Talar Fractures
John M. Schuberth
Shannon M. Rush
Meagan M. Jennings
Although talar fractures are not particularly common, disruption of the integrity of this bone can be debilitating because of the unique anatomic features and functional position of the talus. As a result, talar fractures have been the subject of great discussion. Although the treatment has changed radically since the first reports, the outcome of these fractures can still be poor. Reconstruction of comminuted fractures with varying degrees of joint dislocation can present significant surgical obstacles for even the experienced surgeon. The exact algorithm for treatment of various injury patterns is still an area of debate. Accurate prediction of outcomes based on mechanism, injury complexity, and reduction is still imprecise. Often, patients require complex reconstructive procedures after initial treatment. Some patients with talar fractures may ultimately undergo talectomy. Although this type of procedure is usually reserved for surgical salvage, its continued use demonstrates the challenging aspects of these intriguing fractures.
ANATOMIC CONSIDERATIONS
Functionally, the talus is responsible for torque conversion during locomotion. Ankle joint plantarflexion and dorsiflexion, in combination with subtalar joint pronation and supination, are mediated by the talus, as is the intricate relationship of the articulation with the navicular. Because of its multiarticular functions, the talus is covered mostly by hyaline cartilage. Therefore, an injury to any focal anatomic area of the talus is likely to have an intra-articular component.
BLOOD SUPPLY TO THE TALUS
The blood supply to the talus is an intricate cascade of vessels that are vulnerable to injury. The precarious nature of the blood supply results from the limited nonarticular surface area for penetration of the vessels and the disproportionate degree of cartilaginous surfaces. In spite of the multiple anastomoses of the arterial channels, each of the major nutrient arteries supplies a focal anatomic area of the talus (1). Although all these vessels share an important role in the nutrition of the talus, those arteries that supply the majority of the body of the talus are more important because of the disastrous consequences that may ensue should they become disrupted. In addition, as the severity of the injury increases, so does the vascular disruption.
Some disparities have been described in the precise arrangement of the arterial supply of the talus. These differences involve the anatomic arrangements of the arterial supply as well as the consequences of vascular disruption (1,2,3,4 and 5). Many of the experimental studies undertaken to evaluate the blood supply have differed in technique and, therefore, probably in their accuracy as well, but in the later studies, a more consensusbased arrangement has been determined. However, a wide variability clearly exists in the arterial supply to the talus including the point of origin of each branch, the number of branches, and the region each branch supplies. This may explain the conflicting reports of the incidence of avascular necrosis (AVN) of the talus after injury. The most consistently accepted arrangement of the arterial supply of the talus is a hybrid one (3,4) (Fig. 108.1).
The artery of the tarsal canal has a variable origin from the posterior tibial artery and runs on the undersurface of the talus. It provides a branch that runs through the deltoid ligament, which, in turn, penetrates the talar body through the nutrient foramina on the medial surface. The artery of the tarsal canal has multiple anastomoses with the artery of tarsal sinus, which arises from the perforating peroneal artery near the sinus tarsi. It lies on the floor of the sinus tarsi, on either side of the interosseous talocalcaneal ligament, and enters the talus through the tarsal canal and the area just lateral. Together, the artery of the tarsal canal and the artery of the tarsal sinus supply most of the substance of the body of the talus. The artery of the tarsal sinus may also receive contributions from the dorsalis pedis vessel (Fig. 108.1A). Although not considered a major component, a branch of the posterior tibial artery arises just proximal to the tarsal tunnel and perfuses the small posterior aspect of the talar body (3,4 and 5) (Fig. 108.1B and C). The blood supply to the head and neck of the talus is provided by a branch of the dorsalis pedis artery, which originates distal to the ankle joint capsule and perforates the talar neck through its dorsal surface through its nutrient foramina (3,4 and 5).
With sequential failure of the various ligamentous structures during fracture dislocation, a sequential loss of the arterial supply to the talar body develops. Dislocation of the subtalar and ankle joints interrupts the vessels arising from the posterior tibial and the perforating peroneal arteries. Even after complete dislocation of the talar body from the ankle mortise and the subtalar joint after a fracture of the talar neck, the blood supply of the head and neck is usually not in peril. This is because the fracture line at the talar neck is almost always proximal to the origin of the blood supply from the dorsalis pedis. In the event the talar head and neck were to dissociate from the navicular facet, then this area would also be at risk of an avascular crisis.
