20
SPORTS NUTRITION
Nyree Dardarian, Brandy-Joe Milliron, and Debra Bateman
INTRODUCTION
Dietary behaviors are a crucial component of athletic training and competition. Consuming an optimal balance of energy, macronutrients, micronutrients, fluid, and electrolytes helps maximize athletic performance. However, among athletes, dietary requirements depend on factors such as the frequency, intensity, time, and type of physical activity. In addition to maximizing athletic performance, meeting dietary requirements is essential to minimize the risk of injury and promote repair—before, during, and after exercise. Substantial differences exist between female and male athletes and should be considered when encouraging athletes to meet dietary recommendations. Nutrition requirements for female and male athletes are not the same. Biological, social, and environmental factors influence sex differences in food intake, metabolism, and nutrient bioavailability. Therefore, the purpose of this chapter is to provide evidence-based nutrition recommendations for female and male athletes through a comprehensive overview of the role and recommendations for total energy requirements, carbohydrate, protein, fat, vitamins, minerals, and water.
• Females report greater nutrition-related awareness and knowledge when compared to males (1).
• Females report consuming more fruits, vegetables, whole grains, and dairy products than males (1).
• Female athletes are more likely to restrict energy intake, especially when competing in sports that accentuate body shape and size (2).
• Resting metabolic rate (RMR) is lower in females than males (3).
• There are sex differences in substrate utilization during exercise such that females oxidize more lipids and fewer carbohydrates (4–9).
• Skeletal muscle glycogen utilization and hepatic glucose production is lower in females than males during moderate and intense endurance exercise (10).
• Reported food intake for women is lowest during the periovulatory period of the menstrual cycle when plasma estradiol levels are high (11).
• Phillips et al. demonstrated females exhibit lower oxidation of leucine, a branched chain amino acid correlated to protein synthesis, during exercise compared to males (12,13).
• Overall protein requirements are lower for female than male athletes, 1.3 to 1.4 grams per kilogram of body mass per day (abbreviated as g/kg/d) and 1.7 g/kg/d, respectively (14).
SEX DIFFERENCES IN EATING BEHAVIOR
Males and females differ in their eating attitudes, dietary habits, and food choices as a result of biological and sociocultural factors. The most commonly reported difference between males and females is total energy intake. On average, males consume more energy than females due to their higher mean body mass, energy expenditure, and energy needs (11). In athletes, females are less likely to meet daily caloric requirements (15). Research suggests that males eat faster and take bigger bites of food and sips of beverages when compared to females. Furthermore, when food volume consumption is the same, males ingest more calories than females, suggesting that males consume more energy-dense food items (15). Similarly, male athletes eat more meals per day than females. Among endurance athletes, males report consuming as many as nine meals per day; however, because of the heightened energy needs from physical activity, male athletes can fall short of their total daily energy needs (16).
Females are at a greater risk of inadequate energy consumption. Several studies have reported suboptimal energy intakes for female athletes across a variety of sports (2,17–19). Even when comparing female and male athletes in the same sport, females report dietary intakes characterized by insufficient energy and macronutrient (20). Hoogenboom et al. evaluated the nutrient intakes of female collegiate swimmers and reported only 9.4% of female swimmers consumed 100% of their calculated energy needs (21). Inadequate calorie intake in female athletes is often coupled with inadequate calcium intakes. Low calcium consumption presents the risk of compromised bone health, low bone density, and osteoporosis later in life (18,22).
Regarding food choices, women report consuming more fruits and vegetables, cereals, and whole grains; males report consuming greater amounts of meat products (including nonlean meats such as pork, beef, and sausage), eggs, alcohol, high-sucrose foods, and high-starch foods. Consequently, females eat more fiber and nutrient-dense foods, and males eat more saturated fat and cholesterol (1,23). Both female and male athletes report insufficient carbohydrate consumption, excessive fat consumption, and sufficient or excess protein intakes. This often varies by type of activity or sport. For example, football and baseball players have reported higher fat intakes when compared to triathletes and marathon runners, whose total energy is primarily comprised of carbohydrates but is still too low to accommodate their high carbohydrate utilization (24).
Eating behaviors among female athletes can be largely explained by sociocultural influences. While female athletes report healthier food choices, eating disorders and disordered eating are also more commonly reported and have no clear biological basis (19,21). In fact, the sports recording the highest rates of eating disorders are lean (cross-country, swimming) and aesthetic (diving, gymnastics) sports (18,19). In a study comparing body image and performance goals between the sexes, males’ valued speed, power, and size while females esteemed low body fat above all else. The female athletes viewed leanness as a competitive advantage that could enhance performance and were more inclined to practice restrained eating, even if it meant falling well below their energy and macronutrient recommended dietary allowance (RDA) ranges (2). A similar study surveying males reported an average goal body weight that was 104% of their current weight. The researchers also reported that weight gain, as long as it was in the form of more muscle mass, was revered among 87% of the male athletes (25). Dieting, restricted eating, consuming fewer meals per day, and suffering from eating disorders may manifest from female athletes’ exposure to body image ideals, pressures to be thin, and media promotion of weight-restricting behaviors (21).
ENERGY
For athletes, adequate energy intake is the balance of energy expended and energy consumed to optimize athletic performance. Total daily energy expenditure is represented by four subcomponents: basal metabolic rate, thermic effect of food, nonenergy activity thermogenesis, and exercise energy thermogenesis. The terms kilocalorie (kcal) and calorie are often used interchangeably when describing energy derived from foods sources. The kilocalorie represents the amount of heat needed to raise 1 kilogram of water 1° Celsius. Calories consumed from foods and fluids provide the energy to maintain weight, replenish glycogen stores, and repair damaged tissue. Daily caloric needs depend on the frequency, intensity, time, and type of physical activity. Energy needs fluctuate in response to the duration and intensity of the physical activity, as well as the environmental conditions during training. Determining individualized energy requirements is a crucial component of the diet prescription. Inadequate energy intake can be detrimental to performance; it is associated with loss of muscle mass or inadequate promotion of muscle mass, is detrimental to bone density, and increases the risk of early fatigue, which can translate to increased risk for injury. In addition, inadequate energy intake among female athletes increases their risk for menstrual and endocrine dysfunction.
