Tendons
Structure and Classification
Tendons and ligaments are similar structures in terms of their composition, organization, and mechanical properties. The distinction between them stems from their anatomical location; tendons form a link between muscle and bone while ligaments link bones to bones [1].
Tendons are dense fibrous tissues of musculoskeletal system, which play fundamental roles in stability and mobility. They vary according to their sites of distribution but all have an attachment site to the bone and with the aid of this property, they bare high loads with minimal deformation. Tendons are composed of three components: tendon itself, muscle–tendon junction, and bony insertion. They have a complex structure and are composed mostly of connective tissue. Originating from their functional properties, they are very tough to carry hundreds and even thousands of Newtons. Most of them usually cross the joints to attach their distal sites, which increases the affectivity of muscles on joint movements. Additionally, tendons carry weights similar to the bones and therefore their mechanical properties are variable. Tendons are a part of bone–muscle–tendon unit. Therefore, the localization of tendons should be known to understand its structure since not all muscles have tendons [2]. Tendons are found in some group of muscles, which play active roles in joint movements while the presence of some tendons increases the movement distance of the muscle.
A tendon is a compositionally complex tissue with a predominantly mechanical function: translating muscular contractions into joint movements by transmitting forces from muscle to bone. Achilles tendon, for instance, is a special tendon that centralizes the power of a couple of muscles, and carries their weights. On the other hand, some tendons including posterior tibial tendon act by distributing the weight over more than one bone due to their sites of attachment. Although we know that most tendons originate from the muscle and attach to the bone, some tendons may act like sites of origin for muscles or two muscles may be attached with a tendon [3, 4].
Histologically, tendons consist of dense regular connective tissue fascicles encased in dense irregular connective tissue sheaths. Tendons are composed mostly of parallel arrays of collagen fibers closely packed together [5]. Structurally, tendons are composed of collagen fibrils and collection of these fibrils constitute bundles, collection of the bundles form fascicles, and finally, a group of fascicles come together to form a tendon. Connective tissues surrounding the tendons permit friction. Most tendons have a mesotendon that attaches to the surrounding connective tissue and ensheathes it. This structure is also responsible for the vascularization of the tendon [6]. In addition, tendons contain water representing the 55–70 % of the total wet weight. The water content in tendon could play an important role of healthy or pathological index for different clinical presentations.
In some body sites, surrounding connective tissue forms a sheath and ensheathes the tendon. Multiple bundles are composed of fibroblasts and dense linear collagen fibrils. These form the structure of a tendon and confer a fibrous appearance to it. The low-density connective tissue surrounds tendon fascicles on its own. This structure is then called endotendon. When tendon fascicles are surrounded by endotendon, tendon bundles are enabled to make small sliding movements. Endotendon tissue continues as an epitendon covering tendon surface. At the site where tendon joins muscle, epitendon is continuous with epimysium of the muscle. Muscle–tendon unit should transmit muscle contraction to the tendon. Attachment of a muscle to tendon occurs by interdigitation of collagen fibers of the muscular fibrous tissue layer with collagen fibrils of the tendon. Electron microscopic examination reveals the structure of muscle cells and tendon, which appear as interdigitated fingers of both hands. Collagen fibrils do not enter into the muscle cells, but they firmly attach underneath the cellular basement membrane. Normal tendon sliding movement, transfer of muscle power for joint movement, and vascularization of tendons depend on peritendinous connective tissues. This is also called as peritendon. These structures form a delicate organized structure, which is composed of loose connective tissue [7].