CLASSIFICATION OF TALAR FRACTURES
For the purposes of this chapter, fractures of the talus are discussed on a regional basis. Each specific anatomic area is subject to a unique and specific array of fracture patterns, classification schemes, and, most important, prognosis.
FRACTURES OF THE HEAD OF THE TALUS
Fractures of the head of the talus are relatively uncommon (6,7) because this area has virtually no soft tissue attachments, a feature that essentially precludes the possibility of avulsion
fractures. The capsule of the talonavicular joint attaches to the talus well proximal to the articular surface. Any pure bending stress applied at the joint level would be unlikely to generate sufficient force to avulse the entire head of the talus. Yet, a combination of tensile and shearing forces may result in an injury to the talar head (Fig. 108.2). Fractures of the talar head occur when a fully plantarflexed foot is violently dorsiflexed (8). The most likely sequence is that the talar head is secured in the navicular concavity by the axial load, and then a secondary shearing force is imparted to the forefoot (9). Similarly, the same mechanism could occur with a medial- or lateralward moment of force as the head of the talus is secured by the navicular. Most of these fractures then occur at the periphery of the talar head, but rarely on the lateral hemisphere. Fractures of the talar head may also occur with subtalar dislocations with similar mechanisms. In addition, pure axial load of the talonavicular joint would most likely result in a sagittal plane split of the navicular.
fractures. The capsule of the talonavicular joint attaches to the talus well proximal to the articular surface. Any pure bending stress applied at the joint level would be unlikely to generate sufficient force to avulse the entire head of the talus. Yet, a combination of tensile and shearing forces may result in an injury to the talar head (Fig. 108.2). Fractures of the talar head occur when a fully plantarflexed foot is violently dorsiflexed (8). The most likely sequence is that the talar head is secured in the navicular concavity by the axial load, and then a secondary shearing force is imparted to the forefoot (9). Similarly, the same mechanism could occur with a medial- or lateralward moment of force as the head of the talus is secured by the navicular. Most of these fractures then occur at the periphery of the talar head, but rarely on the lateral hemisphere. Fractures of the talar head may also occur with subtalar dislocations with similar mechanisms. In addition, pure axial load of the talonavicular joint would most likely result in a sagittal plane split of the navicular.
The diagnosis of this injury pattern is made primarily on the basis of radiographic imaging and the physical evidence of swelling, discoloration, and pain in the area of the talonavicular joint. Oblique views of the foot may be particularly helpful in delineating the extent of the fracture. Rarely, computed tomography (CT) scanning is indicated to detail the injury further.
Treatment depends on the degree of displacement, the amount of articular surface involvement, and the amount of comminution (see Fig. 108.2). Generally, large displaced fragments from the articular surface should be anatomically reduced and fixated. Smaller fragments should be removed if they cannot be relocated and secured. Compression-type injuries are difficult to treat surgically and probably should be treated conservatively if the fragments are depressed below the articular surface or the round shape of the talar head is preserved. If the shape of the talar head has been distorted, then some attempt at open or closed reduction may be indicated.
The surgical approach is usually along the interval between the tibialis anterior and tibialis posterior tendons and extending over the navicular. The talonavicular joint can be forcibly abducted to gain visualization of the fracture if it is on the medial hemisphere. If the injury occurs laterally, the surgical approach is created lateral to the common long extensor tendons. Small-caliber screws or Kirschner wires (K-wires) can be utilized to secure the fragments. If screws are utilized, they should be placed below the articular cartilage.
Complications are uncommon when anatomic reduction is obtained or when the areas of impaction to the articular surface are small. Almost all patients experience some stiffness of the hindfoot complex when operative intervention is performed
or articular incongruities exist (10). Late arthrosis may occur if chondral damage has been significant. However, this condition is often diagnosed late if no obvious osseous damage has occurred at the time of injury.
or articular incongruities exist (10). Late arthrosis may occur if chondral damage has been significant. However, this condition is often diagnosed late if no obvious osseous damage has occurred at the time of injury.
