18
SPORTS CARDIOLOGY
Gregory Cascino and R. Kannan Mutharasan
INTRODUCTION
Exercise can be viewed as the process whereby an individual does mechanical work on the environment to move the environment (weightlifting), move oneself through the environment (swimming), or a combination of both (cycling). In an exercising individual the cardiovascular system plays three important roles: the delivery of oxygen and nutrients to exercising muscle, the removal of waste by-products from exercising muscle, and the dissipation of generated heat through convection.
While a sequential and organized program of exercise can train the cardiovascular system to function more efficiently, vigorous physical activity can also exacerbate or make manifest cardiovascular disease. Thus, within the field of sports cardiology, there are two major clinical concerns: identifying athletes with life-threatening cardiovascular disease before competition, and prescribing safe limits on level of exercise intensity for those individuals with known cardiovascular disease.
Important sex differences exist in both the realm of exercise physiology and cardiovascular pathology. Therefore, this chapter is divided into two sections: sex differences in cardiovascular physiology, and sex differences in cardiovascular disease. The first section begins with a primer on cardiovascular physiology; the second section begins with important considerations when evaluating the athlete with known or suspected cardiovascular disease.
Primer in Exercise Physiology
The body makes acute and chronic adaptations to accommodate the increased metabolic demand of exercise. The cardiovascular system, pulmonary system, musculoskeletal system, central and peripheral nervous systems, and hematologic system all make crucial changes during exercise to preserve cellular oxygenation and acid-base homeostasis. Here we examine the body’s adaptive mechanisms to exercise, with a particular focus on the cardiovascular system, and articulate the gender and sex differences in exercise physiology and cardiovascular disease.
Energy Production
The body’s response to exercise is complex and requires many short- and long-term adaptations. The mechanical work done by muscles during exercise requires energy—energy derived from the high-energy chemical bonds present in adenosine triphosphate (ATP). A variety of machineries exist to regenerate ATP, and different types of exercise use different metabolic mechanisms to generate ATP. Aerobic activity, such as swimming, long-distance running, and cross-country skiing, is characterized by low-intensity, long duration exercise. This type of exercise is supported primarily by aerobic metabolism, which requires oxygen to be present for the generation of energy from sources such as glucose, glycogen, and fat. This process, called oxidative phosphorylation, occurs in the mitochondria of muscle cells. In aerobic metabolism, the cardiopulmonary system must replenish oxygen in working muscles.
In contrast, high-intensity activities such as sprinting, heavy weightlifting, and interval training are supported primarily by anaerobic metabolism. In anaerobic metabolism, anaerobic glycolysis rapidly generates ATP from the breakdown of glucose without requiring oxygen. This process is less efficient than oxidative phosphorylation and cannot be sustained due to the production of lactic acid, which inhibits further glycolysis. Anaerobic glycolysis occurs in the cytosol of muscle cells, in contrast to aerobic respiration that occurs in mitochondria. High-intensity exercise can also utilize an additional metabolic pathway, the creatine kinase reaction, to facilitate rapid production of ATP (1).
Aerobic Respiration
The utilization of oxygen by working tissue is a process that involves three distinct steps—oxygenation, oxygen delivery, and oxygen consumption (Figure 18.1). Inspired oxygen diffuses across the alveolar-capillary membrane from the lungs into the blood (oxygenation). Red blood cells then transport hemoglobin-bound oxygen throughout the body (oxygen delivery) and tissues extract oxygen for use in aerobic respiration (oxygen consumption). Thus, the delivery of oxygen to working tissue is dependent on the function of the lungs, the cardiovascular system, and red blood cells in order to meet the metabolic demands of the body (2). Here we briefly describe each of these key steps and describe some of the short- and long-term adaptations the body’s organ systems make during activity.
Oxygenation. Oxygenation occurs when inspired oxygen diffuses across the alveoli into the pulmonary capillaries. This oxygen becomes predominantly bound to hemoglobin in red blood cells, although some oxygen dissolves in the arterial plasma. The driving force for diffusion of oxygen across the alveolar-capillary membrane can best be understood by the alveolar gas equation. Mathematically, the alveolar gas equation is defined as PAO2 = PiO2 − (PACO2/RER), where PAO2 is the alveolar partial pressure of oxygen, PiO2 is the partial pressure of inspired oxygen, PACO2 is the alveolar partial pressure of carbon dioxide, and RER is the respiratory exchange ratio, or the ratio between the amount of oxygen consumed (VO2) and carbon dioxide produced (VCO2) in each breath (VO2/VCO2). PAO2 is preserved during exercise by several mechanisms. The minute ventilation is the amount of air that is inhaled or exhaled in 1 minute. Minute ventilation increases incrementally with increased metabolic demand, primarily due to increased tidal volume, or the volume of air inhaled (or exhaled) with each breath (3). With increased minute ventilation, the alveolar ventilation (VA), the component of tidal volume that participates in gas exchange, also increases. Furthermore, the lung bases—areas that typically have low ventilation/perfusion indices—become better ventilated during exercise due to larger tidal volumes. Additionally, the blood flow to the pulmonary capillaries is increased, which increases the amount of surface area for oxygen to diffuse across. The net result of these adaptations is preservation of the PAO2 despite increased metabolic demand (4).
FIGURE 18.1: Gas transport.
Oxygen Delivery. Oxygen delivery, or DO2, is the rate at which oxygen is transported from the lungs into the peripheral tissue. DO2 equals the product of the cardiac output and the arterial oxygen content (CaO2). Cardiac output in turn is equal to the heart rate in beats per minute multiplied by the stroke volume, or the amount of blood ejected by the heart during each beat. Arterial oxygen content is defined as the amount of oxygen bound to hemoglobin plus the amount of oxygen dissolved in arterial blood. Since most oxygen is bound to hemoglobin, with only a small contribution from oxygen dissolved in arterial blood, oxygen content is mostly dependent on the hemoglobin concentration and the oxyhemoglobin saturation. DO2 is augmented during activity primarily through increases in cardiac output.
The Frank-Starling mechanism is an important model for understanding the heart’s ability to augment its forces of contraction, and therefore stroke volume, in the setting of increased workload. The Frank-Starling mechanism states that contractility increases when end-diastolic volume (EDV), or the heart’s preload, increases (5). This can best be understood on the level of the myocardial cells. As the heart fills with more blood, the nature of the actin and myosin filament interaction changes in a manner that results in increased contractility. It is believed that during exercise, left ventricular (LV) filling is increased due to greater negative intrathoracic pressure and the pumping action exhibited by exercising limbs (6). As a result, the left ventricular-end-diastolic volume (LVEDV) increases, augmenting the contractility and thus stroke volume.