Attachment of tendons to bone is complex; collagen fibrils integrate into fibrocartilage, mineralize, and then unite with the bone. “Sharpey’s fibers”, continuity of tendon fibrils that extend along the periosteum with outer lamellar structure of bone, are important for tendon entry, which is known as enthesis. Attachment of tendon to bone resembles fibrous or indirect attachment seen in metaphysis or diaphysis of long bones, or fibrocartilagenous or direct attachment seen in attachment to epiphysis or apophysis of bones. In fibrous attachments, collagen fibers of the tendon are continuously attached to the periosteum during the entire bone development, while in fibrocartilagenous attachments, transition from tendon to bone is more gradual. This gradual transition observed in fibrocartilagenous attachments includes four zones, namely; (1) tendon, (2) decalcified fibrocartilage, (3) calcified fibrocartilage, and (4) bone. With this organization, the load is evenly distributed at the site of attachment and coordination of collagen fibers is maintained with joint movements. In addition, compressive forces show variances inside a tendon depending on sites of attachment and this affects the structure of fibrocartilage. Therefore, a better protection against compressive forces is ensued [8].
Despite the fact that blood circulation is very important for tendon health, it is not as rich as the muscle and bone tissues. The vascularization of tendons is primarily from muscle–tendon unit, paratendon, and synovium at the site of attachment to tendon. Some tendons are supplied by paratendon surrounding it, as in the Achilles tendon, while others are supplied from a real synovial sheath surrounding them. Since there is a cartilagenous tissue at the site of attachment to bone and tendon, blood flow cannot directly pass through bone–tendon unit. Instead, they make indirect contacts through the vessels of periosteum. Diversely, tendons have a rich nervous network. They are usually innervated from the muscle with which they are interacted, or its local cutaneous nerves. Nerve endings are located at bone tendon units or underneath muscle tendon unit as Golgi organs, Pacinian corpuscles, or Ruffini corpuscles. Golgi organs are stimulated mechanically only with pressure or compression, and their functions differ according to the locations they are situated in. By this, they receive information from the power generated by the muscle itself. Pacinian corpuscles are fast-adapting mechanoreceptors, which contain capsular endings highly sensitive to deformations. By these mechanisms, they dynamically respond to deformation, but they are insensitive to continuous or constant changes. Ruffini endings are originated from a single axon with multiple thin capsular endings and they have slowly adapting mechanoreceptors. With these features, they are stimulated during deformation while they continuously receive information until they reach a constant level of stimulation. Free nerve endings are usually seen in myotendinous unit [9].
Tendons are classified according to their shape, localization, and anatomical structure. In this classification, tendons are divided into spherical and flat types while they do not have significant differences in terms of function and structure. In addition, spherical tendons have parallel collagen alignment, which helps them to give equal responses against tensile forces while flat longitudinal and oblique collagen alignments are found in flat tendons, which are more resistant against pressing and crossing forces [10].
Tendons can also be classified according to their localizations in multiple ways while the most reasonable classification would be according to their function as intra- and extraarticular. While tendons are mostly extraarticular, there are tendons located inside the joint, including the long head of biceps tendon, and popliteus tendon of the knee.
Anatomical localization of tendons can be classified as sheathed or unsheathed and synovium-covered or paratenon-covered. Actually, this anatomical classification should consider soft tissue protection and blood circulation of two tendon types.
Morphology, Histology, Microanatomy, and Cell Biology
The predominant cell type for tendons is fibroblasts. In addition to water, collagen, elastin, proteoglycan, and noncollagenous proteins unite to form macromolecular framework of dense fibrous tissues. These cardinal elements of tendon structure are not randomly aggregated at various ratios and types. Water is the main element of all structures, including tendons. The water content of tendon structure is 50–60 % and alterations in this ratio affect normal structure and function of tendon considerably. This fact becomes obvious in situations at which tendons are damaged in some ways [9, 10].
Collagen constitutes 70–80 % of the dry weight of tendon of which 95 % is collagen type 1 and 5 % is collagen type 3 [11, 12]. In addition, collagens type 5 and 6 are found in small amounts. Collagen molecule found in its structure is primarily found as triple helicals and one or more nonhelical structures integrating into the structure. Each individual chain characteristically contains glycine-x-y tripeptide sequence where x is usually a proline, and y is hydroxyproline [13]. With this, the primary structure of collagen is glycine in 33 %, proline in 15 %, and hydroxyproline in 15 %. As a result, these collagen chains form a superhelical structure with linear stick shape, which is approximately 1.5 nm in diameter and 300 nm in length with 1050 amino acids [14]. Type 1 collagen is produced by tenocytes and forms 90 % of the cellular component of the normal Achilles tendon. But, type 3 collagen may affect the tensile strength of the tendon generally produced under pathological conditions [15].