Energy Expenditure
For nonathletes, RMR is the largest component of total daily expenditure, representing nearly 75% of individuals energy needs over a 24-hour period. RMR accounts for the energy required to maintain the body’s cellular processes for life, such as heartbeat and respiration, while in an awake and postabsorbtive state. Basal Metabolic Rate (BMR) is often used interchangeably with RMR. Age, sex, body weight, hormones, and body composition influence an individual’s RMR. The RMR for adult females is approximately 10% to 15% lower than males due to a higher proportion of fat to muscle mass. Another difference between healthy weight females and males is the average decline in RMR per decade between the ages of 20 and 96 years, by 2% and 2.9%, respectively (3). The decrease in metabolic rate accelerates around the age of 40 years in males and 50 years in females (26,27). The loss of fat-free mass associated with menopause may explain the later and more accelerated decline in metabolic rate for females (26,27). Using 2,400 calories as the recommended energy requirement for the average healthy female or male, a 2% to 2.9% decline in energy requirements per decade would translate to a 48 to 72 daily calorie restriction. Though 48 to 72 calories seems trivial, the clinical significance is the compounding effects of the metabolic decline. A daily 50-calorie surplus in energy intake per day may result in a 5-pound weight gain each year. However, maintaining metabolically active muscle mass can hinder the decline in metabolism and is more frequently the case in athletes of both sexes. The thermic effect of food represents approximately 25% of total daily energy expenditure and is used to express the energy expended for digestion.
Physical activity, categorized as nonexercise activity thermogenesis and exercise energy expenditure, poses the greatest fluctuation when determining total daily energy expenditure. Energy requirements used for nonexercise-related activities of daily living vary depending on an individual’s lifestyle. Active individuals would expend more energy a day simply by sitting less and walking more than someone who is sedentary, thereby increasing their nonexercise activity thermogenesis. For athletes, exercise energy expenditure may result in an energy requirement greater than RMR, based on frequency, intensity, duration, and type. Exercise energy expenditure on any given day may range from zero calories for a nonexerciser up to several thousand calories for an athlete during training or competition.
Determining Energy Requirements
Several methods are used to determine energy needs. These methods include using stable isotopes (i.e., doubly labeled) or indirect calorimetry (i.e., whole room calorimeter or metabolic cart). The measurement of energy expenditure is costly, requiring expensive equipment and skilled staff. Therefore, several prediction equations have been developed to estimate RMR. Most of the predictive equations used to estimate energy needs incorporate height, sex, weight, and age as variables. Fat-free mass is included in the Cunningham equation, thus providing a more accurate estimation for athletes’ needs, though adding a barrier to its use in common practice since measuring body fat is required (28,29). The Harris-Benedict and Cunningham equations are the most commonly used in sports nutrition to estimate the RMR of athletes. Of the two, the Harris-Benedict equation is typically used to estimate BMR, then multiplied by an activity or injury factor to determine total daily energy expenditure (29).
Harris-Benedict Equation
Adult Male: BMR = 66.473 + (13.7516 × weight in kg) + (5.0033 × height in cm)−(6.7550 × age in years)(29)
Adult Female: BMR = 655.0955 + (9.5634 × weight in kg) + (1.8496 × height in cm)−(4.6756 × age in years) (29)
Activity Factors
1.2 | Little to no exercise |
1.37 | Light exercise |
1.55 | Moderate exercise |
1.725 | Heavy exercise |
1.9 | Very heavy exercise |
CARBOHYDRATES
Carbohydrates are one of the three macronutrients and a primary source of energy for the body and brain. In humans, carbohydrates are stored in the muscle and liver as glycogen, as well as a small amount of circulating blood glucose. Consumption of carbohydrates helps to maintain these stores, providing quick and accessible energy for everyday tasks. Carbohydrates are ultimately catabolized into glucose, the preferred source of energy for the brain, muscles, and central nervous system. Compared to nonathletes, athletes require more carbohydrates due to increased energy needs and utilization. Adequate carbohydrate consumption ensures optimal energy metabolism, immediate fuel during training and competition, and rapid post-workout muscle recovery. However, both male and female athletes consistently fall short of the recommended guidelines for carbohydrate intake (19,21,30). Between 45% and 65% of total calories should come from carbohydrates to prevent fatigue, increase muscle glycogen storage capacity, and optimize athletic performance.
Carbohydrates are comprised of multiple subcategories: monosaccharides, oligosaccharides, and polysaccharides. Monosaccharides and disaccharides are commonly referred to as simple carbohydrates. Monosaccharides (glucose, fructose, and galactose) are distinctively sweet compared to other carbohydrates. Disaccharides include lactose, maltose, and sucrose and consist of two monosaccharides. Oligosaccharides and polysaccharides are larger, complex carbohydrates that consist of three or more monosaccharides. Oligosaccharides and polysaccharides are commonly referred to as complex carbohydrates and include starch (amylose and amylopectin), glycogen (glucose storage in humans), and fiber. The difference between starch and fiber resides in their glucose linkages. In starch, monosaccharides are joined by alpha-linkages that are easily cleaved during the digestive process. However, monosaccharides in fiber are joined by strong beta glucose linkages and require specific enzymes or bacteria for digestion, which humans lack. Nondigestible fiber, such as cellulose, is associated with a number of health benefits, including the maintenance of bowel regularity, and should hold a secure portion of a healthy balanced diet (31–35).
Carbohydrate Metabolism During Exercise
The primary function of carbohydrates is to produce energy in the form of adenosine triphosphate (ATP) (36). ATP fuels endergonic cellular reactions that affect mechanical energy for muscle movement. It is also an important coenzyme required for body temperature regulation during exercise. All carbohydrates follow the glycolytic pathway, whereby energy is attained through aerobic and/or anaerobic respiration. The reactions from the oxidation of glucose in the glycolysis pathway and subsequent oxidation of pyruvate result in a total production of 36 ATP molecules (36).
During energy production, glucose is derived from either circulating blood glucose or glucose stored as glycogen. The average adult stores between 300 and 400 grams of glycogen in muscle (37). Males typically possess higher muscle-to-body weight ratios than females that results in a greater glycogen storage capacity, closer to 400 grams (6,9,38). Higher levels of muscle glycogen extend the time and amount of work that an athlete can perform before the body begins converting other substrates (36). Oxidation of alternative substrates can lead to muscle breakdown and adverse by-products such as lactic acid, therefore maintaining optimal glycogen capacity recommended for athletes.
Carbohydrate Loading
Athletes can augment glycogen stores through carbohydrate loading. Trained muscle is able to store more glycogen than untrained muscle (39). Consuming a carbohydrate-rich diet (50% to 75% of total calories) 3 to 4 days consecutively leading up to competition has been shown to increase both muscle glycogen stores per kilogram body weight and improve muscle stamina (9). This phenomenon is of particular interest to endurance athletes. For example, distance runners, who practice carbohydrate loading, may not run faster initially, but are able to sustain a faster pace throughout the duration of their race, resulting in a better overall performance (40–42) (Figure 20.1).