FRACTURES OF THE LATERAL PROCESS
The lateral process of the talus helps to ground stabilizing structures of both the ankle and subtalar joints including the lateral talocalcaneal, anterior talofibular, and posterior talofibular ligaments (11). Although fractures of the lateral process of the talus are relatively uncommon (accounting for about 20% of all fractures of the talus) (12,13 and 14), the incidence appears to be increasing because of the popularity of snowboarding (15). This finding may be attributed to the use of flexible boots that allow more freedom of movement, yet provide less protection for the often extreme ranges of subtalar motion necessitated by this sport (16,17). Hybrid boots have been shown to reduce the incidence of these injuries (15). Often, recognition of the injury may be latent because of the subtle radiographic findings. Since the lateral process of the talus is a component of both the ankle and the posterior facet of the subtalar joint, the consequences of this biarticular injury may be significant.
The mechanism of injury has most commonly been described as a combination of inversion and dorsiflexion (14,18,19), during which a compressive force is transmitted to the lateral process. The foot must be locked into an inverted position for this mechanism to propagate; otherwise, the subtalar joint would be more mobile, and calcaneal eversion would most likely ensue. When the subtalar joint is forcibly pronated or the foot is dorsiflexed, the lateral process may collide with the floor of the sinus tarsi and may produce a compression-type injury pattern, but this is not as common.
Two fracture patterns are typically observed. The more frequent pattern occurs with a mechanism consisting of greater axial load that results in a fracture that is comminuted, but with little displacement (Fig. 108.3). In those patients with more of a shearing mechanism, the fracture fragment is usually larger and not comminuted. These fractures usually involve a larger area of the subtalar joint facet and can involve the lateral talarfibular articulation, depending on the morphology of the latter joint.
Fractures of the lateral process are frequently missed during the initial presentation to the emergency room (14,19,20). Most commonly, the injury is mistaken for a lateral ankle ligament injury because of the similar supinatory mechanism and clinical and radiographic findings (12,13,14,15 and 16). A distinguishing clinical feature of lateral process fractures is the absence of an anterior drawer sign, although obtaining a reliable measure of this test may be difficult in patients with acute symptoms. The diagnosis is more reliable when one has a high index of suspicion (21). The fracture is most commonly evident on anteroposterior or mortise radiographs of the ankle, depending on the exact positioning. Films made in a non-weight-bearing mode allow for better visualization. The fracture is more difficult to detect on the lateral radiograph, but some subtle findings aid in the diagnosis such as blunting or the loss of continuity of the inferior margin of the lateral process of the talus. In some instances, plain tomography or CT scanning is necessary to appreciate the entire articular involvement of the fracture (15,22,23 and 24) (Fig. 108.4).
Patients with small, comminuted fragments may be placed in a short leg walking cast for 4 to 6 weeks. Early range of motion may be instituted as soon as comfort allows. If patients are still symptomatic after an appropriate period of time, excision of the fragments and remodeling of the lateral process is indicated. Although recovery is often protracted after this operation, there is no significant alteration in subtalar or ankle mechanics and stability (25) (Fig. 108.5)
When the fragment consists of a large displaced articular component, operative reduction is indicated. A lateral subtalar arthrotomy is performed, and access to the fragment is obtained just inferior to the anterior talofibular ligament. Both the subtalar and talofibular articulations can be easily approached from this exposure. Internal fixation can vary, depending on the fracture pattern, but the fragment is most commonly entirely articular. As such, even with small screws, good countersinking is required to ensure that the fixation will not be prominent. Care should be exercised to ensure that both articular surfaces are reduced because rotation of the fragment is common, and one of the articular surfaces may be offset (Fig. 108.6).
Long-term complications primarily involve posttraumatic arthritis. In those patients with larger fragments, a local resection arthroplasty often relieves the symptoms, although a large articular void is created. In recalcitrant cases, subtalar fusion is indicated. It is extremely rare to encounter lateral talofibular facet arthrosis that necessitates an ankle salvage operation (Fig. 108.7).
FRACTURES OF THE POSTERIOR PROCESS
Injuries of the posterior process of the talus are somewhat because of the vulnerable anatomic position of this structure between the tibia and the calcaneus. Other potential reasons for the relative prevalence of this injury are the anatomic variability of both the size and shape of the posterior process and the high incidence of an accessory ossicle in this area (26,27,28 and 29) (Fig. 108.8). The accessory ossicle is most commonly termed an os trigonum. Debate still exists as to whether the separate posterior process represents an ununited center of ossification or a consequence of trauma (30,31,32 and 33). Regardless of the true origin, many patients present with symptoms in this area with varying histories of trauma (32,33).