Proteoglycans are other fundamental units of the structure, which constitute 1–5 % of the dry weight of tendons. Proteoglycans are macromolecular proteins and contain negatively charged glycosaminoglycans as carbohydrate chains. With the help of this organization, tendons are hydrated and maintain their viscoelastic structure. Proteoglycans also contain more than 30 molecules forming the elements of extracellular matrix. Among these molecules, decorin and tenascin-C are well-known. Decorin is the most abundant proteoglycan found in tendon, which takes role in surface attachment of collagen fibrils and fibrillogenesis inside collagen itself as seen by in vitro settings. Tenascin-C is found abundantly in osteotendinous and myotendinous areas, which contributes to the matrix structure and affects the cells found in extracellular matrix, which are in contact [16–18].
The predominant cell type in a mature tendon is tenocyte while they are named as tenoblasts in newborns. The substantial difference between tenoblasts and tenocytes is predominance of aerobic cycle in tenoblasts while tenocytes prefer anaerobic respiration over time. These conditions are explained by the decrease in the metabolic load of mature tenocyte, as well as ischemia and necrosis risk against loading. In addition, there are some recently described cells with complex structures organized within a tendon. It is thought that there are cytoplasmic connections between these cells are and these networks are sensitive to loading. Therefore, tendon cells respond to loading and similar other stimuli with coordination [19].
Most of the tendons move inside of vascularized tendon sheaths, which are lined with mesenchymal cells resembling synovium. The factor ensuing the sliding of tendon is hyaluronic acid synthesized by mesenchymal cells. This sliding function can be disturbed by inflammation caused by fibrous adhesions developed due to immobility after trauma or surgery.
Functions and Biomechanics
The common function of tendons independent from their shape and size while transmitting the muscle contraction force to the bone. In other words, the primary function of tendons is transmitting the load of weight endured by the muscle to bone. Therefore, tendons are more powerful than muscles and they are subject to intense pressure and tensile forces due to their characteristics. Tendons have stronger structure than muscles, in terms of the resistance that they show against tensile forces, are equal to bone tissue but more flexible and more extensible than the bone [20]. Therefore, tendons execute joint movements and help muscles affect a point further from itself. In addition, they are resistant to tensile forces, but are also flexible, which helps them elongate a little bit as an elastic tissue. With the help of these features, the force generated by the muscle is transmitted to bones, and they exhibit perfect interactions with joint areas and the surroundings of bones. The resilience of tendon against tensile forces is similar to the bone, for example, a tendon of 1 cm thickness can endure a load of 600–1000 kg. Some tendons can store elastic energy in elongation and shortening cycles. By this way, it is obvious that they carry 400–1800 times more elastic tensility stress/unit. In addition, due to their viscoelastic structure, in high-energy injuries or sudden direct trauma, they decrease the severity of muscle injury. Some tendons act to strengthen the capsule of synovial joint and provide joint surface [21, 22].
Some tendons contain bony or cartilaginous sesamoid bones. These bones enable the compatibility of tendon to bone surface. The most vulnerable site of tendon is its end on muscle side. Enthesis, on the other hand, which is the site of attachment to bone is very strong and is susceptible to calcification. The fibrous tendon to bone enthesis is established through a structurally continuous gradient from uncalcified tendon to calcified bone [23]. Depending on where they are located, as in the case for Achilles tendon, they can take role in a single attachment site as a center of more than one muscle, or they may transmit the power of contraction of an individual muscle to more than one bone as in tibialis posterior tendon. Apart from all these, at joints including wrist and ankle, they enable the effect of muscle at a site distant from muscle attachment site, and passage through narrow sites of the body, or as in replacement of posterior tibial tendon around medial malleolus, it may change its way with the help of pulley and align its direction. Moreover, tendons act as dynamic stabilizers of joint in addition to their neurosensorial and proprioceptive functions. They function as the joint stabilizers of the force originating from the muscle and acting on the joint.