FIGURE 20.1: How to build a training plate. Carbohydrate needs increase when preparing for competition. Use this plate model to add more carbohydrate fuel to maximize glycogen storage.
Sex Differences in Carbohydrate Metabolism and Requirements
Recent research suggests that carbohydrate loading may not be as advantageous for females as compared to males. Among male athletes, carbohydrate loading is associated with better performance outcomes such as increased stamina and maximal oxygen (O2) consumption (4–6,43–45). Researchers hypothesize that the sex hormones estrogen and progesterone promote glycogen sparing during intense exercise such that fat is more commonly utilized as energy compared to carbohydrates. Similarly, females oxidize fewer carbohydrates during low- and moderate-intensity endurance exercise when compared to males. Estradiol, the most biologically active form of estrogen, is a female steroid hormone produced by the ovaries. Estradiol is involved in menstrual cycle regulation and ovulation and has been linked to energy metabolism. Animal studies have been conducted to investigate the effects of estradiol on glycogen metabolism. In animal studies, administration of estradiol to male rats was associated with increases in lipid availability and utilization during exercise, decreases in glycogen utilization, and increases in work output and exercise duration (46–49).
Female and male athletes use fuel sources differently during exercise that last longer than 90 minutes. Males oxidized more carbohydrates than females, and females metabolized more fat than males. Even when matched in terms of training, females use fewer carbohydrates than males. The higher content of intramuscular fat in women may account for the differences in metabolism. As with glycogen loading, hormonal differences in estradiol levels may also play a role (4,43,46,49–51).
Despite the reported differences in glycogen utilization and loading between females and males, the carbohydrate recommendations for both are determined by calculating total energy needs requirements (9). The minimum carbohydrate requirement for the average adult is 130 grams per day. More specifically, carbohydrates should contribute 50% to 55% of total caloric requirements. Sugar should comprise no more than 25% of those carbohydrates. Due to increased energy demand and output, athletes have slightly higher recommendations. Approximately 45% to 65% of total energy intake should consist of carbohydrates. In terms of dietary fiber, no differences have been established between active and nonactive individuals. Based on total energy needs, males are recommended to consume 38 grams of fiber per day, and females are recommended to consume 25 grams per day (52,53).
Carbohydrate needs can also be estimated using body weight in kilograms and activity level. Regardless of sex, athletes require between 5 and 10 grams of carbohydrates per kilogram of body mass per day (g/kg/d). Endurance athletes usually require between 7 and 10 g/kg/d while individuals engaged in low-intensity training may require no more than 5 g/kg/d (52,54).
FATS
Fat is our most highly concentrated energy source that fuels our bodies through low- and high-intensity work. Unlike carbohydrates and protein, which provide only 4 calories per gram, fat provides 9 calories per gram. Once glycogen stores are depleted, energy production is sustained through the metabolism of fat circulating in plasma and stored in muscle and adipose tissue. Fats are essential for the regulation of numerous bodily functions, including molecular signaling, cellular membrane structure, digestion, insulation, joint lubrication, and vitamin transport. In addition, fat adds flavor and texture to foods and provides sustained satiety after eating. Contrary to the common misconception that dietary fat intake and body fat are inversely associated with athletic performance, athletes need fat. However, some types of fat are more beneficial than others.
Types of Fat
Fats are distinguished as a group of compounds that are soluble in organic solvents of no or low polarity, but are not soluble in water. The nonpolar nature of fat is due to mostly carbon and hydrogen atoms comprising a large portion of the molecule. Typically this nonpolar region is a fatty acid. Lipids are commonly found in foods and the human body as triglycerides, phospholipids, and sterols. Triglycerides are the most common form of fat found in the diet (95%). Animal fat, butter, oil, and margarine are examples of triglycerides that we consume. Fats, such as those derived from animals, are soluble at room temperature whereas most oils, such as vegetable oil, are in the liquid state. Triglycerides are classified by their chain length, level of saturation, and molecular shape. Triglycerides, according to their chain length, are classified as short-chain fatty acids (usually fewer than 6 carbon atoms in length), medium-chain fatty acids (6 to 12 carbon atoms in length), or long-chain fatty acids (14 or more carbon atoms in length). Fatty acid chain length determines the method of digestion and absorption. Short- and medium-chain fatty acids are metabolized more quickly than longer fatty acid chains. The number of carbon-carbon double bonds determines the level of saturation. All carbons are bound to hydrogen such that the chains are saturated with hydrogen. Saturation results in a more stable structure with limited molecular mobility. Unsaturated fats (vegetable oils) have a double bond between carbon atoms, and polyunsaturated fats have several double bonds. Double bonds introduce kinks in the fatty acid chains, which prevent the chains from matching up and solidifying at room temperature. With the assistance of a catalyst, double bonds in unsaturated fats can be hydrogenated to solidify at room temperature. Naturally occurring cis-isomer arrangements are converted to trans-arrangements, resulting in trans saturated fats that behave more like saturated fats. Saturated and trans saturated fats are both associated with higher levels of low density lipoprotein (LDL) or “bad” cholesterol and lower levels of high density lipoprotein (HDL) or “good” cholesterol and should be consumed in moderation (55).
Omega-3 fatty acids have been researched for their potential gains in athletic performance. Several studies looking at omega-3 fatty acid have reported enhanced oxygen transport to the muscles and tissues, improved aerobic metabolism, decreased constriction of bronchial tubes (airways) during exercise, increased production of recovery-related hormones, and reduced inflammation of joints, muscles, and surrounding tissue (56–60). Linoleic acid, also known as omega-6 fatty acid, has a double bond at the sixth carbon on its fatty acid chain. Linolenic acid, or omega-3 fatty acid, has a double bond at the third carbon on its fatty acid chain. The recommended ratio of linoleic to linolenic acid for optimum health is between 1:1 and 5:1 (61).
Fat Metabolism
Contrary to carbohydrates, which can be metabolized both aerobically and anaerobically, fat can only be metabolized under aerobic conditions. During low-intensity exercise, plasma triglycerides are the preferred fuel source. As intensity increases, metabolic preference shifts to carbohydrates. As intensity and duration continue to rise, glycogen stores diminish and intramuscular fat oxidation becomes the primary source of fuel (6,43,62,63).