The mechanism of injury is one of forced plantarflexion of the ankle in which the posterior process is compressed between the posterior malleolus of the tibia and the tuber of the calcaneus (34,35,36 and 37). If the posterior process and the body of the talus are confluent, then the compressive force serves to fracture the tubercle from the talar body. If prior anatomic separation was present at the time of trauma, then the impingement could further separate the two fragments or disrupt the fibrous tissue that binds the two together. In addition, a localized inflammatory condition may be precipitated to the capsular tissues in this area. The confluence and close proximity of the posterior talofibular ligament, the inferior transverse ligament, the posterior talocalcaneal ligament, and the flexor hallucis longus tendon may also influence the type and degree of symptoms or disruption seen at this level. Nevertheless, the acute compressive forces may account for symptoms that can be provisionally
interpreted as an acute fracture (38). It has been suggested that dorsiflexion of the foot at the ankle may result in an avulsiontype injury from the thick array of soft tissue that invests this area (30,31,32,33,34,35,36,37,38 and 39).
interpreted as an acute fracture (38). It has been suggested that dorsiflexion of the foot at the ankle may result in an avulsiontype injury from the thick array of soft tissue that invests this area (30,31,32,33,34,35,36,37,38 and 39).
Impingement of the posterior capsule may incite a local synovitis aggravated by repetitive plantarflexion of the ankle (31,40,41). Sports or activities that encourage a plantarflexed attitude of the ankle are most commonly implicated. This includes ballet, soccer, or other athletic activities in which ankle sprains are common (31,35,42,43,44 and 45).
It may be difficult to differentiate between an actual fracture and impingement mediated by the os trigonum. The temporal association of the trauma with the onset of symptoms is often difficult to correlate. Several authors have stated that many patients with symptoms had distant antecedent trauma, although no published report has ever been able to establish a direct cause-and-effect relationship (35,46,47). Fortunately, when symptoms do occur, one seldom needs to determine the true origin of the posterior process fragment or the mechanism of injury because treatment is directed toward the symptoms and is similar regardless of the precipitating factors. Although many patients may exhibit symptoms that are localized to the posterior process of the talus, in clinical practice, it is more unusual to find that the posterior process has actually been fractured (Fig. 108.8).
The most reliable clinical sign in evaluation of an injury to this area is pain with forced plantarflexion of the ankle or the so-called nutcracker sign (35,48). Other less reliable clinical features include pain on direct palpation of the posterior triangle and pain with dorsal excursion or active range of motion of the hallux. This last clinical maneuver has been heralded as a pathognomonic sign, but it yields a high percentage of false-negative results (35). Similarly, the injection of local anesthetic into the ankle joint may not be reliable as a diagnostic technique because both the subtalar and ankle joint capsules are involved, although this practice has been widely described (35,39,44,47,49).
Although radiographs are warranted on initial presentation, confirmatory evidence of an acute fracture or an inflammatory condition may still be lacking. On the lateral view, if one sees an irregular or jagged separation between the body of the talus and the fragment, then a fracture is more likely. Serial radiographs that demonstrate progressive widening of the separation between the structures may also be more suggestive of a fracture (50). If the fragment demonstrates a smooth cortical border, then an os trigonum or an attendant inflammatory process would be favored. The use of comparison radiographs with the asymptomatic foot is of limited value in patients with unilateral symptoms because no correlation exists between the symptoms and the presence or absence of accessory ossicles (51). When the radiographic findings are equivocal, then a technetium bone scan may be useful, particularly if the scan is negative (35,50,52,53 and 54). A negative technetium scan essentially rules out a fracture if the study is performed more than
24 hours after the injury. A positive bone scan is less diagnostic because uptake may occur without acute disruption of osseous integrity (55,56). Perhaps with increased sophistication of magnetic resonance imaging (MRI), more definitive diagnoses may be made (57,58).
24 hours after the injury. A positive bone scan is less diagnostic because uptake may occur without acute disruption of osseous integrity (55,56). Perhaps with increased sophistication of magnetic resonance imaging (MRI), more definitive diagnoses may be made (57,58).