The systemic circulation also makes key adaptations that enhance oxygen delivery. Blood flow is preferentially redirected to working muscles and away from less metabolically active organs, such as the gastrointestinal system and the kidneys. Although systolic blood pressure increases with activity, diastolic blood pressure changes little. Furthermore, the rise in mean systemic blood pressure is less than expected given the increase in cardiac output, indicating a decrease in systemic vascular resistance (SVR) (7). Exercise performance has been correlated with the ability to decrease SVR (8).
Oxygen Consumption. Once oxygen is delivered to working muscles, it must be extracted for use in aerobic respiration. Oxygen consumption, or VO2, is defined as the rate at which oxygen is taken up by working tissues. The Fick equation is useful for calculating VO2. The Fick equation defines VO2 as the cardiac output multiplied by the amount of oxygen muscles are able to extract from the blood passing through them, quantified by the arteriovenous oxygen content difference, (a-v)O2. During exercise, VO2 increases due to elevated cardiac output and increased (a-v)O2. The (a-v)O2 is expected to increase during times of strenuous exercise, as working muscles are extracting more oxygen. This is facilitated by changes in the oxyhemoglobin dissociation curve (9). Local lactic acidosis during strenuous activity causes a rightward shift in this curve, decreasing affinity between hemoglobin and oxygen and increasing the availability of unbound oxygen for working muscles (10).
Anaerobic Threshold. The anaerobic threshold (AT) is the point at which aerobic metabolism transitions to anaerobic metabolism. This occurs at the exercise intensity when the partial pressure of oxygen is no longer sufficient for a given VO2. At this point, several physiological responses occur. Muscle cells use anaerobic glycolysis to generate ATP for energy use. Lactic acid is produced as a result, leading to increased metabolic acidosis, increased ventilatory drive, and increased fatigue. The anaerobic threshold varies dependent on the fitness level of the athlete, with higher-level athletes being able to meet a higher VO2 before entering anaerobic metabolism. Several mechanisms have been proposed to explain how AT can be increased, including enhanced oxygen delivery to working skeletal muscle fibers, increased rate of lactate clearance during exercise, and biochemical alterations within skeletal muscle that reduce lactate formation (11). AT can be increased by high-intensity interval training and moderate-intensity continuous training.
Long-Term Adaptations of Exercise Training
Exercise training confers long-term adaptations throughout many of the body’s organ systems. The cardiovascular system, pulmonary system, and musculoskeletal system all exhibit adaptive responses that improve efficiency of energy utilization during exercise. Cardiac hypertrophy, or “athlete’s heart,” is a physiological adaptation to increased workload. In contrast to pathological hypertrophy, physiological hypertrophy is associated with normal or augmented contractility (12). The type of physiological hypertrophy is dependent on the type of exercise. Endurance training is associated with LV eccentric hypertrophy, where both left ventricular cavity and wall thickness are increased from the volume overload produced during exercise. Strength training, on the other hand, is associated with left ventricular concentric hypertrophy in response to increased afterload during isometric exercise, where there is increased wall thickness but no change in cavity size (13).
Endurance training improves stroke volume, cardiac output, and maximal oxygen uptake (VO2max), defined as oxygen consumption at maximal heart rate. VO2max is the fastest rate at which the body can utilize oxygen during activity and is widely accepted as the best indicator of overall cardiopulmonary fitness and functional aerobic capacity. During the first stage of aerobic exercise intensity, from rest to approximately 40% VO2max, cardiac output is augmented primarily by increased stroke volume, with a moderate increase in heart rate. Cardiovascular response differs between trained and untrained individuals at the second stage of aerobic exercise intensity, from 40% VO2max to 90% VO2max. In untrained individuals, peak contractility is reached at 40% VO2max. Thus, any increase in cardiac output after this threshold is reached is a result of increased heart rate. Well-trained individuals, on the other hand, are able to augment stroke volume up to VO2max. During the third and final stage of aerobic exercise, from 90% VO2max to VO2max, cardiac output increases due to a sharp increase in heart rate. This increased heart rate reduces left ventricular filling time, so stroke volume becomes slightly decreased. During this final stage of aerobic exercise, untrained individuals rely primarily on anaerobic metabolism (14).
The lungs and skeletal muscle also undergo important adaptations in response to endurance training. In skeletal muscle, endurance training confers an increase in mitochondrial size, muscular capillary density, and muscle myoglobin levels. All of these adaptations improve the muscle’s ability to extract oxygen during exercise (15). In the lungs, ventilation (VE) at maximal aerobic exercise increases with training experience (16). Untrained individuals primarily augment VE by increasing respiratory rate. The trained individual, on the other hand, can increase both tidal volume and respiratory rate. The augmentation of VE exceeds the amount of blood that circulates through the lungs at exercise and thus is usually not the limiting factor during maximal aerobic metabolism.
Sex Differences in Exercise Physiology
The cardiovascular system, pulmonary system, hematologic system, and metabolic system exhibit sex-specific differences in function and morphology in response to activity.
Sex Differences in Cardiovascular Physiology
There are several important sex-related differences in cardiovascular physiology. Males and females have different cardiac structure and hemodynamic response to exercise. Cain et al. used cardiac MRI to measure left ventricular dimensions in healthy volunteers. Compared to adult females, adult males had higher body surface area (BSA)-adjusted end-diastolic volumes (EDV) and end-systolic volumes (ESV) with similar stroke volumes and ejection fractions (EF) (17). No sex differences were seen in the younger (11–15 years old) age group, suggesting that the difference in left ventricular dimensions may be due to differences in sex hormone profiles between the sexes. Another study evaluated echocardiographic parameters in males and females at rest and at peak VO2 (18). In males and females with equal peak VO2, males were found to achieve higher exercise workload while females were able to reach higher heart rate. The two groups had similar left ventricular stroke volumes and cardiac outputs when indexed for BSA. While indexed EDV and ESV were smaller in females, females had higher early diastolic velocity, suggesting enhanced lusitropy, or relaxation function of the heart. Indexed stroke volume was the sole multivariable predictor of peak VO2 in men. Markers of increased left atrial pressure—such as raised early diastolic velocity and short diastolic filling time—were predictions of peak VO2 in women. These findings suggest that the cause of exhaustion in the athlete differs according to gender. While stroke volume is the main limiting factor in males, raised left atrial pressure—and subsequent pulmonary venous hypertension—may be the main cause of fatigue in females. This pulmonary venous hypertension may be exacerbated during endurance exercise in females, which may result in LV hypertrophy, a decrease in LV cavity size, and further increase in left atrial pressure. This could partially explain the differences in endurance exercise athletic performance between the sexes.