One of the most important biomechanical features of tendons is their nonlinear anisotropic behavior. The initial response of tendon to tensile forces is flattening of fibers. This feature is particularly due to the elastic properties of collagen and it constitutes the first section of force–deformation curve. Tendons demonstrate relatively higher compliance, particularly under conditions with lesser load. However, with increasing tensile strength, tendons increase in toughness and this toughness continues as long as a linear toughness is reached. At this step, elastic elongation and molecular separation occur due to separation of fibrils. After this level, energy is absorbed and tendons lose their tensile strength. In other words, the behavior of tendons might change depending on the increases in the rate of tensility. At higher levels of tensile forces, tendons get harder and as they gain tensility, they absorb more and more energy. On the other hand, to minimize the effect of injury, alterations occur at sites of tendon attachment to bones. With the help of this feature, they act at high toughness and power at sudden tensile forces. By this way, bone–tendon complex adapt and react to minimize trauma at high tensile forces [24, 25].
The position of tendon during endurance is particularly important during tendon ruptures. During oblique forces at eccentric positions, tendon ruptures are more common. In general, the final loading of tendon is more powerful than its own muscle or attachment site and thus, tendon avulsions are more common than tendon ruptures.
Since tendons are viscoelastic, their current and past characteristics are important. Therefore, their mechanic behaviors depend on the ratio of loading, limits of loading, loading histories, and environmental factors including heat and water content. In addition, tendons maintain biomechanical balance not only during normal daily activities and repeated displacements, but also during lower loads (i.e., static shape changes) or repeated lower loads (i.e., static position changes) [26]. This behavior pattern is important in clinics because deformation is reversible to some extent. However, joint laxity may ensue after pathological deformation-related injury, repair, or reconstruction.
In addition to their mechanical features, tendons take important roles in joint proprioception. Proprioception means that an extremity has a conscious perception in space. Central nervous system receives inputs from more than one centers and it describes these inputs as perception or sensation according to the movement of joint. Motor control of central nervous system centers gives response depending on visual, vestibular, cochlear, cutaneous, articular, or muscle-related information. At this point, tendons play active and important roles as well. Tendons function as trauma absorbers and maintain posture. Tendons are important to draw muscle from a small area, which helps changing the pulling direction of muscle and controlling the muscle from a distance [25].
Tendon Diseases and Injuries
Tendon diseases may involve any structure constituting a tendon and it may arise due to different reasons at different sites of a tendon (Table 28.1). Therefore, it is difficult to differentiate it. Moreover, the majority of tendon injuries occur due to excessive forces applied to vulnerable sites of muscle–tendon complex. These kinds of injuries show variations depending on anatomical localization, vascularity, skeletal maturity of patient, and the force being exposed to. Tendon does not rupture with the normal borders of physiological loads. However, tendon that has a deterioration of its structure, particularly as a result of aging and external injuries, can rupture without sudden and extreme loads [27, 28].