Protein-bound blood triglycerides and intramuscular triglycerides are metabolized for energy by the same beta-oxidation pathway in the mitochondria. Lipases hydrolyze lipids into component fatty acids and glycerol. Fatty acids are split per carbon pairs and metabolized to acetyl-Coenzyme A (acetyl-CoA) in the cycle. At each two-carbon interval, the abridged chain reenters the beta-oxidation cycle until it has been completely reduced to acetyl-CoA. Carbohydrates play a vital role in this metabolic pathway and are required for the complete oxidation of fats. At the termination of the beta-oxidation cycles, each fatty acid yields 14 molecules of ATP. The additional phosphorylation of glycerol delivers another 2.5 to 4.5 molecules of ATP. It is important to note that glycerol may also be used for other energy needs. For example, glycerol, which is metabolized like a carbohydrate, may be converted to sugar in the liver and used to regulate blood glucose when liver glycogen stores have been exhausted.
At approximately 60% to 65% of the body’s maximum oxygen consumption (VO2 max), fat oxidation is at its highest and most efficient. At a VO2 max higher than 65%, substantial fat oxidation is still taking place. Endurance training can lead to an increase in the body’s capacity to burn fat by increasing the size and quantity of mitochondria (location of beta-oxidation) and the number of metabolic enzymes involved in the pathway (63).
Dietary Fat Recommendations
Similar to dietary carbohydrate recommendations, female and male dietary fat recommendations are based on total energy intake, body weight, and activity level. Differences in fat oxidation are not incorporated into dietary fat recommendations. Research findings are mixed regarding the ideal fat consumption for athletes. Some evidence suggests that appropriate ranges are activity or sport specific. Studies of cyclists and triathletes report total fat intakes between 27% and 32% of total food energy (17,64). Research on female swimmers reports intakes as low as 22% energy from fat (65,66), while other studies report above 40% for female and male swimmers (22,67–69). Proximity to race day may also influence fat intake. Some studies report decreased fat intake leading up to competition, while others showed no change (24,70). Variability also exists among countries. One study reported that Croatian gymnasts consumed an average of 30% total energy from fat (18). Other studies have reported that Kenyan runners consumed only 13% total energy from fat (53,71). On average, fat in the diet should account for 20% to 35% of total calories; athletes may benefit from no more than 25% of total calories from fat (52,53).
Other recommendations based on body weight rather than caloric load suggest 1 gram of fat per kilogram of body weight per day for active individuals and low-intensity sports, and up to 2 grams per kilogram of body weight per day for endurance athletes (52,53). Athletes who have difficulty maintaining weight and/or consuming sufficient calories to meet energy output may require higher intakes of dietary fat. For health benefits, unsaturated fats from fruits, vegetables, nuts, oils, and cold-water fish are preferred to saturated and trans saturated fats from animal products or processed and fried foods. Increased fat utilization is believed to spare glycogen, which can result in stamina gains and increased performance. The “fat loading” theory suggests that the body adapts to the available fuel by burning fat more efficiently. However, when tested in a research setting, fat loading did not result in significant performance improvement and was shown less beneficial than conventional carbohydrate and fat intakes (72,73). Consumption of medium-chain triglycerides is another approach to augmenting performance. Medium-chain triglycerides are catabolized and absorbed more quickly when compared to long-chain fatty acids and are believed to deliver energy as fast as carbohydrates; however, results of research investigating the effects of medium-chain triglyceride consumption on performance are conflicting (74–76).
Glycerol, a three-carbon compound found in triglycerides and phospholipids, has been consistently shown to aid athletes competing in endurance activity performed in high temperatures (76–79). This is because glycerol is a humectant, meaning it can hold water. Consuming glycerol prior to competitions where excessive water loss is a risk allows the body to retain more water. This gives rise to a “hyperhydrated” state. Advantages were observed in studies assessing Olympic triathletes, mountain bikers, and tennis players subjected to hot environments who were hyperhydrated prior to competing (77–79). However, the World Anti-Doping Agency has banned the use of excess glycerol as an athletic aid.
Sex Differences in Fat Metabolism
Females naturally have a higher percentage of total body fat than men. The normal percent body fat range for a nonathlete female in her mid-20s is 25% to 31%, while that of an age-matched, nonathlete male is 18% to 25%. As an individual’s fitness increases, percent body fat decreases. The normal body fat range for active females and female athletes is 21% to 24% and 14% to 20%, respectively. The normal body fat range for active males and male athletes is 14% to 17% and 6% to 13%, respectively. Essential body fat, defined as body fat necessary for life and reproduction, is also higher for females to ensure appropriate body composition for childbearing and adequate hormone regulation. For all persons, athletes and nonactive individuals, essential body fat ranges from 10% to 12% in females and 2% to 4% in males (80,81).
Females exhibit unique metabolic responses to ingested and stored fat. Comparisons between equally conditioned males and females during the same endurance activity show proportionately higher fat oxidation rates in females. This may result from higher essential fat requirements and greater levels of intramuscular fat, which would suggest greater availability and accessibility to fat for energy conversion (4–6,43,44). Hormones are also believed to potentially influence this metabolic process. Estrogen’s active form, estradiol, is the hormone suspected of influencing fuel utilization. Estradiol has been linked to energy metabolism and greater fat-to-carbohydrate conversion in animal studies (46–49). There are no differences in the recommendations for dietary fat exist between males and females, despite these findings (53).
PROTEIN
Proteins play a fundamental role in athletic performance. They vary in structure and function. Connective tissues, tendons, certain organs, and muscle fibers are made of fibrous proteins. Fibrous proteins are insoluble in water and primarily serve a structural purpose in the body. Globular proteins are also found in animals and transport substances throughout the body. Unlike fibrous proteins, globular proteins are soluble in water. Hemoglobin is an example of a globular protein that serves as a transporter, carrying oxygen from the heart to the tissues. Enzymes are also globular proteins that facilitate metabolic reactions. Enzymes are often referred to as catalysts as they speed up biochemical reactions (82).
The functions of proteins are not limited to structure, transport, and catalysis. Proteins are also involved in movement (muscles), hormone synthesis (insulin), nutrient storage (ferritin), pH balance (amphoteric buffers), fluid and osmolarity homeostasis, immunity (antibodies), and regulation of various cellular responses (rhodopsin). While protein performs a variety of functions in the body, it does not make a significant contribution in energy production; protein contributes only 2% to 4% of total ATP (16,83,84).
Amino Acids
Amino acids are the building blocks of proteins whose order and properties largely determine a protein’s chemical behavior. Humans require both essential and nonessential amino acids. Essential amino acids must be consumed through the foods that we eat. Nonessential amino acids can be synthesized in the body.