In addition, males and females exhibit differences in the heart’s adaptation to progressive overload. Cardiac remodeling (meaning the change in heart size and wall thickness) is more pronounced among male athletes. When compared to male athletes of similar age and training regimen, females were found to have 23% less left ventricular wall thickness and 11% smaller left ventricular size (19). Data from various animal studies also demonstrate sex-specific regulation of physiological hypertrophy in response to exercise. In these studies, females were shown to have an increased hypertrophic response to training load compared to males. It was hypothesized that sex hormones, particularly estrogen and testosterone, were important in modulating the development and progression of physiological cardiac hypertrophy (20). It has also been postulated that substrate utilization of myocardial cells may impact the sexual dimorphism of physiological cardiac hypertrophy. Animal studies have shown increased plasma free fatty acid levels and augmented lipolysis in females after training when compared to males, indicative of preferential fatty acid utilization (21). Fatty acid utilization as the primary fuel source during sustained activity has been suggested as one of the important mechanisms distinguishing physiological from pathological cardiac hypertrophy (22).
Females have a lower VO2max when compared to males. Astrand (23) reported a 17% lower VO2max for 18 female students when compared to 17 male students of comparable stature, while Bruce et al. (24) determined that females had 23% lower VO2max when compared to male counterparts. The gender-related difference in VO2max is probably related to differences in left ventricular dimensions. Sex differences in VO2max, however, are reduced to about 10% when comparing elite athletes. And if VO2max is adjusted for fat-free mass, the differences disappear in some studies (25). Nonetheless, studies have consistently shown increased endurance performance in athletic competition in men compared to women. This is thought to be due to greater VO2max, lower body fat percentage, and higher hemoglobin count (26) in men.
Sex-related cardiovascular differences are also demonstrated during anaerobic exercise. The peak Wingate anaerobic test is used to measure anaerobic capacity and peak anaerobic power. At peak Wingate anaerobic test, Sagiv et al. (27) showed that males had significantly higher values of cardiac output and stroke volume, while (a-v)O2, VO2, and %VO2 out of total energy utilized were significantly lower when compared to females. Males also had a significantly lower resting heart rate. The data suggest that women had a significant increase in oxygen extraction during anaerobic activity, which partially compensated for lower cardiac output. Another study demonstrated that although males had higher absolute values in anaerobic capacity and power, statistical significance diminished when indexed with fat-free body mass (28).
Sex Differences in Pulmonary Physiology
In addition to the cardiovascular system, there are also several sex-specific differences in pulmonary function during exercise, which are largely a product of hormonal and morphological differences between males and females. Sex hormones play a key role in the pulmonary response to exercise. The reproductive hormones progesterone and estrogen can influence vascular volume dynamics, ventilation, substrate metabolism, and thermoregulation (29). Increased estrogen levels can increase fluid retention and therefore blood volume, which could potentially affect gas exchange in the lung (30). With the exception of possible improvement in endurance during the luteal phase, there do not seem to be conclusive data on the effect of the menstrual cycle on athletic performance (26).
Structural pulmonary differences have also been noted between males and females. Height-matched men have larger diameter airways (31), larger lung volumes, and increased diffusion surface areas compared with postpubertal women (32). It has been hypothesized that gender differences in diffusing capacity of the lung can be explained by these differences in surface area and airway diameter. Studies indicate that during heavy exercise, women experience increased work of breathing and greater expiratory flow limitation compared to men. It is plausible that respiratory muscle fatigue could adversely impact aerobic capacity and exercise tolerance in women compared to men, consistent with differences in airway diameter. These findings suggest that respiratory limitation to oxygenation may play a greater role in determining exercise tolerance in women compared to men (29).
Some studies (33,34) indicate that women experience exercise-induced arterial hypoxemia (EIAH) more frequently than males. Harms et al. (34) found that women developed EIAH, defined as greater than 10 mmHg decrease in PaO2 from rest to exercise, across all levels of aerobic fitness and exercise intensity. The etiology of EIAH is thought to be multifactorial. Oxygen diffusion limitation, inadequate hyperventilation, and ventilation-perfusion inequality are thought to contribute to the incidence of EIAH (33). It was hypothesized that EIAH was also due to smaller lung volumes and airway diameters with resultant mechanical constraints (e.g., expiratory flow limitation) during exercise. This study demonstrated that reversing these mechanical restraints with heliox gas partially reversed EIAH in females who developed expiratory flow limitation. An additional study demonstrated that even a small amount of EIAH had a significant detrimental effect on VO2max in well-trained female athletes (35). Furthermore, VO2max improved when the exercise-induced hypoxemia was corrected with increased fraction of inspired oxygen (FiO2).
Sex Differences in Hematologic Physiology
The hematologic system, critical for the transportation of oxygen to working tissues, is also influenced by sex. On average, men have mean hemoglobin levels approximately 12% greater than women. The sex difference in hemoglobin levels is thought to be a result of the activity of sex hormones in erythropoiesis. Androgens stimulate erythropoietin production in the kidney and have a direct stimulatory effect in association with erythropoietin in the bone marrow. Estrogen in females, on the other hand, has been shown to have an inhibitory effect on erythropoiesis (36). As a result of higher mean hemoglobin levels, men have a higher oxygen-carrying capacity compared to women, which allows for greater aerobic capacity.
At the center of the heme moiety in hemoglobin is elemental iron, responsible for the binding of oxygen and allowing for its transport. Athletes may have low iron stores through a variety of mechanisms. Iron-deficiency anemia has been observed in athletes after endurance exercise due to hemolysis and iron losses from gastrointestinal bleeding. Furthermore, iron absorption can be impaired due to the release of inflammatory cytokines in response to physical activity (37). Premenopausal females are at increased risk of iron-deficiency anemia compared to men due to blood losses from menstruation, which represent a significant source of iron excretion. Anemia is believed to affect over 40% of pregnant women and 30% of nonpregnant women as a result (38). Ostojic and Ahmetovic (39) found a high prevalence of iron deficiency anemia among female athletes from across different sports, with similar incidence in individuals independent of their training volume. Anemia in the athlete can impair tissue oxygenation, which can in turn lead to a decrease in endurance, a decrease in aerobic adaptation and metabolic responses, and increased muscle fatigue (40).
