(1)
University of Pittsburgh Medical Center, 3200 South Water, St. Pittsburgh, 15203, PA, USA
4.1 Introduction
The healing process following tendon injury is governed by a plethora of biomechanical factors that has been well studied in the literature from the basic science cellular model to interventions in the clinical setting. Overall, a fully healed tendon is characterized by reduced final mechanical stability (Sharma and Maffulli 2005). In order to optimize the treatment of tendon injury, the treating physician should have a thorough understanding of the principles of tendon healing. The following chapter will discuss tendon pathology, homeostasis, principles of healing, as well as biologic and mechanical factors affecting the healing response.
4.2 Tendon Homeostasis
The maintenance and viability of tendons are largely dependent on the structural molecules that constitute the extracellular matrix (ECM) (Butler et al. 2004). The tenocytes are the primary regulator of the ECM and tendon homeostasis (Galloway et al. 2013). These fibroblast-like cells are interconnected with one another and with adjacent collagen fibers, enabling recognition of mechanical changes in the ECM (Wang 2006). The tenocytes are able to respond to load and mechanical changes by modulating the degradation and formation of ECM (Wang 2006). The preservation of ECM homeostasis is critical in the tendon healing process and its capacity to respond to injury.
The ECM is composed predominantly of type I collagen, which is the primary constituent of the native tendon (Hogan et al. 2011). The longitudinal and parallel arrangement of type I collagen enables the ECM to respond to tensile loads (Karousou et al. 2008). Proteoglycans also play a role in the ECM’s capacity to resist tensile and compressive forces (Karousou et al. 2008). Indeed, the collagen network of the ECM is mechanosensitive and is stabilized by mechanical strain (Bhole et al. 2009). Bhole et al. showed, using dynamic differential imaging, that non-strained collagen fibril was resorbed faster than collagen fibrils subjected to strain (Bhole et al. 2009). Normal physiologic loads on the tendon unit are necessary to maintain the ECM homeostasis and structural integrity (Nabeshima et al. 1996; Flynn et al. 2010).
4.2.1 Mechanism of Tendon Injury
The pathophysiology of tendon injury encompasses a wide spectrum of disorders ranging from chronic tendinopathy to the acute complete tendon tear. One school of thought believes that acute tendon injuries are indicative of a chronic underlying tendon disorder (Maganaris et al. 2004). Others believe that acute injury results from one tensile overload, and chronic injury results from repetitive abnormal load over an extended period of time (Galloway et al. 2013; Maganaris et al. 2004; Lin et al. 2004). The viscoelastic properties of tendon allow its capacity to withstand compressive, tensile, and shear loads that are generated from muscle and transmitted to the rigid bone (Bunker et al. 2014). Noyes et al. described three primary failure modes of tendon injuries: (1) failure through the tendon substance, (2) avulsion fracture through the cancellous bone beneath the tendon attachment, and (3) failure at the enthesis (tendon bone interface) (Noyes et al. 1974). While the location of the tear has been well described, the type of abnormal load on the tendon unit plays an integral role in the pathophysiology of tendon injury.
4.2.1.1 Stages of Tendon Healing
The biologic cascade that modulates tendon healing is divided in three distinct stages, each governed by different cell types (Sharma and Maffulli 2006) (Fig. 4.1). The inflammatory stage is initiated by the traumatic event surrounding tendon injury, precipitating the hematoma and the agglomeration of platelets, releasing a slew of chemotaxic molecules, cytokines, and growth factors. The phagocytic cells, monocytes, neutrophils, and microphages, migrate to the site of injury via dilated vessels and begin the process of breaking down the blood clot and extracellular matrix. The process of angiogenesis is introduced by macrophages where a new network of vasculature matures in the healing tissue (Gelberman et al. 1992). The ECM is notably stabilized by the increasing amount of collagen type III, which are not yet aligned in parallel. The inflammatory stage lasts between 3 to 7 days following tendon injury. The amount of collagen will steadily increase during the first 5 days where the tendon callus will reach its largest size (Oliva et al. 2011).


Fig. 4.1
Stages of tendon healing: (a) Inflammatory phase. (b) Proliferative phase. (c) Remodeling phase (Strickland 2000)
The proliferative stage follows with an increasing amount of intrinsic fibroblast in the ECM. These cells emerge from the endotenon and epitenon and play a primordial role in resorbing and producing new collagen (Muller et al. 2015). At this stage, the immature healing ECM is still stabilized by a soaring amount of type III collagen, and this phase lasts for about 6 weeks. During the remodeling stage, the biomechanical strength of the tendon is at its greatest. The collagen is reorganized in a longitudinal, parallel fashion, while the collagen type III is replaced by collagen type I. The ECM will continue to mature for the next year with a rising amount of longitudinal and cross-linked collagen, while the callus volume of the tendon will decrease and the tendon biomechanical strength will continue to improve (Hogan et al. 2011). The healed tendon is biomechanically weaker than the uninjured tendon, with less cross-linking and smaller diameter collagen, and is more susceptible to reinjury (Hyman and Rodeo 2000). Dyment et al. corroborated these findings in a histologic and biomechanical study in the mice model, where they demonstrated that the healed tendon regains 63% of its original strength after an 8-week time period (Dyment et al. 2012).
