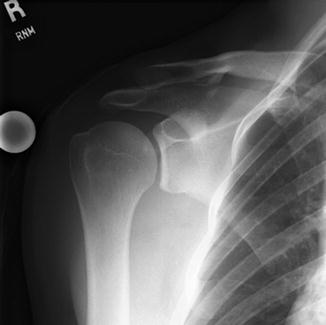
Fig. 7.21
AP radiograph of a right shoulder in a patient with an acute type II AC joint injury.
Type III Injuries
Patients with type III injuries usually present in moderate to severe pain with the arm in an adducted position and the weight of the arm supported either by a sling or the uninjured arm to help provide pain relief. The joint is tender to palpation and an obvious deformity is usually present which represents significant superior displacement of the distal clavicle. Manipulation of the clavicle would reveal both horizontal and vertical instability although significant guarding is usually present in the clinical setting. Radiographically, the clavicle will appear superiorly displaced relative to the acromion by approximately 100 % the width of the distal clavicle (Fig. 7.22). It should be recognized that the distal clavicle actually does not translate superiorly by a large amount—much of this superior displacement is related to the weight of the arm which pulls the acromion inferiorly relative to the clavicle. This injury pattern requires complete disruption of both the AC joint capsule and the CC ligaments while the deltotrapezial fascia remain intact. A shrug test has been described to differentiate type III and V injuries. In this test, reduction of the injured AC joint by having the patient shrug their shoulders indicates that the deltotrapezial fascia is intact. If shrugging does not reduce the joint, the deltotrapezial fascia has been ruptured which usually signifies a type V injury [17].
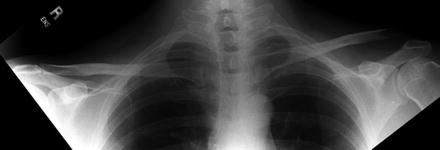
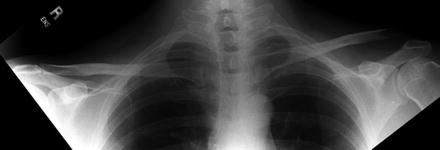
Fig. 7.22
Zanca radiograph demonstrating a left type III AC joint dislocation.
Type IV Injuries
Type IV injuries are characterized by complete posterior dislocation of the distal clavicle which typically pierces or punctures the fascia of the trapezius muscle. Patients with type IV injuries often present with severe swelling and pain localized to an area posterior to the medial acromion. In some cases, the distal clavicle may also produce skin tenting posteriorly. Evaluation of Zanca radiographs may reveal mild superior displacement whereas the axillary view will show significant displacement of the distal clavicle posteriorly, possibly making contact with the anterior aspect of the scapular spine (Fig. 7.23). Although infrequent, type IV AC joint injuries can occur in combination with an anterior dislocation of the medial clavicle at the SC joint, thus producing a “floating clavicle” (Fig. 7.24). Therefore, the clinician should also examine the SC joint for any signs of instability in cases where a type IV AC joint injury is suspected (details regarding examination of the SC joint are presented in Chap. 8).