The oxygen-binding characteristics of red blood cells are also sex-dependent. Humpeler et al. (41) found that P50 values (the partial pressure of oxygen at 50% oxygen saturation of hemoglobin) were significantly higher in sexually mature women than in men, indicating less affinity between hemoglobin and oxygen. This was due to a significantly elevated level of 2,3-diphosphoglycerate (2,3-DPG) observed in women. A rightward shift in the oxyhemoglobin-dissociation curve is induced by 2,3-DPG, favoring the deoxygenated state of hemoglobin and increasing availability of oxygen for utilization in peripheral tissues. Interestingly, no difference in oxygen affinity was found before puberty and post-maturity in females. This suggests a sex hormone and maturation-induced influence in the development of the oxygen transport system, with estrogens favoring a decrease in oxygen affinity of hemoglobin. The physiological consequences of the gender-related difference in oxygen affinity are unclear, though they may be beneficial as an adaptation to tissue hypoxia or in cardiovascular disease (41).
Sex Differences in Metabolism
It is believed that men have greater reliance on anaerobic glycolysis when compared to women. Men have greater muscular force of contraction with an associated greater metabolic demand. This force of contraction may cause local compression of the vascular bed, reducing the availability of oxygen and increasing the utilization of anaerobic metabolism to generate energy (42). Interestingly, greater reliance on anaerobic glycolysis by men has been observed under similar oxidative conditions as compared to women. Multiple studies have demonstrated that women exhibit greater resistance to fatigue than men when engaging in submaximal, isometric exercise. It was hypothesized that this difference in fatigue was related to difference in utilization of metabolic pathways. Russ and Kent-Braun (42) tested this hypothesis by having healthy young males and females perform 4 minutes of intermittent muscular contraction under free-flow and ischemic conditions. Females exhibited less fatigue under free-flow conditions, but comparable fatigue during ischemia. This suggests that women may have what is known as an “oxidative advantage” compared to men, whereby they are more effective in utilizing oxidative phosphorylation during muscular activity (43).
SEX DIFFERENCES IN CARDIOVASCULAR DISEASE
General Considerations in the Cardiac Evaluation of the Athlete
The Symptomatic Athlete
Exercise has numerous health benefits, including reducing insulin resistance (44), coronary heart disease (45), cardiovascular events (46), and all-cause mortality (47). Despite the long-term health benefits associated with regular activity, cardiovascular events such as arrhythmia, myocardial infarction, and sudden cardiac death can occur during or immediately after exercise. Cardiovascular disease can also present as a functional limitation of the athlete, with a reduction in exercise tolerance and athletic performance. This functional limitation can also manifest clinically as progressive fatigue, dyspnea on exertion, chest pain, pre-syncope/syncope, and palpitations. As these are relatively nonspecific symptoms, the astute clinician must have a high index of suspicion when evaluating an athlete with exercise-related symptoms and perform a careful history, physical examination, and diagnostic evaluation in order to exclude potentially life-threatening cardiovascular disease processes.
Sudden Cardiac Death
Of the negative outcomes associated with cardiovascular disease in the athletic population, sudden cardiac death (SCD) is the most dreaded. Thus, much of the evaluation of the symptomatic athlete focuses on assessing risk of SCD in addition to understanding any functional limitations imposed by cardiovascular disease. Exercise-related SCD is defined as death occurring within 1 hour of participation of sports, and it is estimated to occur in one to five cases per 1 million athletes per year (48). It is the leading cause of death among athletes (49). The most common sequence of events leading to SCD is thought to be degeneration of ventricular tachycardia (VT) into ventricular fibrillation (VF), during which disorganized ventricular contractions fail to pump blood effectively, causing a pulseless state (50). The underlying cause of SCD differs depending on the age of the athlete. SCD in “masters athletes” older than 35 is most often related to coronary heart disease with myocardial infarction, while SCD in younger athletes is primarily due to hereditary cardiovascular disorders (51). The incidence of SCD is much higher in men than in women, with men accounting for an estimated 75% of all SCD. Men also have a 50% higher age-adjusted rate of SCD (52). This is due to multiple different factors, including difference in sex hormones as well as rates of participation in different sports.
Return-to-Play Guidelines
The presence of cardiovascular disease elevates the risk of SCD. Many cardiovascular diseases limit or may even preclude participation in various sports. Clinicians are often confronted with the decision of whether to advise athletes to return to play (RTP) after a cardiovascular condition is discovered, either by medical history, routine pre-participation screening, or after a cardiac event has occurred. In the United States, the 36th Bethesda Conference Eligibility Recommendations for Competitive Athletes with Cardiovascular Abnormalities are the most often-used guidelines to make this determination.
Sex Differences in Specific Cardiovascular Diseases
Cardiovascular Disease in Athletes
Broadly, cardiovascular diseases that affect athletes can be grouped into three different categories: arrhythmogenic disorders, structural heart disease, and coronary heart disease (Figure 18.2). In this section we discuss various cardiac disorders that can affect athletes by affecting athletic performance or by putting them at risk for SCD. We then discuss important known sex and gender differences in these various cardiovascular disorders and point out areas where literature is lacking. In general, we restrict our discussion to disorders with known gender and sex differences in either prevalence or outcomes, unless the disorder is common.
Arrhythmogenic Disorders
Arrhythmogenic disorders with important gender-related differences include arrhythmogenic right ventricular cardiomyopathy, long QT syndrome, Brugada syndrome, catecholaminergic polyventricular tachycardia, Wolff-Parkinson-White syndrome, and supraventricular tachycardias.
Arrhythmogenic Right Ventricular Cardiomyopathy. Arrhythmogenic right ventricular cardiomyopathy (ARVC) is an autosomal dominant genetic disorder characterized by right ventricular enlargement and dysfunction as well as life-threatening ventricular arrhythmias. The pathologic hallmark of ARVC is fibrofatty infiltration of the myocardium. ARVC is the leading cause of SCD in athletes in the Veneto region of Italy, accounting for approximately one fourth of events. The incidence of SCD in athletes with ARVC is estimated to be 0.5/100,000 persons/year (53). The risk of sudden death with ARVC is about 5.4 times higher during competitive sports than during sedentary activity. It is theorized that engaging in physical activity acutely increases right ventricular afterload, causing ventricular cavity enlargement and stretching of diseased myocardium, which may precipitate ventricular arrhythmias and favor reentrant circuits (18). ARVC has a typical EKG appearance with T-wave inversions in the right precordial leads beyond V1. The diagnosis may be confirmed with noninvasive imaging studies such as echocardiography or cardiac MRI. Endomyocardial biopsy may be pursued if imaging studies are equivocal. Due to the high risk of SCD during competition, the diagnosis of ARVC in an athlete leads to disqualification from sports participation.