4.2.1.2 Growth Factors
Growth factors are molecules involved in modulating the tendon healing response, more particularly during the inflammation stages following injury (Molloy et al. 2003; Sciore et al. 1998). The molecules involved include fibroblast growth factor (FGF), vascular endothelial growth factor (VEGF), platelet-derived growth factor (PDGF-?), transforming growth factor-beta (TGF-?), and insulin-like growth factor-1 (IGF-1) (Wurgler-Hauri et al. 2007; Holladay et al. 2016). While these growth factors have been studied extensively in vitro and in vivo, more recently, the attention of the scientific community has gravitated toward platelet-rich plasma (PRP) to augment tendon healing (Filardo et al. 2016). PRP has been studied in patellar tendon, rotator cuff, lateral elbow tendon, and Achilles tendon (Behera et al. 2015; Brkljac et al. 2015; Crescibene et al. 2015; De Carli et al. 2016; Carr et al. 2015). While PRP is widely used in the clinical setting, its efficacy is still controversial in the literature due to the heterogeneity of the study protocols (Filardo et al. 2016). Furthermore, new studies have focused on mesenchymal stem cells and their potential ability to differentiate into tendon cells and promote tendon regeneration (Chen et al. 2009).
4.2.1.3 Tendon Healing of Intrasynovial and Extrasynovial Tendon
As discussed earlier, the healing process following tendon injury is dictated by a multifaceted cellular healing response and is also influenced by the anatomical location of the injured tendon. The intrasynovial tendons, such has flexor tendons of the hand, and extrasynovial tendons, such as Achilles tendon, have different physiological mechanism of healing. The sheathed tendon heals with growth factors secreted from the epitenon and endotenon which passively diffuse through the synovial fluid to the site of injury (Strickland 2000). On the other hand, extrasynovial tendons, lacking the sheaths and synovial fluid, heal via blood flow and growth factors secreted by cells from the paratenon (Molloy et al. 2003; Galvez et al. 2014). The process of tendon healing is further characterized in intrinsic and extrinsic healing. During extrinsic healing, fibroblasts from the paratenon migrate to the site of injury and promote adhesion that potentially inhibit tendon excursion (Sammer and Chung 2014). On the other hand, intrinsic healing is characterized by fibroblasts migrating from the endotenon and epitenon in the synovial sheet, preventing the formation of adhesion with the surrounding tissue, facilitating early mobilization and strength of healing (Sammer and Chung 2014). Gelberman et al., in a study of flexor tendons in a dog model, showed that tendon that is immobilized after injury leads to collagen resorption while promoting adhesion with the surrounding sheath (Gelberman et al. 1983). In contrast, the mobilized tendon had an increase in collagen number and longitudinal orientation while demonstrating a decrease in adhesion with the peripheral soft tissue (Gelberman et al. 1983).
4.3 Rehabilitation
The cellular response during the tendon healing process is greatly influenced by the rehabilitation protocol initiated after injury. While the healed tendon never reaches the original strength of the native tendon (Dyment et al. 2012), there is a need to optimize the rehabilitation factors influencing tendon healing. Schizas et al., in an animal study on Achilles tendons, demonstrated that the immobilized healed tendon had decreased strength, length, collagen diameter and organized orientation (Schizas et al. 2010). Another study exhibited similar finding showing that immobilization impairs tendon healing (Bring et al. 2009). Bring et al., in a histologic study of rat Achilles tendons, demonstrated that immobilized tendon had a decreased amount of angiogenesis, fibroblasts, and production of collagen (Bring et al. 2009). Furthermore, early mobilization has been shown to enhance healed tendon strength and decreased adhesion formation in intrasynovial tendons (Strickland 2000). The translation of basic science to the clinical setting has yielded precious information regarding post-injury rehabilitation protocols. The application of controlled mechanical load should be an integral part of tendon healing to support ECM regeneration and maintenance (Flynn et al. 2010). Valkering et al., in a randomized controlled trial of Achilles tendon rupture, showed that functional weight-bearing enhances early healing response (Valkering et al. 2016). Functional weight-bearing also upregulated the release of metabolites associated with improved healing. The rehabilitation program should emphasize physiologic loading applied to the healing tendon to optimize ultimate tendon function and repair (Galloway et al. 2013).