Higher quality proteins have higher biological value, meaning the body absorbs a greater proportion of the ingested protein. A protein’s biological value is determined by the amino acids that it is comprised of; an appropriate combination of amino acids, including all of the essential amino acids, will be better absorbed and incorporated into the body. In deamination and transamination, discussed later in the chapter, proteins lose their nitrogen atoms for storage or through new protein formation. These processes will only occur if the protein cannot be synthesized by the body (nonessential) and is not in high demand. Comparison of a protein food to albumin from egg protein is a way to assess protein quality. Albumin is recognized for its complete distribution of essential amino acids. Egg-matched quality for whey protein is highest at 104/100, 100/100 for a whole egg, and 91/100 for cow’s milk. Beef and soy are slightly lower at 80/100 and 74/100 (8,85,86).
Protein Metabolism
Proteins are digested in descending order of structure: first polypeptides, then smaller peptides, and finally the original amino acid building blocks. Amino acids enter a pool for reconstruction into proteins as needed by the body. Hair, skin, nails, muscle, enzymes, and hormones all source from this pool. The liver is the main regulatory station for protein synthesis, maintaining bodily protein requirements through transamination and deamination. Transamination takes place when the nitrogen from one amino acid is cleaved and reused for the synthesis of another amino acid. In situations of protein excess, deamination occurs. Through deamination, amino groups are removed from proteins, leaving behind long skeleton chains of carbon, hydrogen, and oxygen. These carbon-rich structures can be converted to glucose for energy or may be stored as fat to later be metabolized for energy. The residual ammonia enters the urea cycle and is excreted. Excessively high protein intake yields a greater need for urea excretion, which can increase overall water loss. Consequently, athletes on high protein diets who sweat during exercise are at an increased risk for dehydration (8,12).
Muscle Building
Many athletes believe that protein is the sole dietary factor necessary for muscle growth. Strength-building and power-lifting athletes are especially notorious for overconsuming protein (87,88). Furthermore, common sources for their protein surplus includes powders and supplements. The standard amino acid supplement not only costs more than high-protein food alternatives, but also contains less than 15% the amount of high-quality amino acids in a 1-ounce serving of meat (89).
Protein intake only needs to be altered slightly for muscle-building purposes and is no more important than obtaining sufficient levels of other macronutrients for lean muscle mass gains. The daily recommendation per kilogram of lean muscle desired is an increase of only 1.2 to 1.7 grams of protein. Carbohydrate intake must also increase by 5 to 12 grams per kilogram. From a net energy perspective, carbohydrates make up a larger fraction of total energy volume than protein. It is necessary to accompany the increase in calories with an increase in resistance and strength-building exercises so that the added energy is not stored as fat (89,90).
Protein Timing
Consuming protein prior to physical activity and following physical activity in conjunction with carbohydrates have both been associated with enhanced absorption and improved muscle growth and repair. Intermittent extremes in protein intake have been linked to blunted muscle gains as compared with moderate consistent ingestion across the day (91–93). Optimal muscle synthesis peaks at a maximal dose of 20 grams at a time. This 20-gram maximal dose value has been established for both females and males, regardless of size and sport (94,95). Hence, it is recommended that athletes evenly distribute their protein consumption throughout the day for optimal utilization (6,7,92,93).
Sex Differences in Protein Metabolism and Requirements
Protein recommendations for sedentary individuals are between 12% and 15% total calories, or 0.8 gram per kilogram body weight per day; whereas protein recommendations for athletes are between 1.2 and 1.7 grams per kilogram body weight. Athletes need higher quantities of protein due to increased muscle breakdown and tissue repair, higher lean body mass and lower body fat, protein metabolism during endurance or high-intensity exercise, and a potential increase in urinary protein loss (89,90). Males need more protein than females, closer to 1.7 grams per kilogram of body weight, because of generally higher lean body mass ratios and greater amino acids oxidation during exercise, especially long-duration, high-intensity work. Research of top tier athletes suggests even higher protein requirements, as these athletes will oxidize higher amounts of amino acids due to increased bodily demands for energy (4,6,7,12,85).
Percentage intakes based on total energy assume that total energy intake is adequate. Athletes must be careful to consume enough calories to accommodate their activity levels. Most athletes do well in consuming sufficient amounts of protein (85). However, vegetarian athletes, young athletes who are growing and developing, and athletes with other types of restricted diets may be at risk for inadequate protein intakes (85).
FLUID AND ELECTROLYTES
Water
Just as a car cannot function without gas, humans cannot function without water. It is the single most important nutrient in our diets. Water makes up approximately 60% of our bodies, 70% of our brains, and 90% of our lungs (82). Its main functions include thermoregulation, digestion, nutrient and oxygen transport, waste elimination, and joint and tissue lubrication (82). Water also serves as a medium for a number of chemical reactions underlying these processes. Temperature regulation, oxygen and energy transfer, and the structural role of water in muscle tissue make this nutrient especially critical to athletic performance (96–98). One of the easiest and most beneficial practices athletes can enlist to optimize their performance is sustaining their hydration levels throughout exercise. Well-hydrated athletes benefit from a normal rise in heart rate, regulated core body temperature, improved cardiovascular endurance, and prolonged muscle glycogen usage (82).
Fluid Balance
Body fluid is tightly maintained within 0.2% of total body weight through systems that increase water retention and increase water loss (82). Osmoreceptors in the hypothalamus and baroreceptors that regulate blood vessel pressure stimulate the release of hormones and enzymes that control water volume. Sixty-five percent of our body water is intracellular, while 35% is outside of the cell (96). Sodium is the primary component in the plasma that dictates the balance between intracellular and extracellular fluid, and in turn influences the receptors and hormones responsible for fluid balance (89). During exercise lasting more than an hour or higher in intensity, more water is lost than sodium. Under conditions of dehydration, the electrolyte-water balance in the blood, or blood osmolality, rises. The hypothalamus detects this change and signals the pituitary gland to release an antidiuretic hormone. In response to the antidiuretic hormone, the kidneys retain water (99).
Humans lose fluid through their sweat, skin, urine, feces, apocrine glands (armpits and groin), and respiration. Individual variations in metabolism lead to different fluid loss rates for all of the aforementioned (82). Males have larger and more active apocrine glands than females, as well as more androgenic steroids, which work in conjunction to increase axillary fluid secretions in the armpit (100). Individual differences may also be amplified by sport-type and environmental conditions. Warm environment, prolonged exercise, and high-intensity training can increase fluid loss through sweat and urine (101–104). Multiple studies have reported that the majority of athletes training in warm environments fail to consume adequate fluid and electrolytes to offset their losses (105–107). Cold, dry, or high-altitude environments raise fluid loss through respiration. When dry air is inhaled, the lungs humidify it and small amounts of water vapor are lost during each exhalation. The combination of dry air and lower temperatures increases our respiratory rate, leading to increased fluid losses. Athletes exercising in cold or high-altitude environments, such as cross-country skiers, may need to take extra precautions to ensure sufficient fluid intake (108).