The prevalence of ARVC is higher in males than females. Bauce et al. evaluated 171 consecutive patients fulfilling the ARVC diagnostic criteria. In this study 71% of the patients were males and, in addition, the men had larger right ventricular (RV) volumes, lower RV ejection fraction, and more severe LV involvement (54). Despite the gender-associated differences in disease prevalence, there was no significant difference between genders in the incidence of life-threatening ventricular arrhythmias. The reason for these gender-related differences remains unclear.
FIGURE 18.2: Cardiovascular diseases in athletes.
Long QT Syndrome. The long QT syndrome (LQTS) is an inherited ion channel disorder characterized by prolongation of the QT interval on EKG and a predilection for torsades de pointes polymorphic ventricular tachycardia. LQTS is characterized by variable penetrance: while some patients remain completely asymptomatic, others experience syncope, seizures, or SCD (6). Several genetic mutations have been implicated in LQTS, though the most frequently encountered—LQT1, LQT2, and LQT3—contribute to about 99% of all genotyped cases (55). The EKG is almost always abnormal in LQTS, demonstrating a prolonged QT interval. The heart is structurally normal in most patients, and these syndromes are often clinically silent prior to a catecholamine surge and SCD in times of extreme stress (49).
LQTS has a distinct sex-specific prevalence, with females representing approximately 70% of all congenital cases. Furthermore, female sex itself is an independent risk factor in the development of arrhythmias in LQTS (56). Interestingly, the risk of aborted cardiac arrest or SCD with LQTS is age-dependent. Boys are at three- to fourfold higher risk for cardiac events compared to females. After puberty, a sex risk reversal occurs. Adult women aged 18 to 40 have a higher risk and higher cumulative probability of cardiac events as compared to men. The age-dependent incidence of cardiac events between genders is likely related to shortening of the QT interval after puberty in boys attributed to elevation in testosterone levels (57).
Brugada Syndrome. Brugada syndrome is an inherited arrhythmia disorder characterized by coved-type ST elevations in the right precordial leads. This disease typically presents as non-prodromal syncope, aborted cardiac arrest, or SCD. Though the prevalence of Brugada syndrome is relatively low, it accounts for at least 20% of SCD cases in patients with structurally normal hearts (58). There is a stark contrast in prevalence of Brugada syndrome between genders, with the disease affecting eight- to tenfold more males than females (59). The exact pathophysiology of this gender discrepancy is not completely understood, but it is thought to be a result of sex hormones, namely testosterone, affecting ventricular repolarization (59).
Catecholaminergic Polymorphic Ventricular Tachycardia. Catecholaminergic polymorphic ventricular tachycardia (CPVT) is a heritable channelopathy that classically manifests as syncope or SCD during exercise, emotion, or stress (60). CPVT is typically associated with a normal resting EKG and a structurally normal heart and only becomes suspected with exercise- or catecholamine-induced ventricular ectopy. Mutations in the RyR2-encoded cardiac ryanodine receptor 2/calcium release channel represent the most common genetic subtype of CPVT and account for 50% to 60% of cases. These mutations are thought to result in the excessive release of calcium during sympathetic stimulation. This results in calcium overload, delayed depolarization, and ventricular arrhythmias (60). The majority of cardiac events occur during childhood, with more than 60% of individuals experiencing syncope or cardiac arrest by the age of 20 (61). Gender is an important risk factor in the etiology and pathogenesis of CPVT. Priori et al. (60) performed genetic mutation screening in a series of patients with the clinical phenotype of CPVT as well as their family members. Genotype-phenotype analysis showed that male sex was a strong risk factor for syncope in patients with RyR2 mutation. Patients with an identified RyR2 mutation were also noted to have earlier onset of symptoms. Furthermore, there was a strong predominance of symptomatic females among patients with nongenotyped CPVT. Tester et al. (62) demonstrated that most RyR2-positive patients were males, while most RyR2-negative patients were females. The exact pathomechanism behind this gender discrepancy remains unclear.
Wolff-Parkinson-White and Atrioventricular Reentrant Tachycardia. In Wolff-Parkinson-White (WPW) syndrome, an accessory pathway exists that connects the atria and ventricles, allowing for electrical activity to bypass the atrioventricular node. This accessory pathway allows for the action potential propagated to the ventricles to “reenter” the atria in an arrhythmia called atrioventricular reentrant tachycardia (AVRT). Clinically, this rhythm disturbance can manifest as palpitations, lightheadedness, or syncope. Although SCD is rare in WPW, atrial fibrillation is a particularly dangerous rhythm for patients with an accessory pathway since the accessory pathway allows for direct conduction of atrial fibrillation waves to the ventricles, bypassing the atrioventricular node, which normally slows the signal. This direct one-to-one conduction can drive ventricular tachycardia, which can in turn degenerate into ventricular fibrillation. The classic EKG finding of WPW is shortened with PR interval with a slurred upstroke in the QRS complex, known as a delta wave. A relatively long atrioventricular (AV) conduction time favors the manifestation of pre-excitation in WPW and may play a key role in the clinical significance of an accessory pathway (63). There is also a gender difference in the native conduction system of the heart. Men have longer PR, atrial-His, and His-ventricular intervals as well as longer AV block cycle lengths than women (56). Because of these longer conduction intervals, arrhythmia via accessory pathway, as is seen in WPW and AVRT, is more common in men compared to women. Patients with recurrent or severe symptoms related to WPW can be successfully treated with radiofrequency ablation (RFA) of the accessory pathway.
Supraventricular Arrhythmias. Supraventricular arrhythmias are rhythm disturbances that arise from the atria or the atrioventricular node. These rhythm disturbances are not generally life-threatening, but can cause pre-syncope, syncope, dyspnea, palpitations, and impaired exertional tolerance in the athlete. The most common cause of sustained supraventricular tachycardia (SVT) in the athlete is AV nodal reentrant tachycardia (AVNRT) (64). In AVNRT, a reentrant circuit exists within the AV node, allowing for rapid electrical conduction and causing elevated heart rate. AVNRT is twice as common in women as men. This is thought to be related to shorter conduction times and shorter AV block length cycles in females, as previously described. Due to excellent success rates, many electrophysiologists recommend RFA, wherein part of the atrioventricular node is ablated to remove the accessory pathway, for initial treatment of AVNRT (65). RFA is equally effective in both women and men, and more than 95% of cases can be successfully treated with RFA (66). The incidence of SVT is also influenced by sex hormones. In premenopausal women, the number of SVT episodes and symptoms are more pronounced during the luteal phase of the menstrual cycle when progesterone levels are elevated (67).