Treatment of the acute injury almost always involves some degree of immobilization, typically 4 to 6 weeks in a cast, especially if the fragment is large. Early range of motion of the hallux may be used to diminish the chance of tendon adhesions. True radiographic union of the fracture union may not ensue, and a fibrous union will result. Many of the patients with nonunions are asymptomatic, at least until another inciting event occurs. Union is compromised by the finding that the fracture fragments tend to separate when the ankle is dorsiflexed by virtue of traction imparted to the posterior process from the posterior tibiofibular and posterior talofibular ligaments. Contrarily, the thick ligamentous cloak that invests the posterior process usually prevents more significant displacement. It is extremely rare to encounter such an acute fracture that would require operative reduction or early excision unless a large portion of the talar body is involved (59,60). Patients with smaller fragments may also require immobilization for comfort, but one should not expect osseous consolidation of these fragments. Immobilization may be of a shorter interval than in those patients with larger fragments. If symptoms persist, then corticosteroid injections may be helpful. Less commonly, nonsteroidal anti-inflammatory drugs may reduce symptoms to a tolerable level. Other conservative treatment modalities often fail because of the repetitive injury that occurs with full plantarflexion of the ankle.
A small percentage of patients experience persistent pain that may require excision of the fragment or the intact posterior process. The residual symptoms are mostly related to bony impingement of the fragment. Excision can be accomplished through a posterior-lateral or posterior-medial approach (61). If a posterior-lateral approach is employed, then the procedure may be facilitated by placing the patient in the prone or lateral position. Alternatively, the patient may be placed in a supine position with a beanbag or other bolster placed under the ipsilateral hip, but visualization is more difficult. An incision is made just posterior to the peroneal tendons. Dissection is carried through the subcutaneous tissue, with care taken to retract the sural nerve anteriorly. Once the dorsal surface of the calcaneus is identified, deep dissection may proceed medially at this level until the posterior subtalar joint is defined. In the lateral approach, the surgical cavity is deep. The flexor hallucis longus tendon serves as a consistent medial boundary to locate the fragment. Sharp dissection is usually necessary to free the fragment from the posterior aspect of the talus. If confluent with the posterior talus, a small osteotome or rongeur can be used to separate the process from the body of the talus. Careful contouring of the remaining, exposed osseous surface is recommended to ensure that all of the offending bone is removed. Intraoperative imaging is helpful, as there is a tendency to remove less bone than necessary to decompress the area. Occasionally, the sheath of the flexor hallucis longus tendon sheath is violated, but repair is not necessary and is quite difficult because of the limited corridor of exposure (49,56,62,63). If the procedure is performed from the posterior medial approach, the patient can remain supine, but one must dissect past the posterior tibial neurovascular bundle and the flexor hallucis longus tendon to access the fragment.
Arthroscopic excision is also possible from either a prone or lateral position with a two-portal approach. Two posterior lateral portals between the Achilles and the peroneal tendons are most commonly utilized. A posterior-medial portal can be developed medial to the Achilles tendon, but there is more risk of injury to the neurovascular structures (56,64,65,66,67 and 68). Regardless of the method of excision, complications are uncommon.
POSTERIOR-MEDIAL FRACTURES
Numerous case reports of fractures of the posterior-medial aspect of the talus have been published (43,69,70,71,72,73 and 74). Various mechanisms of injury can cause this fracture pattern, but significant functional deficits can result afterward because of the biarticular involvement. Many patients have a delay between the injury and the diagnosis (70,74,75) because of the subtle radiographic appearance
of the fracture and of the difficulty of demonstrating this area of the talus on standard radiographs. The use of special oblique views has been suggested for optimal visualization (76,77).
of the fracture and of the difficulty of demonstrating this area of the talus on standard radiographs. The use of special oblique views has been suggested for optimal visualization (76,77).
CT is diagnostic if there are any subtle radiographic findings (30,69,70,71 and 72,75) (Fig. 108.9).
Treatment of this fracture depends primarily on the size of the fragment, the degree of displacement, and time elapsed since the injury. In the acute setting, operative reduction is generally indicated except if the fracture is nondisplaced (43,72). Cast immobilization and careful surveillance by radiograph can provide a satisfactory outcome in the latter situation. However, the intra-articular nature of the injury may make early operative intervention the treatment of choice (69,70,73). Open reduction and internal fixation is carried out unless the fragments are grossly comminuted (71).