Table 28.1
Tendon injuries and diseases
Disease | Description | Example | Clinical findings |
---|---|---|---|
Ruptures | Disruption in the integrity of a tendon (partial or complete) | Shoulder tendon rupture—Achilles rupture | Pain (may be absent), loss of strength, feeling of emptiness on palpation |
Paratenonitis | The disease of paratenon layer | Achilles paratenonitis | Pain, tenderness, swelling, crepitation, warmth (early term) |
Tenosynovitis | The disease of synovial sheath itself | De Quervain disease | Pain, tenderness, swelling of the sheath, crepitation, warmth (early term) |
Stenosing tenosynovitis | The disease of tendon and synovial sheath | Trigger finger | Pain, crepitation, nodule on palpation, triggering in the finger |
Tendinosis | Degeneration of tendon structure (wear and tear) | Lateral epicondylitis, shoulder tendinosis | Pain, localized tenderness, nodule on palpation |
Entesopathy | Problem in the attachment site of tendon to the bone | Achilles tendinosis | Tenderness, swelling at the site of attachment to bone, pain |
Contracture | Hardening of tendon sheath and attachment of it to the surrounding tissues | Joint contracture | Restrictions in joint mobility |
Depending on their types of occurrence, tendon injuries can be classified as direct and indirect mechanisms. Direct mechanism means that the injury occurs as a result of a trauma without being linked to a prior disease. They usually occur at hand–elbow region and the healing process in such injuries might change depending on the presence of paratendon or sheath. However, with indirect mechanisms, tendons are damaged from bone or muscle–bone unit. There is usually a pathological degeneration process prior to such injuries. Histological changes include tendinosis and exhibit increases in collagen degeneration, fibrillar disorientation, hypercellularity, vascular alterations, and glycosaminoglycans in between fibrils. Together with that, Achilles tendon injuries, which are particularly common in our daily life, may present with different clinical pictures. For instance, a young sportsman who has no prior Achilles tendon problem may have a direct trauma, which can cause tendon injury. In other way, a middle-aged sportsman with no prior sports carrier may experience Achilles tendon injury after physical activity, which usually demonstrates an “angiofibroblastic hyperplasia” assessed by pathological studies, which is a degenerative process and underlies the preexisting tendinosis. Similarly, supraspinatous tendon injuries, which are common in daily life and occur after degenerative processes, have histological alterations, including decreased cellularity, thinning of tendon fibrils and disorganization, presence of granulation tissue, glycosaminoglycan infiltration, fibrocartilaginous alterations, calcification, and partial tears. In addition, tendon degeneration originates mostly from multifactorial reasons and among them are mechanical compression, pressing, and tensile forces. In addition to them, ischemia, free oxygen radicals, hypoxia, pathological changes, tenocyte apoptosis, and fluoroquinolone use are among other potential mechanisms [14, 29–31]. In addition, in repaired complete ruptures, a hard and heterogeneous pattern of tendon structure may be a natural consequence of tendon healing [32].
Radiological examination of tendons is based on magnetic resonance (MR) imaging. MR imaging makes it possible to evaluate the presence of tendinous “degeneration”, or of a tear partial or complete, the extent of tendon retraction in full thickness tears, and the presence of associated lesions.
It is obvious that excessive or oblique load-related tensile forces might cause disruptions in tendon structure. However, this damage is at microscopic level and it is usually repaired by tendon cells. With prolonged or repeated tensile forces or compressions, repairing capacity might not reach damage rate, which causes accumulation of damage and partial or complete ruptures with pain. In addition to that, gene structures, structural reasons including nutrition and environment, decrease in blood flow, various rheumatic diseases, including gout and rheumatoid arthritis, directly affect tendon and decrease inflammation or repair abilities, causing accumulation of damage [33, 34]. Additionally, a high Body Mass Index (BMI) >25 and constitutional factors (such as the asymmetry of the lower limbs) may exert an excessive or abnormal load on tendons. Also, hypercholesterolemia appears to be associated with an increased risk of tendon rupture. Furthermore, clinical studies have pointed an association between diabetes and calcific tendinitis.
The fundamental manifestation of tendon diseases is pain and loss of function. Clinical examination may also show swelling and thickening. Generally, there is a local tenderness and pain increases with passive tension and active resistance. With predominant loss of strength, rupture of tendon should be thought. Severe paratenonitis demonstrates crepitation and triggering is also seen. Palpable tendon nodule is consistent with tendinosis. Degenerative changes are named as tendinosis and inflammatory changes are termed tendonitis, which separates these two terms. The difference between paratenonitis and tendinosis is difficult when cardinal signs of inflammation including swelling, redness, and increased heat are absent.