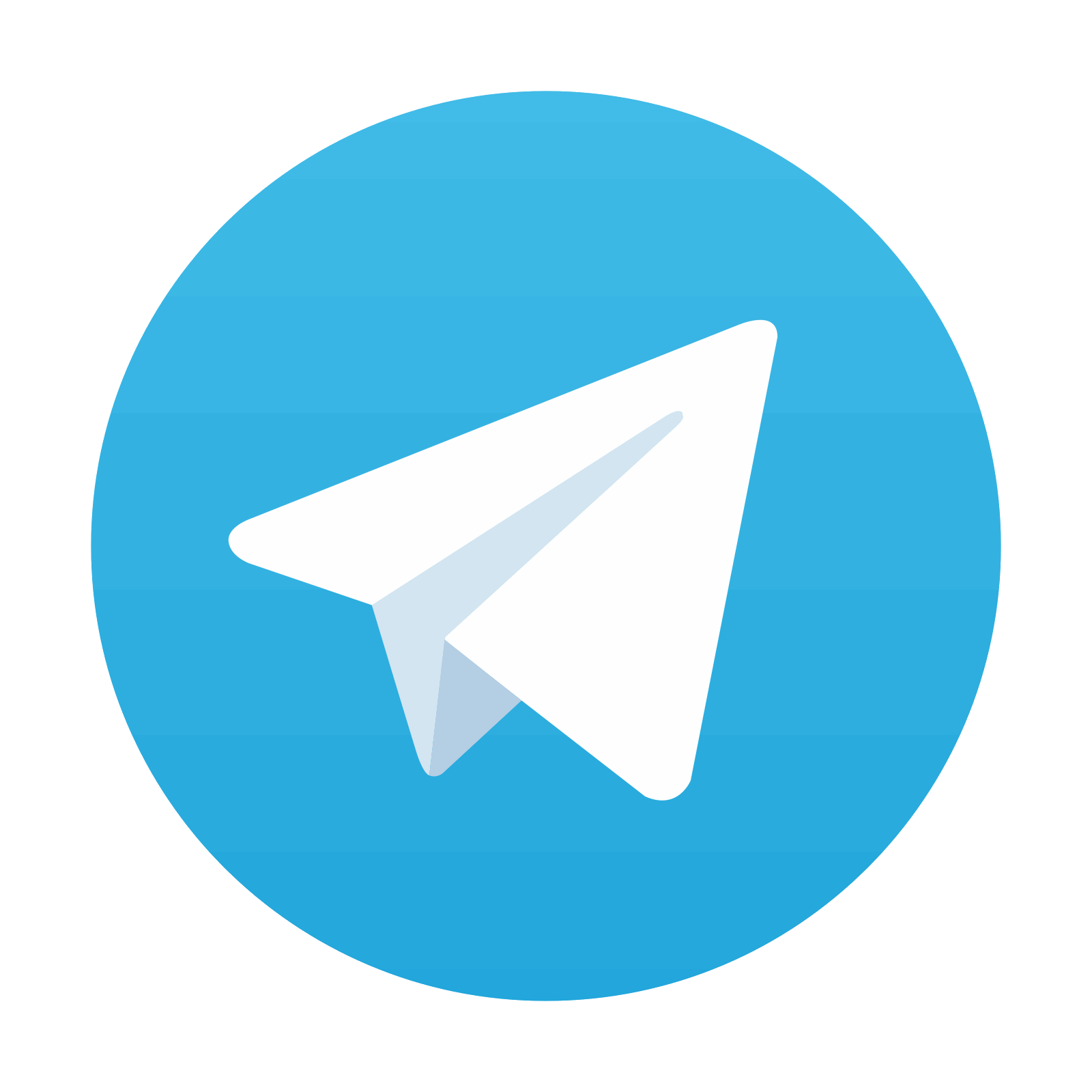
Stay updated, free articles. Join our Telegram channel

Full access? Get Clinical Tree
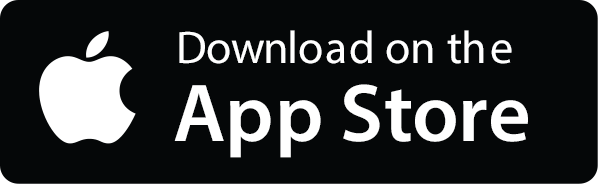
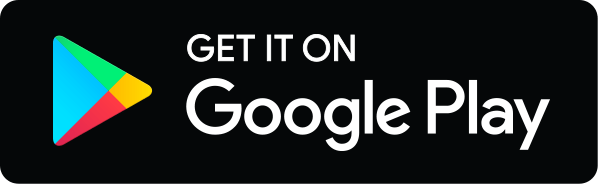