When the diagnosis is delayed, the rationale for treatment changes slightly, and the degree of existing articular damage should be factored into the process. If the fracture fragment is large, but viable, some attempt at delayed internal fixation may be attempted. However, some patients may have displaced, degenerative fragments. In this circumstance, excision of the fragments may be preferable (43,70,74). The surgical approach to the posterior-medial aspect of the talus is through an incision overlying the tarsal canal. The flexor digitorum longus is retracted anteriorly to allow access to the fracture fragment (69). Alternatively, a medial malleolar osteotomy can be performed. Results of treatment of either the acute or latent presentation are generally favorable, although a large series of patients with this injury has not been described (43,69,70,71,72,73,74 and 75).
FRACTURES OF THE TALAR NECK
The mechanism of injury of talar neck fractures has been described as a forced dorsiflexion in which the anterior lip of the tibia hits the sulcus on the dorsal aspect of the talar neck (78,79 and 80). The relatively sharp anterior edge of the tibia cleaves away the head and neck from the remaining portion of the body. If the force is continued, sequential dislocation of the various joints of the hindfoot and ankle will occur. The sequential failure and dislocation also form the basis of the Hawkins classification for talar neck fracture-dislocations (81). Furthermore, based on the injury pattern, the prognosis of these injuries may be more accurately assumed.
Critical radiographic observation of a large series of fracture dislocations of the talus has cast some doubt on the exact mechanism of injury. If one assumes that the morbid forces are propagated from the anterior lip of the tibia to the transverse sulcus anterior to the talar dome, then most, if not all, fracture lines would occur at this level, or at least one would observe some damage to the area with a potential loss of the blood supply to the head and neck of the talus. However, the primary fracture develops posterior to the transverse sulcus, and the distinct rarity of an AVN of the head and neck portion renders this exact mechanism unlikely. Intuitively, the mechanism would seem to be more the result of a direct axial load, with the anterior tibia serving as the weapon of insult (82). This would account for the large percentage of patients who have primary fracture lines well posterior to the junction of the anterior tibia and dorsal talar neck (Fig. 108.10). In addition, there is rarely an associated compression or shear fracture of the anterior tibial plafond. Even though the ankle may be plantarflexed at the time of impact, there is an erroneous assumption that there is a dorsiflexion thrust to the foot that forces the talus through its range of motion to produce the fracture (8,78,83).
Recently, motor vehicle accidents have been the most frequent cause of talar neck fractures (84,85 and 86). With the increased prevalence in the use of seat belts and air bags, the foot is more vulnerable to axial load during impact. It is highly unlikely that a normal but excessive arc of ankle motion would ensue after impact. Rather, a shearing type of mechanism mediated by an axial load with the foot in a slightly plantarflexed but locked position is the more likely event. These shearing-type injuries may possibly create fractures of the body of the talus, as opposed to a fracture variant of the neck of the talus (83) (Fig. 108.10).
Classification
The universally accepted convention Hawkins classification (81) scheme is based on the correlation between the number of dislocated joints and the likelihood of AVN. Furthermore, treatment can be directed on the basis of the classification (87) (Fig. 108.11).
If the talar neck is fractured and no further disruptive force is present, then the body of the talus will remain in an anatomic location within the subtalar and ankle joints without ligamentous disruption. This is categorized as a Hawkins type I fracture (Fig. 108.12). The risk of AVN is extremely low because no disruption to the blood supply to the talus has occurred (81).
If additional force is imparted to the foot, the usual event is a dislocation of the posterior facet of the subtalar joint. This is referred to as a Hawkins type II fracture (81). In most instances, the body of the talus is extruded posteriorly, with the intact deltoid ligament serving as a hinge for rotation (6). The body of the talus plantarflexes in the ankle mortise, and the anterior edge of this fragment becomes entrapped behind the superior aspect of the posterior facet of the subtalar joint. In most cases, the lateral process of the talus lodges behind the posterior facet of the calcaneus (Fig. 108.13A). Other variants are possible, depending on the frontal plane position of the talar body after dislocation.
The interosseous talocalcaneal ligament may fail when the fracture line is close to the attachment of this structure. Because one of the major components of the blood supply to the talar body courses through this ligament, a corresponding disruption of the blood supply may exist. The reported incidence of AVN in the type II injury varies considerably, ranging anywhere from 15.8% to 71% (6,7,80,81,87,88,89,90 and 91). Perhaps the discrepancy results from the variability of the specific fracture location, the anatomic variability of the blood supply, and the degree of dislocation. In those fractures that are posterior to the junction of the head and neck of the talus, there may be a decreased incidence of vascular disruption in the sinus tarsi.