In conclusion, tendon injuries may occur as a result of direct or indirect mechanisms or rapid contraction due to overloading of muscle or sudden change in position of an extremity with rapid muscle contraction. We can classify all types of tendon injuries depending on the duration of lesion development. In this method, acute lesions are the ones with less than 2 weeks of duration, subacute lesions are between 2 and 4 weeks, and chronic lesions are more than 4 weeks [35].
Tendon Repair and Healing Periods
Healing process of tendon is slow and in case of injury, surgical repair methods cannot obtain mechanical features of tendon as in uninjured tendon [36]. Two separate models are proposed to explain tendon healing mechanisms. Extrinsic healing model describes the formation of granulation tissue with migration of cells and vessels from surrounding tissues while intrinsic healing model encompasses synovial fluid-supported healing with the healing capacity of cut edges of tendon. Both of these mechanisms are clinically seen in injured tendons, but extrinsic healing predominates in early period while intrinsic mechanisms take place in later phases.
Extrinsic mechanism encompasses the invasion of inflammatory healing tissue and fibroblasts into the injured area from outside the tendon. Extrinsic mechanism is held responsible for high cellularity, high water content in injury line and disorganized collagen matrix, and adhesion formation occurring initially. Adhesion formation is dependent on the severity of trauma, surgical trauma, lack of blood supply to the tendon, immobility of tendon, and loss of tendon sheath. In other words, prolongation of extrinsic mechanism during healing period causes adhesions of tendons, thus, suppressing extrinsic mechanism in tendon repair is important. On the contrary, intrinsic mechanism includes synovial fluid diffusion and intrinsic vascularization of tendon. With this mechanism, fibroplasia and new collagen formation can take place with no adhesions on superficial layer. In general, the mechanism is responsible for reorganization of collagen fibrils and their maintenance. Several growth factors operate in organization of cellular response and its activation during entire tendon repair process. These factors or cytokines bind to specific receptors found on cell surface, activating particular signalization events inside a cell. This starts with a cascade, which leads to transcription of specific regulatory genes. Elaboration of these factors occurs during entire remodeling process by cells localized at the border of injury and also with mechanical pressures on injured tendon. As a result, one of these two healing pathways predominates. Under some circumstances in which synovial sheath integrity is maintained and tendon mobility is ensued, intrinsic healing predominates and tendon adhesion occurs at a bottom level. If extrinsic healing predominates, adhesions in tendon and surrounding tissues would be more prominent [37, 38]. In addition, tensile testing and clinical studies have sought to compare different suturing techniques, often focusing on strength, gapping resistance, glide and resultant mobility [39]. A strong tendon repair that reduces dehiscence and allows early movement may be all that is needed to produce good results [40].
The healing of tendon takes 6–8 weeks, which can be evaluated in three stages: inflammatory, proliferative, and remodeling. Inflammatory stage encompasses the time between days 1 and 5 after tendon injury. This stage is the time when tendon strength is minimum and is characterized by cellular migration and phagocyte activation. Hematoma formation occurs in tendon healing line due to the injuries to blood vessels. The clot that is formed causes the migration of inflammatory cells from the surrounding tissues, and elaboration of several proinflammatory and vasodilator molecules in addition to chemotactic factors. Fibronectin, which is also a chemotactic molecule, increases as well as cell surface integrins. Basic Fibroblast Growth Factor (bFGF) also operates in tendon healing and it is a growth factor that takes place in early mitogenic activities. It acts as a potent angiogenic factor at the same time. Erythrocytes, thrombocytes, neutrophils, monocytes, and macrophages migrate to the wound areas. Clot, cellular debris, and foreign molecules are phagocytozed. During the first week, the area of injury is filled with extrinsic peritendinous tissue, as well as epitenon and endotenon-originated cells. As the cells proliferate, phagocytosis increases and new collagen formation occurs. Fibroblasts populate in this area to initiate extracellular matrix synthesis. Angiogenic factors are released during this period and they initiate vascular network formation. DNA and extracellular matrix increase, both of which take place in maintenance and partial stability of wound area. It is possible that a combination of wrapping periosteum and injecting bone marrow to the tendon graft would have a synergistic effect (early and strong). To prove this hypothesis, future studies, which would combine both methods, are needed [41, 42].