Atrial fibrillation is another common type of SVT that can impair athletic performance. Atrial fibrillation is characterized by disorganized electrical impulses most often originating from the left atrium near the pulmonary veins. If rapidly conducted, this abnormal electrical activity can cause elevation in heart rate with palpitations, shortness of breath, lightheadedness, and heart failure. Men have a 1.5-fold higher risk of developing atrial fibrillation than women, though the absolute number of women with atrial fibrillation is higher due to increased longevity compared to men. Furthermore, women with atrial fibrillation tend to have a lower quality of life and more symptoms than men (56).
Structural Heart Disease
Hypertrophic Cardiomyopathy. Responsible for approximately 26% of all SCD events in U.S. athletes, hypertrophic cardiomyopathy (HCM) is the most common cardiac abnormality leading to SCD (68). HCM is an autosomal dominant disease characterized by gross myocardial hypertrophy, contractile dysfunction, abnormal systolic anterior motion of the mitral valve with left ventricular outflow tract obstruction, and electrophysiological disturbances. The characteristic appearance on pathology is myocyte disarray defined by a marked variation in myocardial cell shape and arrangement. The structural and functional abnormalities of myocyte disarray are thought to lead to aberrant electrical conduction that provides the substrate for abnormal electrical pathways that may degenerate into lethal arrhythmias (69). The clinical manifestations of HCM are variable. The majority of patients live without significant morbidity (70), though progression of the disease is unpredictable. Patients may present with exercise-induced syncope or pre-syncope, progressive dyspnea, angina, or signs and symptoms of heart failure. The first manifestation of HCM may be SCD. The characteristic HCM murmur is a systolic murmur that increases with maneuvers that decrease preload, such as going from squatting to standing or performing the Valsalva maneuver. A high clinical suspicion for HCM with prompt evaluation and diagnosis in the athletic population is critical for surveillance and appropriate management. Since aerobic activity has been linked with SCD in patients with HCM, it is recommended to exclude athletes with HCM from all competitive sports.
As HCM is an autosomal dominant condition, one would expect a fairly equal male-to-female distribution. However, a multicenter study evaluating 969 consecutive patients from Italy and the United States found a 3:2 male-to-female distribution. Furthermore, female patients were older and more symptomatic than male patients upon initial evaluation. Female sex was independently associated with the risk of symptom progression or death from heart failure or stroke when compared with male gender (71). These findings were further corroborated by Terauchi et al. (72), who investigated sex differences in the clinical features of HCM caused by cardiac myosin-binding protein C mutations (MYBPC3). Females with MYBPC3 mutations showed later onset of the disease, but were more symptomatic at diagnosis and had more frequent heart failure events. Another recent genotyped cohort study by Page et al. reported higher disease penetrance in males than females (73). These sex-related differences in disease penetrance and presentation are believed to be due to differences in sex hormones, particularly in estrogen, which is thought to have cardioprotective and myocardial modulating properties.
Congenital Heart Disease. Congenital heart disease encompasses a variety of cardiovascular lesions with variable presentations. Some congenital defects may be clinically overt at birth, necessitating prompt surgical correction, while others may first come to medical attention during sports participation. Congenital heart disease can be categorized by the type of defect present. Shunt lesions include atrial septal defect, patent foramen ovale, ventricular septal defect, and patent ductus arteriosus. Valvular lesions include bicuspid aortic valve, mitral valve prolapse, congenital pulmonic stenosis, and coarctation of the aorta. Cyanotic heart disease includes tetralogy of Fallot, transposition of the great vessels, total anomalous venous return, truncus arteriosus, and tricuspid atresia. Though significant gender-related differences are not observed in many of the congenital heart diseases, there may be some key differences in cardiac outcomes. A study from the CONgenital CORvitia (CONCOR) Dutch national registry evaluated over 7,000 adults with congenital heart disease. Though the male-to-female distribution was similar, the study identified sex differences in select cardiac outcomes. Women had a 33% higher risk of pulmonary hypertension and a 33% lower risk of aortic outcomes (aneurysm, dissection, or aortic surgery), a 47% lower risk of endocarditis, and a 55% lower risk of implantable cardioverter defibrillator use. The risk of arrhythmia also appeared to be lower in females. No sex difference in mortality was found (74). Whether these sex-specific differences in cardiac outcomes have a biologic or genetic basis is yet to be determined.
Aside from cardiac outcomes related to congenital heart disease, there are a few select disease processes with sex-specific differences. Bicuspid aortic valve and mitral valve prolapse have been identified as disease processes with important differences in clinical manifestations and prevalence between males and females.
Bicuspid Aortic Valve. The bicuspid aortic valve is one of the most common congenital heart defects, affecting approximately 1% of the population (75). An athlete with a bicuspid aortic valve is at risk for many potential cardiovascular complications, including aortic stenosis, aortic regurgitation, endocarditis, thoracic aortic aneurysm, and aortic dissection. Some athletes with bicuspid aortic valve may remain asymptomatic, while others may experience symptoms related to these valvular abnormalities, such as exertional dyspnea, dizziness, syncope, palpitations, or atypical chest pain. The physical examination of an athlete with a bicuspid aortic valve may reveal a click best heard at the left lower sternal border followed by a systolic ejection murmur. Transthoracic echocardiography is the most common modality used in the diagnosis of bicuspid aortic valve. The bicuspid aortic valve is more prevalent in males than females, with an estimated 3:1 ratio between the two sexes (75). Most cases of bicuspid aortic valve are thought to be familial—as such, this gender-related difference is thought to be due to a genetic cause.
Mitral Valve Prolapse. Mitral valve prolapse (MVP) is another common congenital valvular abnormality, with an estimated prevalence of 2.4% in the general population (76). Mitral regurgitation is the most common complication associated with MVP, and its severity is the main predictor of left ventricular and left atrial abnormalities (77). MVP is also associated with embolism, arrhythmia, and SCD. The athlete with MVP-associated mitral regurgitation may experience various nonspecific symptoms, such as chest pain, dyspnea on exertion, palpitations, and dizziness. Physical examination may reveal a nonejection click followed by the holosystolic murmur characteristic of mitral regurgitation. Transthoracic echocardiography is typically used to diagnosis MVP and its valvular complications. MVP is more common in women than men. A study at the Mayo Clinic evaluated the clinical characteristics, echocardiographic parameters, and clinical outcomes of 4,461 women and 3,678 men diagnosed with MVP. It found that women had less posterior prolapse, less leaflet flail, and less frequent severe regurgitation than men. Left ventricular and atrial dimensions were smaller in women than in men, but larger when indexed to BSA. In patients with severe regurgitation, women were less likely to undergo cardiac valve surgery. At 15 years, women with no or mild mitral regurgitation had better survival than men, but those with severe mitral regurgitation had worse survival (78). These findings suggest a sex-difference in disease mechanisms associated with MVP. Further investigation is needed to better define the biology behind these differences, as well as implications for athletes.