Thirst
Sensations of thirst change in response to plasma sodium concentrations. When dehydrated, plasma osmolality concentrations increase, stimulating the urge to drink. Conversely, a decrease in plasma osmolality, which could generate from overhydration, suppresses thirst. Despite this physiological response, accumulating evidence suggests that thirst may actually be an inaccurate gauge of fluid needs (101,108). Research by Volpe et al. found that among college athletes involved in various sports, as many as 60% initiated practice in a dehydrated state (109). Other studies report that underhydrated athletes fail to consume enough fluid during and after competition to offset their losses (105–107). Performing under a dehydrated state has been shown to cause an increased heart rate and suboptimal performance (110). However, consuming fluid within an hour of training or competition is associated with lower heart rates and attenuated core body temperatures (82). Therefore, it is essential for athletes and coaches to implement and maintain a rigid fluid-replacement plan to avoid the negative effects of dehydration and ensure optimal performance.
Thermoregulation
During physical exertion, muscles can generate up to 20 times the amount of heat when compared to muscles at rest (111). One of the unique properties of water is its exceptionally high capacity for heat absorption and therefore its ability to perform as a homeostatic regulator of temperature. Thermoregulation is maintained through two mechanisms of action. The first is vasodilation and constriction, which adjusts the amount of blood that reaches the surface of the skin. The second is through sweat. As the sweat evaporates it also helps to cool the skin, which then cools the blood and ultimately lowers core body temperature (89).
Electrolytes
Electrolytes are positively or negatively charged minerals that control the on-and-off state of nearly all brain and muscle cells. They go hand-in-hand with water due to their ability to carry an electrical current through water, since water alone is a poor conductor of electricity. Electrolytes most closely associated to athletic performance include sodium, chloride, potassium, calcium, and magnesium. Heavy sweating and increased urinary output can lead to appreciable electrolyte losses during and after exercise. Sodium and chloride are lost in the greatest concentrations through sweat; however, prolonged and intense exercise can lead to substantial potassium losses as well. Individual variation prevents the determination of the exact concentrations of electrolytes lost through sweat. While magnesium and calcium losses are very small in comparison to other electrolytes, dietary needs are still higher for both minerals to account for losses through sweat.
Potassium deficiency is often blamed as the underlying cause of muscle cramping during activity, however sodium is to blame (101,112). Potassium lost through sweat only represents a small fraction of total body potassium loss, whereas sodium lost through sweat can account for as much as 20% of total body sodium loss. A moderate to vigorous 2-hour workout can deplete as much as one fifth of total body sodium (101,112). Athletes who train several hours per day in hot environments or who sweat heavily have an increased risk for excessive sweat sodium loss (103,104,113). Drinking plain water replenishes fluid loss, but does not compensate for electrolyte losses. In fact, plain water can increase the risk of electrolyte imbalance by diluting the blood, increasing urinary fluid excretion, and decreasing the urge to drink. Electrolyte imbalance and low sodium pose more threat than just cramping (112,114). Hyponatremia, a condition marked by dangerously low blood sodium, can lead to nausea, vomiting, cardiac and neurological abnormalities, and even death. A higher incidence of hyponatremia has been reported in female when compared to male athletes, most likely due to lower total body water volume (115–117) (Figure 20.2).
The ingestion of salt and salty foods is biologically driven through cravings following a workout and excessive sweat loss, minimizing the risk for developing hyponatremia (118). However, much like thirst, salt cravings may not manifest into significantly higher consumption of those minerals. Another biological mechanism to protect an athlete from hyponatremia is increased hunger resulting in greater total energy consumption. A high-calorie, well-balanced diet naturally includes higher levels of vitamins and minerals. Consequently, many athletes eating to meet their energy needs instinctively meet their increased mineral requirements. Other ways in which mineral needs can be easily met is by the consumption of sports drinks during and after workouts. Unlike water, sports drinks include the electrolytes lost in greatest concentrations through sweat. Though often avoided for their higher sugar content, sports drinks contain carbohydrates for the restoration and sparing of muscle glycogen, improved absorption, and increased palatability to provoke drinking (119–123). Consequently, sports drinks are a good alternative to plain water to foster fluid and electrolyte replenishment during and after practice and competition (106).
FIGURE 20.2: Urine color chart. The urine color chart uses urine color as an indicator and ongoing monitor of hydration status.
Assessing Fluid and Electrolyte Needs
Because so many variables contribute to individual fluid and electrolyte needs, a personalized approach is necessary to determine the appropriate dietary requirements for each individual athlete (101). Examples of individual discrepancies include heavy and salty perspirers who exhibit extraordinarily higher fluid and electrolyte losses relative to their teammates when engaged in the same activity (112–114). Beyond mere sweat observation, there are several ways that athletes, coaches, and practitioners can gauge fluid and electrolyte losses and needs. Assessing urine output and concentration is one of the easiest, cheapest, and simplest ways to determine hydration status. Maintaining a light yellow urine color indicates adequate hydration. Low urinary output and dark brownish-colored urine denotes dehydration (112–114). Nearly clear urine and heavy output suggests fluid intake in excess of need/loss. Another affordable means of assessing hydration status observes skin turgor. When skin on the back of the hand is pulled up and released and does not return to its original state, this may signal dehydration. Keep in mind that skin turgor is not a conclusive test of hydration, but rather one physical parameter that should be used in conjunction with other assessment methods. A more involved and reliable way to determine fluid needs is by weighing oneself before and after training or competition. The difference in body mass represents the total water lost, taking into account fluid consumed during training (124,125). Specific gravity of urine and plasma and/or urinary osmolality are two other methods to establish hydration status. These methods are less common in sports settings and often limited to labs due to higher costs and the need of equipment.