In fact, more recent MRI analysis of talar neck fractures suggests all injuries develop AVN to some degree (92). However, the pattern of incomplete AVN trends toward the anterolateral quadrant. (93)
In fact, more recent MRI analysis of talar neck fractures suggests all injuries develop AVN to some degree (92). However, the pattern of incomplete AVN trends toward the anterolateral quadrant. (93)
![]() Figure 108.12 A: A nondisplaced type I injury in pediatric patient. B: A minimally displaced talar neck fracture without dislocation of the subtalar joint. |
Some discussion exists regarding the pathologic distinction between type I and type II fractures (80,81,87,89). Hawkins referred to the completely nondisplaced fracture as type I and to fractures with some displacement as type II. However, it is possible to have a displaced fracture of the talar neck without dislocation of the subtalar joint. The disparity arises from the definition of “displaced” and the stipulation by Hawkins that in a type I injury, “a vertical fracture of the neck of the talus must be undisplaced” (81). Intuitively, displaced fractures without subtalar joint incongruity behave more like type I injuries with
respect to the genesis of AVN. There may be rotatory displacement in the frontal plane that may or may not be obvious on the lateral x-ray. A CT scan is helpful to determine of the exact nature and planar orientation of the displacement (Fig. 108.13B). In addition, an anteroposterior radiograph of the foot will often show varus malalignment of the talometatarsal axis.
respect to the genesis of AVN. There may be rotatory displacement in the frontal plane that may or may not be obvious on the lateral x-ray. A CT scan is helpful to determine of the exact nature and planar orientation of the displacement (Fig. 108.13B). In addition, an anteroposterior radiograph of the foot will often show varus malalignment of the talometatarsal axis.
Continuation of the disruptive forces dislocates the talar body from the ankle mortise. The body of the talus is usually dislocated posteriorly, although other forms of dislocation may be seen in rare cases (94). The combination of an ankle dislocation and a subtalar dislocation constitutes a Hawkins type III fracture dislocation (Fig. 108.14). Although it has not been proven, this injury is presumed to represent a sequential failure of the subtalar joint followed by the ankle joint. This presumption is substantiated by the mere failure to observe patients only with an isolated dislocation of the ankle in combination with a fracture of the neck of the talus. However, a simultaneous dislocation of both joints can conceivably produce the type III pattern.
Uncommonly, a vertical fracture of the medial malleolus occurs with subluxation or dislocation ankle joint. The most likely mechanism is inversion with some degree of axial load. Often, the medial malleolar fragment stays attached to the talus by the deep deltoid pedicle, preserving this important blood supply to the talus in these injuries. It is unclear if this fracture variant lends a more favorable prognosis with respect to AVN.
Injury to the blood supply that traverses the deltoid ligament can either be from extreme torsion or the ligament can fail outright. In either instance, the integrity of the blood supply to the body of the talus from this source is lost. Coupled with the loss of blood supply from the sinus tarsi consequent to subtalar dislocation, this dual loss of blood supply to the body of the talus would virtually ensure that AVN will develop (6,7,80,81,87,95,96). However, Peterson et al (90) reported only a 33% incidence of AVN in nine patients with type III injuries. Kenwright and Taylor (7) reported only a 75% incidence of AVN in four cases of type III injuries. They also reported that none of the tali were misshapen after the avascular event. The reason for the discrepancy between the reported incidence in these small cases series and anatomic logic is obscure. Those cases that do not result in clinically significant avascularity may represent subtotal or focal AVN. Use of more a more rigid definition of AVN (involvement of the entire talus) likely decreased the likelihood of a firm diagnosis in these reports (7,90).