Marfan Syndrome. Marfan syndrome is an autosomal dominant connective tissue disorder with a wide range of characteristic musculoskeletal, ocular, and cardiovascular manifestations. Athletes with Marfan syndrome are characteristically tall and thin, with disproportionately long limbs and long, thin facies. Joint hyperextension is common among patients with Marfan syndrome. It is important for the sports physician to be familiar with this phenotype so that the risk of SCD in this patient population may be reduced. Marfan syndrome accounts for approximately 2% of SCD in U.S. athletes (79), most commonly because of aortic rupture from progressive aortic root dilatation. MVP or mitral valve degeneration may also occur (80). Athletes with Marfan syndrome are typically asymptomatic—thus, the clinical recognition of this disease is critical in monitoring for silent progression of aortic dilatation. Due to the risk of aortic injury, it is typically recommended that patients with Marfan syndrome avoid isometric exercises, high-intensity activities, or collision sports. No significant sex-related differences have been observed in the incidence or clinical manifestations of Marfan syndrome.
Coronary Artery Abnormalities. Coronary artery abnormalities are the second leading cause of nontraumatic SCD in young athletes (81). This heterogeneous group of diseases includes anomalous origin of the left anterior descending artery, tunneled coronaries, myocardial bridging, coronary fistulae, and single coronary arteries. These abnormalities may lead to transient myocardial ischemia during intense activity, which can cause lethal arrhythmia in the form of VT or VF. About one third of athletes will experience syncope, angina, or exertional dyspnea prior to an SCD event (82). Physical examination is oftentimes normal, and definitive diagnosis is typically made by CT of the coronary arteries, coronary angiography, or cardiac MRI. Aydar et al. (83) retrospectively evaluated coronary angiography in patients referred for left heart catheterization. Females were found to have a significantly higher incidence of coronary artery abnormalities, primarily due to anomalous origin of the left anterior descending artery or circumflex artery. Men were found to have a higher incidence of myocardial bridging, but the incidence of coronary fistula was similar between the two groups. The sex-associated difference in pathophysiology is unclear.
Peripartum Cardiomyopathy. Peripartum cardiomyopathy (PPCM) is a cardiovascular disease unique to females. PPCM is defined as the development of heart failure within the last month of pregnancy or within 5 months postpartum in the absence of any determinable etiology of heart failure and without heart disease prior to the last month of pregnancy (84). The signs and symptoms of heart failure in the peripartum athlete may be similar to normal pregnancy. Dyspnea and tachycardia are the most common clinical features upon presentation (85). A careful physical examination may reveal elevated jugular venous pressure, rales, the presence of an S3 gallop, and characteristic murmurs of tricuspid or mitral regurgitation. Transthoracic echocardiogram is typically used to confirm the diagnosis of PPCM. The prompt initiation of standard heart failure therapy is of critical importance in PPCM, with careful avoidance of potentially teratogenic therapies (angiotensin-converting enzyme inhibitors, for example) if the patient is still pregnant. The prognosis of females with PPCM is variable, with an estimated 1-year mortality of 6% to 10% in the United States (86). Left ventricular function typically recovers within 6 months of diagnosis.
Coronary Heart Disease
Most of the cardiovascular diseases previously discussed present in the younger athlete. SCD in “master’s athletes”—athletes older than 35 years old—is most often related to underlying atherosclerotic coronary heart disease (CHD) with resultant myocardial ischemia and myocardial infarction during activity. It is estimated that 80% of SCD in athletes older than 35 is due to CHD (52). Important sex-related differences are present in the epidemiology, clinical presentation, and outcomes of CHD.
Coronary Heart Disease Risk Factors. Risk factors for the development of CHD include diabetes mellitus, dyslipidemia, hypertension, tobacco use, obesity, and physical inactivity. There are some key gender-related differences in the risk associated with CHD in men and women, however. There is a significantly lower age-associated risk of CHD in women than in men—specifically, the risk of death due to CHD in women is similar to that of men 10 years younger (87). CHD is unusual in the premenopausal woman, particularly in the absence of other strong CHD risk factors. The Women’s Ischemia Syndrome Evaluation (WISE) study demonstrated that young women with endogenous estrogen deficiency had a more than sevenfold increase in CHD risk (88). Estrogens are believed to have several cardioprotective effects, including regulating metabolic factors, inflammation, and coagulation, as well as causing vasodilatation through activity at alpha- and beta-receptors (89). The vasodilatory properties of estrogen are further illustrated by the effect of smoking on CHD risk. Smoking increases the risk of first acute myocardial infarction (MI) in females younger than 50 more than in males (90). Smoking is believed to cause downregulation of estrogen-dependent receptors of the endothelial wall (91). Accordingly, the transition to menopause represents a key CHD risk factor in women. A dramatic rise in the incidence of CHD and the severity of presenting symptoms in females was seen after menopause in the Framingham study (92). The risk associated with menopause cannot be completely attributed to change in sex hormones, however. The Women’s Health Initiative (WHI) study evaluated the use of hormone replacement therapy (HRT) for primary prevention of CHD and found no difference in primary/secondary cardiovascular outcomes or in all-cause mortality (93). The Heart and Estrogen/progestin Replacement Study (HERS) evaluated the use of HRT for secondary prevention in females with known CHD and found no change in rate of CHD events (94).
Other CHD risk factors also exhibit sex differences. Women who have diabetes are more likely to suffer cardiovascular complications than men. A meta-analysis demonstrated that the risk of fatal CHD is 50% higher in females with diabetes compared to males with diabetes (95). The risk of hypercholesterolemia is less in younger females compared to males, though this risk increases significantly after menopause (96).
Coronary Heart Disease Clinical Presentation. The clinical presentation of CHD may differ in women compared to men. In fact, CHD can represent a diagnostic challenge due to the variable clinical presentations associated with it, such as dyspnea on exertion, angina pectoris, and heart failure, or less obvious symptoms like nausea, vomiting, abdominal pain, and fatigue. In the Myocardial Infarction Triage and Intervention (MITI) project, women with MI were significantly more likely to present with atypical symptoms like upper abdominal pain, dyspnea, nausea, and fatigue (97). Furthermore, in a study of over 1 million people in the National Registry of Myocardial Infarction, 42% of women with MI presented without chest pain, compared to 31% of men (98). Additionally, females presenting with classic anginal pain are more likely not to have CHD. Kaski et al. studied a series of patients with syndrome X, defined as angina pectoris, a positive stress test, and a normal coronary angiography. The patients with syndrome X were primarily postmenopausal females (99). Despite this, another study evaluating patients presenting with symptoms of obstructive coronary artery disease found substantial overlap of symptoms in men and women (100).