Fluid and Electrolyte Requirements
When fluid loss information is available from pre- and post-workout weigh-ins or other methods, athlete-specific formulas can be employed. Formerly, experts advised consuming 100% of water weight lost in pounds after a workout. However, subsequent research pointed out that a 1:1 ratio does not account for increases in drinking-related urinary output. For complete rehydration, it is now encourage to consume 150% of total water lost after exercise (124,125). The American Dietetic Association, Dietitians of Canada, and the American College of Sports Medicine published a comprehensive position statement on fluid and electrolyte replacement before, during, and after exercise. Their recommendations include 5 to 7 mL of water or sports drink per kilogram of body weight at least 4 hours before activity. During exercises, these organizations suggest adherence to an individual hydration program that prevents total body water loss above 2% of total body weight. Following exercise, at least 16 to 24 ounces of fluid should be consumed for every pound of body weight lost. Note that the minimum requirement equates 16 ounces consumed to 1 pound of fluid lost. And finally, in terms of electrolytes balance, the benefits of sports drinks and salty foods are accredited (85,126).
Fluid and Electrolyte Timing
One of the important pillars of the American Dietetic Association, Dietitians of Canada, and American College of Sports Medicine’s publication is the emphases on fluid and electrolyte timing. The timing of intake can have an impressive impact on performance. The experts advised consuming 5 to 7 mL of fluid per kilogram of body weight, which translates to about 1 ounce of fluid per 10 pounds of body weight, approximately 4 hours prior to competition (85,126). Why 4 hours? The rationale behind this time frame is that 4 hours allows for adequate time to consume more fluid if necessary or to excrete excess prior to work (127). Within an hour preceding training, athletes may still gain advantages from drinking, including lower heart rates and decreased core body temperatures. Replacing fluid and electrolytes during exercise has also shown positive effects on temperature and heart rate. Post-workout fluid and electrolyte replenishment is arguably the most critical to hasten the body’s recovery (82).
Sex Differences in Fluid and Electrolyte Balance
Our bodies range between 45% and 75% water, with 60% as the mean marker for both males and females. Because muscle contains roughly 75% more water than adipose tissue, fat is inversely correlated with total body water. Males average approximately 10% higher in body water percentage due to a generally higher proportion of lean muscle mass and lower total body fat relative to females. Females typically exhibit around 50% body water and males fall around 60%. Obese individuals may have as little as 40% body water due to high fat mass, and sometimes even less depending on the severity of their condition. Athletes, who have a higher proportion of lean muscle, may have as much as 70% total body water (89,101).
General rules of thumb for fluid requirements have been tailored throughout the years. Initially consuming half of one’s body weight in ounces was the general recommendation and is still the common acceptable practice used today. Because males typically weigh more than females, their fluid needs are higher. A sort of innate compensation, similar to that described with dietary mineral intake, accounts for males’ increased fluid needs. Males tend to consume more energy in their diets, and at least 20% of our fluid comes from the food we eat, so men naturally consume more fluid than females (101).
MICRONUTRIENTS AND SPORT
Micronutrients are vitamins and minerals that humans require in minute quantities and that play key roles in energy metabolism and muscle function. Humans need to consume a wide range of vitamins and minerals from our diets in order to satisfy the needs of our bodies and prevent the adverse effects that result from deficiencies. Vitamins are carbon-containing molecules that may be water- or fat-soluble. The primary purpose of vitamins in physical activity is the conversion of the three macronutrients into usable fuel for the body. In addition to energy metabolism, vitamins also help in red blood cell synthesis, immune health, skeletal integrity, and protection against oxidative damage (128).
Minerals are inorganic nutrients in simple elemental form. They are often consumed and found in trace amounts in the body. While miniscule in concentration, minerals serve a generous set of services in the body. Calcium is the most abundant mineral in the body that, along with phosphorus and magnesium, contribute to bone structure and integrity. Iron is the central atom in hemoglobin. Sodium and chloride are crucial to nerve and muscle stimulation. Magnesium is involved in over 300 metabolic reactions. Copper is the main element involved in the respiratory electron transport chain. Zinc, like magnesium, is a cofactor in many enzymatic reactions and aids in tissue repair (128).
Exercise impacts the turnover of micronutrients, increasing demands on the metabolic pathways in which these nutrients are required. Higher energy and oxygen consumption also raises oxidative stress, which may increase antioxidant-vitamin needs (129). While in theory athletes should need more micronutrients due to the increased utilization and demand of exercise, there are no athlete-specific recommendations in place that differ from the dietary guideline ranges for the general population. It can be safely assumed that due to increased metabolic processes and macronutrient needs, athletes require slightly higher levels of micronutrients. Nonetheless, there may be an inherent recompense at play: Athletes consume micronutrients in excess of standardized ranges due to higher total energy intake. Increased food consumption, given a balanced diet, results in inevitably higher vitamin and mineral intake. This does not insulate all athletes from the risk of deficiencies. Athletes with restricted eating habits and endurance competitors are susceptible to vitamin and mineral shortages, which have been associated with poor performance and compromised physical health and well-being (130). The literature recognizes vitamins A, C, E, D, and the major B vitamins, electrolyte minerals, and iron, copper, and zinc, for their supporting role in athletic performance. In this section, we review each of these vitamins and minerals with respect to athletic performance as well as their specific sex needs.
Vitamins
It is not unusual for athletes to mistake vitamins as sources of energy. In fact, one study found that 65% of athletes believed that vitamin and mineral supplementation increased their total available energy (84). While these nutrients are devoid of energy units, the athletes were not entirely wrong to associate energy with micronutrients.
B Vitamins
Many vitamins are involved in mitochondrial energy metabolism. Those best known for their energy-aiding effects are the B vitamins. Each of the B vitamins has a unique mission in energy metabolism. Thiamin’s main metabolic role is in carbohydrate metabolism, riboflavin partakes in the electron transport chain, niacin contributes to several metabolic pathways as nicotinamide adenenine dinucleotide (NAD) or nicotanimide adenine dinucleotide phosphate (NADP), B6 contributes to muscle growth and repair via amino acid manufacturing, and folic acid and B12 are vital to red blood cell synthesis. Deficiencies in B vitamins often result in fatigue, decreased endurance, and even anemia (130). Nowadays, B-vitamin deficiency is rare thanks to an increase in fortified foods. The RDA values for adolescents is between 0.9 and 2.0 mcg per day, and 2.4 mcg per day for 14- to 18-year-olds and adults. Pregnant and lactating women have slightly higher needs, at 2.6 mcg/day and 2.8 mcg/day, respectively (131). Animal proteins and fortified foods such as cereal, nuts, lentils, and some dairy products are good sources of B vitamins.