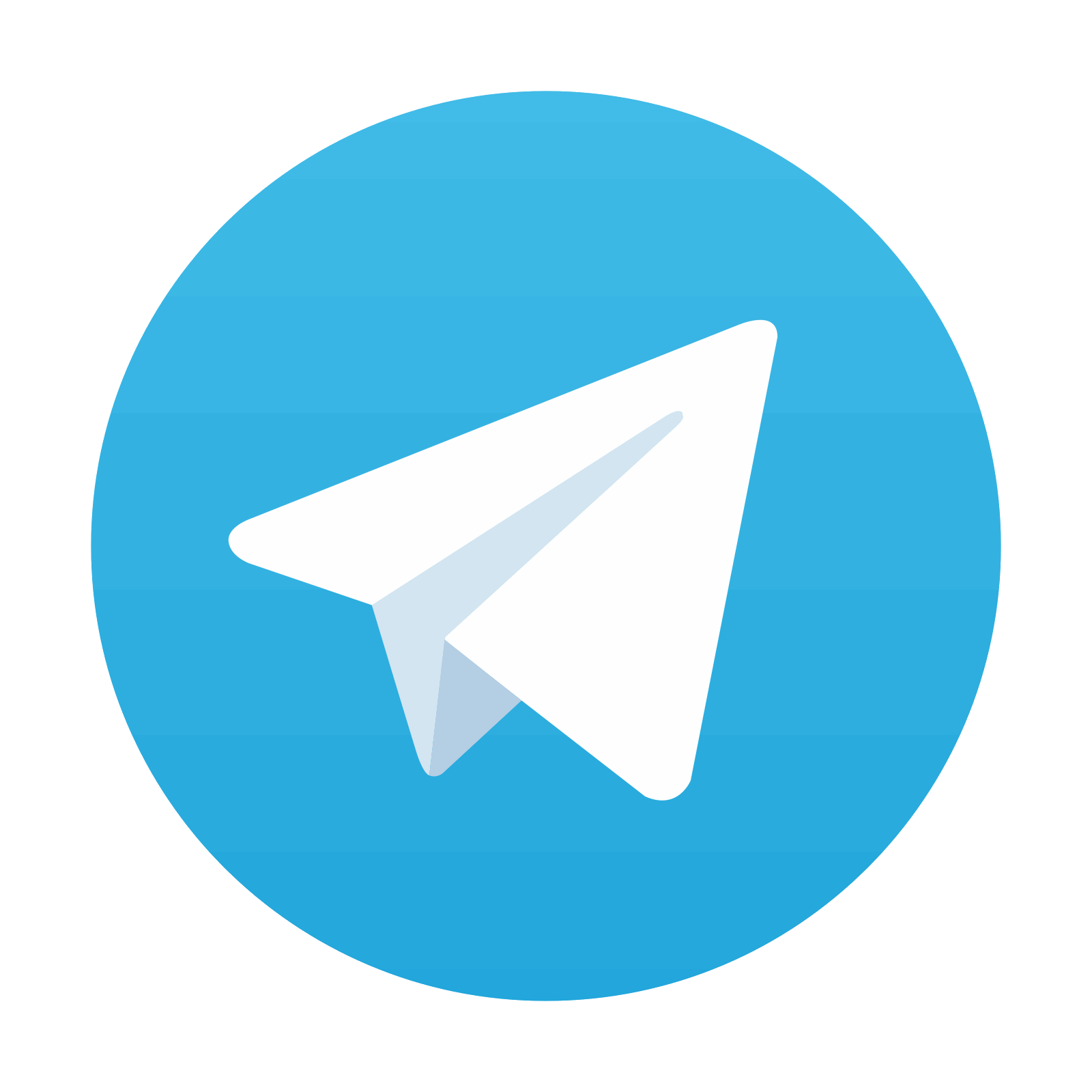
Stay updated, free articles. Join our Telegram channel

Full access? Get Clinical Tree
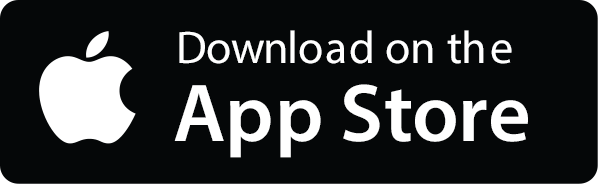
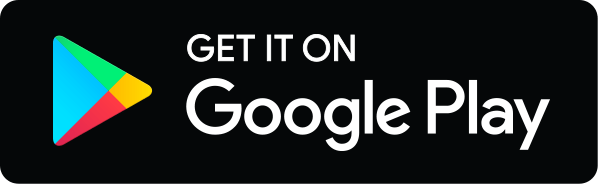