During proliferative phase, clot organization occurs with cellular proliferation and matrix synthesis. With the organization of clot, fibroblasts come from parenchymal tissue and wounded tendon sheath, proliferate in the area of injury, and synthesize all components of extracellular matrix including collagen, and proteoglycans. Between days 7 and 10, vascularization increases rapidly in epitenon and endotenon. On day 7, collagen fibrils are visible. During initial phases, these components are randomly arranged in extracellular matrix (ECM) and type 3 collagen is primarily produced in this phase. A dense blood vessel network is formed and the wound seems to be scarred. At the end of this stage, repair tissue is highly cellular and the water content, as well as ECM component is increased. During this period, granulation tissue is seen at the site of repair. Matrix is unorganized as well. Tensile strength and proper organization of the tendon are not well constructed. With the synthesis of collagen, tendon strength increases gradually.
Remodeling is the terminal stage of tendon healing. After these stages, repair tissue is formed during the second week and this unites tendon endings together. During remodeling phase, cellularity, matrix synthesis, and type 3 collagen decrease, while type 1 collagen synthesis increases. Collagen synthesis occurs not only at the site of injury, but in the tendon as a whole. Between days 14 and 28, collagen synthesis is maximum. Type 1 collagen fibrils align as long as the entire axis of tendon longitudinally, and are responsible for mechanical force and tissue regeneration. As long as the remodeling phase continues, vascularity and cellularity decreases while collagen fibrils and cross bindings increase. At 20th week, minimal histological changes are seen in tissue during early tendon healing, while collagen content and tendon collagen fibril organization and diameter change substantially. While some tendon characteristics return back to their normal structure, this process usually takes months, and even years [43].
Taking into consideration the biomechanical properties of tendon in the healing process is critical for the management of tendon repair and healing. The scar tissue in the first stages of the repair process is in insufficient state for stretching. For this reason, immobilization of the joints related to tendon in the process of repair and recovery is necessary for optimal healing. It has been mentioned that, in tendon healing process, the mechanical stimuli play an important role in the formation of scar tissue and collagen fibrils. To achieve this depends on the strength of the repaired or healing tendon tissue. Therefore, to obtain the strength of healing tissue was considered as main part in the development of tendon repair methods. Tendon-to-bone healing is vital to the ultimate success of the various surgical procedures performed to repair injured tendons. Achieving tendon-to-bone healing that is functionally and biologically similar to native anatomy can be challenging because of the limited regeneration capacity of the tendon–bone interface [44–48]. Beside the ruptures, the exposure of tendon to the degenerative process sometimes may result in malfunction, which can need medical treatment [49]. During this period, using of heparin is controversial [50].