Coronary Heart Disease Outcomes. Sex differences are appreciated in the outcomes associated with CHD as well. Women with CHD have less of a risk of SCD compared to men. A 38-year follow-up from the Framingham Heart Study evaluated the incidence of SCD in women compared to men. This study found that the risk of SCD was approximately one half that of men in patients with CHD (101). It also demonstrated that about two thirds of SCD in women occurred in the absence of prior overt CHD, compared to a rate of about one half in men. Women with CHD are also more likely to develop congestive heart failure. In a retrospective examination of clinical characteristics, left ventricular ejection fraction, and end-diastolic pressure and volume in a series of patients undergoing diagnostic cardiac catheterization, female sex was an independent predictor of congestive heart failure, despite having a higher ejection fraction. Diastolic dysfunction was thought to be the primary mechanism behind this gender discrepancy (102).
A prospective cohort study across 388 U.S. hospitals found that women presenting with stable angina had higher in-hospital mortality than men, though there was no significant difference in mortality between sexes presenting with acute coronary syndrome (ACS) (103). Furthermore, a retrospective examination of percutaneous coronary intervention (PCI) cases across 22 hospitals found that compared with men, women had higher unadjusted procedural mortality, higher vascular complication rates, and more strokes. When adjusted for clinical risk factors and BSA, women had similar PCI mortality risk when compared to men. Interestingly, women remained at higher risk for stroke, vascular complications, and repeat in-hospital revascularization than men, even after risk adjustment (104). This was believed to be due to smaller body size of women on average.
Thus overall, there are important sex differences between men and women in both coronary heart disease presentation and outcomes. While some of these differences may relate to underlying biological differences between sexes, differences in outcome may also be related to disparate care. These findings highlight the need for physicians involved in medical evaluation of athletes to recognize that fitness in athletes of either gender does not preclude the possibility of coronary heart disease.
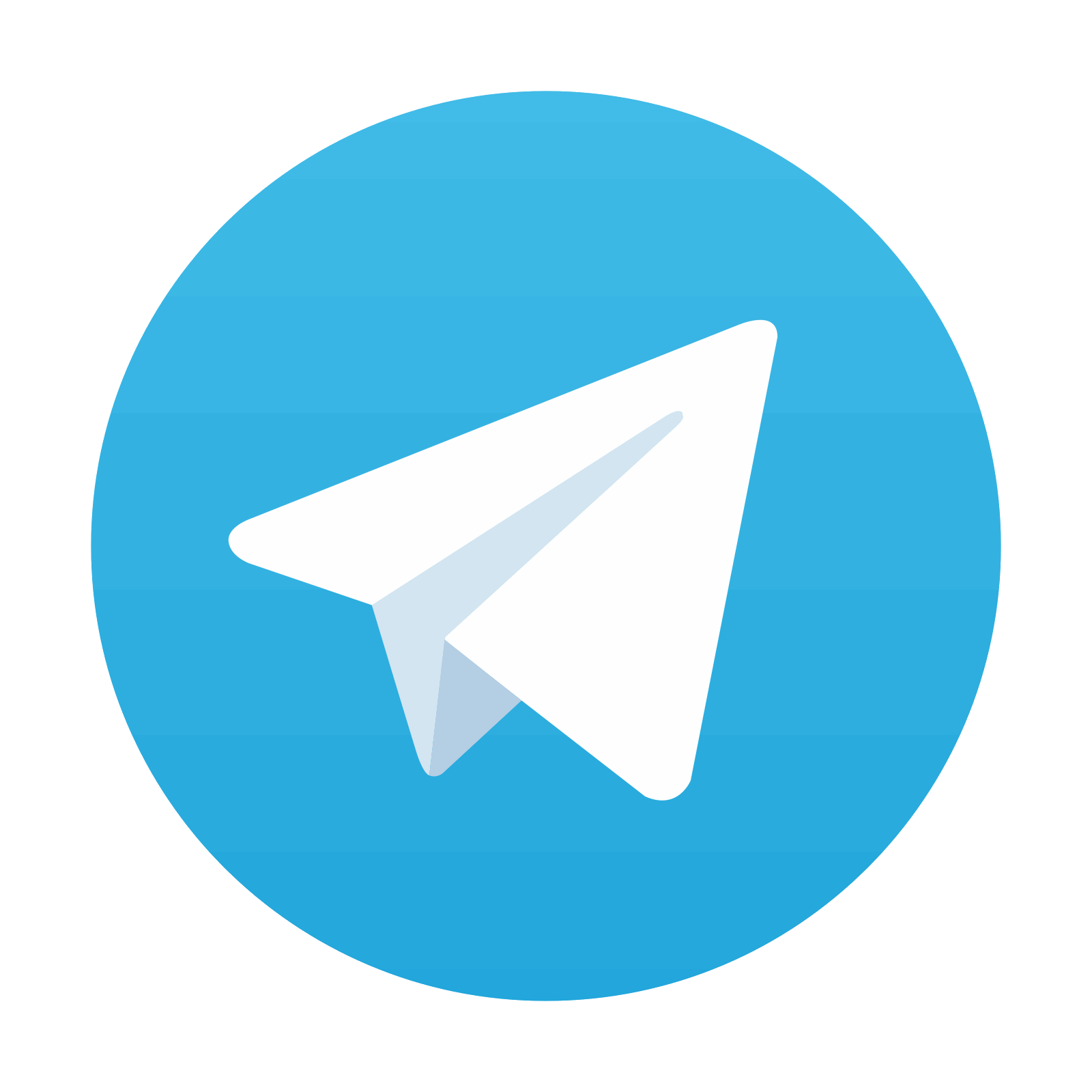
Stay updated, free articles. Join our Telegram channel

Full access? Get Clinical Tree
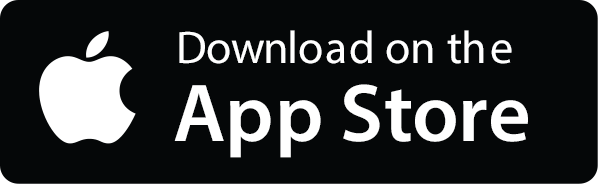
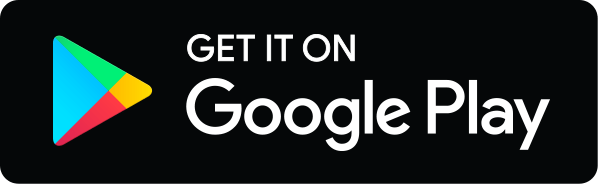