Vitamin D
Vitamin D is one of the most common vitamin deficiencies in the United States. We derive vitamin D from ultraviolet exposure, fatty fish, dairy, and fortified soymilk and orange juice. Evidenced by that list, dietary access to vitamin D is limited, while the functions of vitamin D are not. Calcium absorption and bone development, mineralization, and integrity rely on adequate levels of vitamin D. Insufficient vitamin D levels have been connected to increased incidence of fractures in athletes and depressed mood states (132–134). Even with above-average energy intakes, adequate vitamin D levels may still be a concern. Athletes at a higher risk for deficiency include those who compete in indoor sports (e.g., gymnastics, wrestling) or live in northern regions where outdoor exposure may be limited (135). The RDA for both male and female athletes is approximately 600 IU per day (131). Athletes lacking sufficient serum vitamin D levels may want to supplement with 200 IU to boost plasma concentrations (Table 20.1).
Antioxidants
The antioxidant nutrients beta-carotene, vitamin C, and vitamin E act as protective effects against free radical damage. Antioxidant activity is of particular importance in active individuals who have increased oxygen consumption. The elevated oxygen consumption exposes the muscles to a higher degree of oxidative stress. Elevated levels of reactive oxygen species in athletes have been associated with muscular and skeletal dysfunction leading to fatigue (129). Antioxidants are also affiliated with the maintenance of adequate immune function, which may be compromised by the added stress of exercise. In addition to its role against oxidative damage, vitamin C is implicated in collagen synthesis related to joint and muscle fitness and tissue repair. The RDAs for vitamin C are slightly different between males and females, at 75 mg per day (mg/d) for women and 90 mg/d for males. Different RDA values are also established for vitamin A. Females should consume 700 mcg of vitamin A per day, and males require around 900 mcg per day. The intake variations between sexes for these two vitamins are attributable to generalized differences in body mass and metabolic utilization. Vitamin E has an RDA value of 70 mg/d for both males and females (131). Deficiencies in these nutrients are rare and slow to develop. Symptoms may include joint pain and abnormal bone development with insufficient vitamin C. Poor ocular health arises without adequate vitamin A. Cell membrane degeneration in erythrocyte, neuronal, and muscle cells occurs if vitamin E is missing (128,130).
TABLE 20.1: Food Sources of Vitamin D
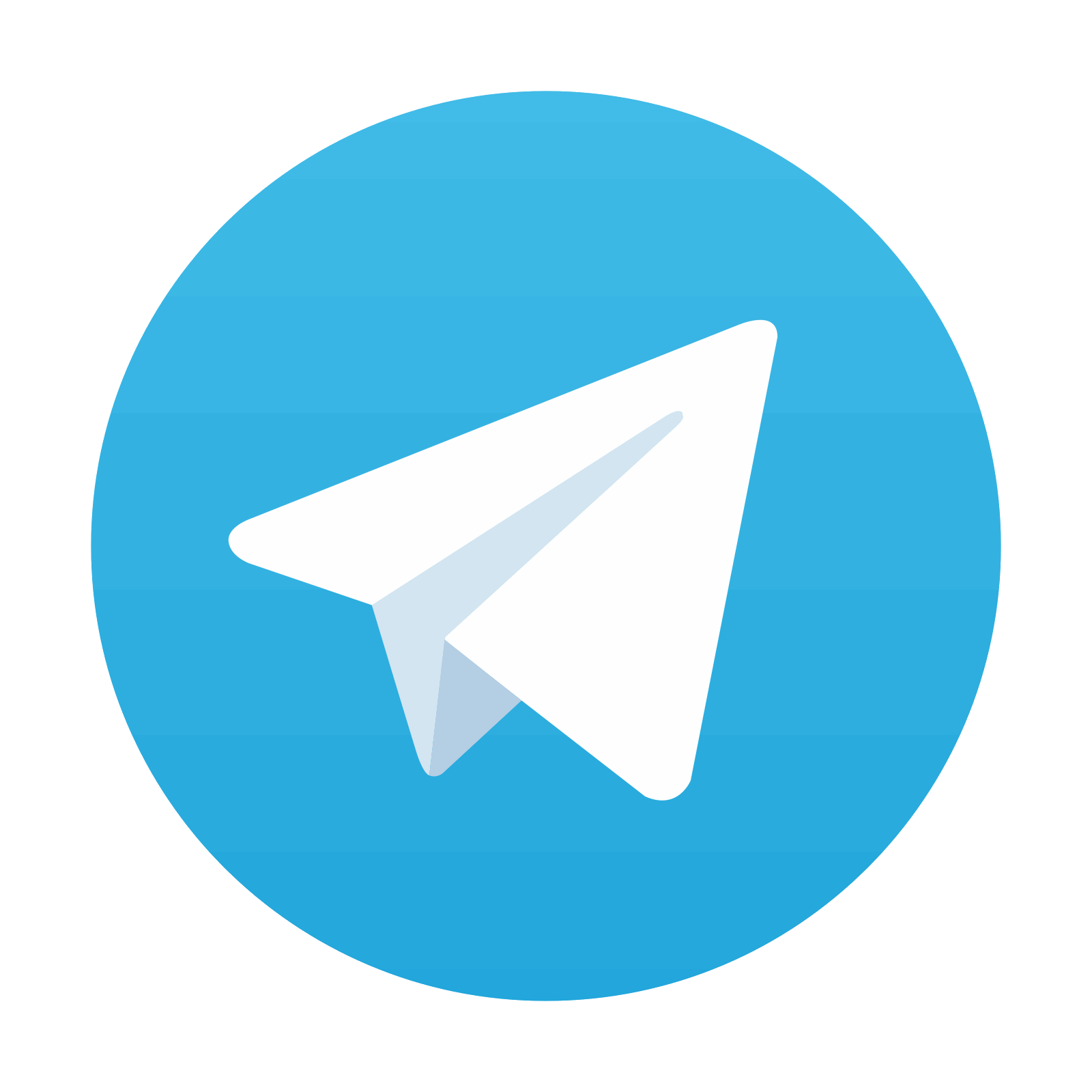
Stay updated, free articles. Join our Telegram channel

Full access? Get Clinical Tree
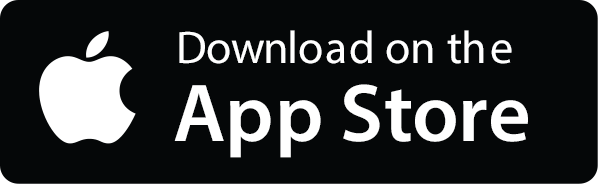
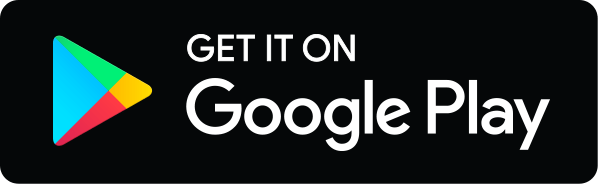