< div class='tao-gold-member'>
Only gold members can continue reading. Log In or Register a > to continue
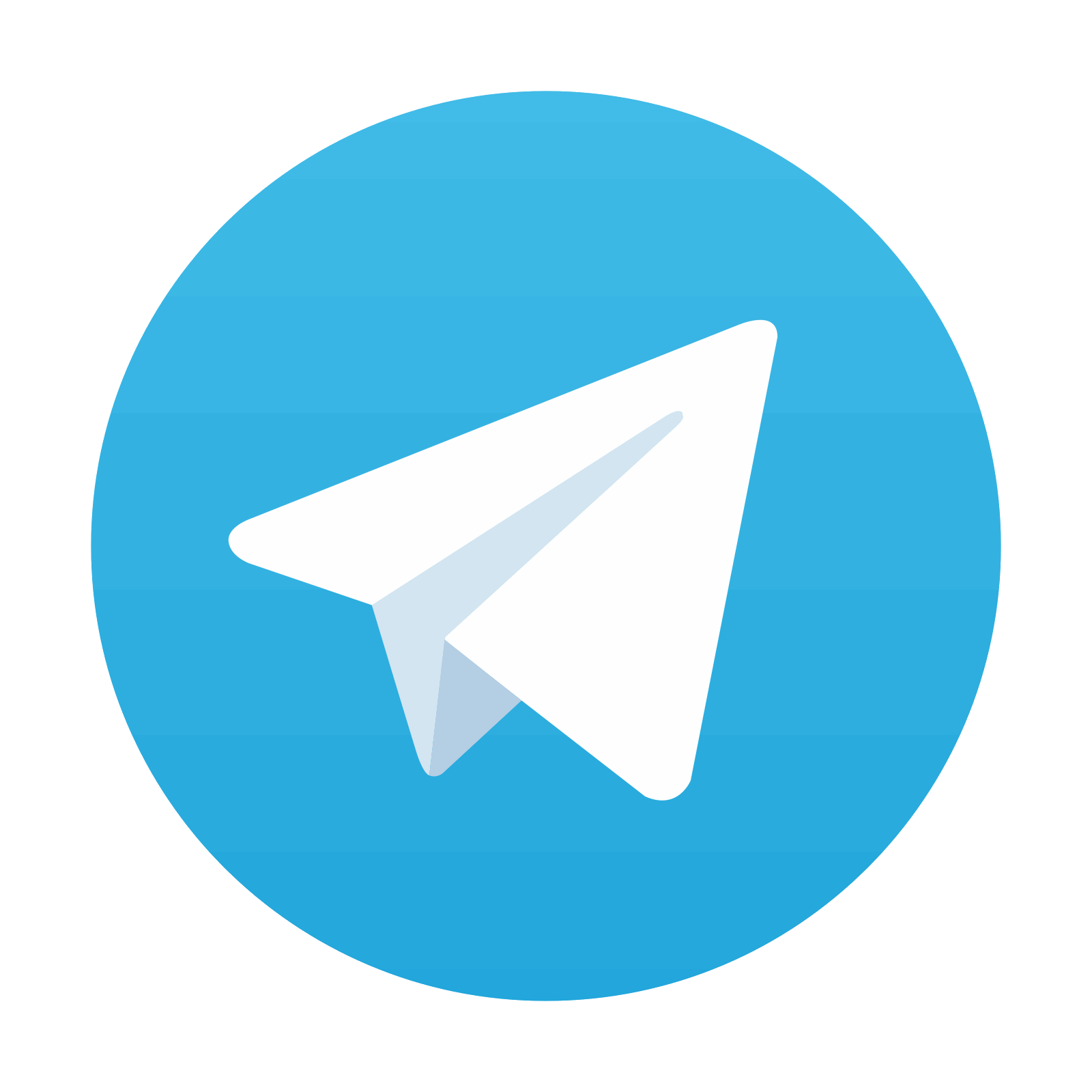
Stay updated, free articles. Join our Telegram channel

Full access? Get Clinical Tree
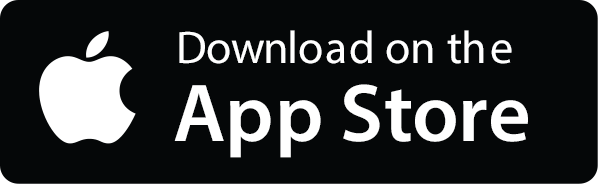
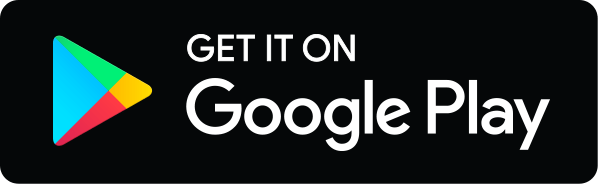