Chapter 55 Spinal Cord Injury
Historical Perspective
The Edwin Smith Surgical Papyrus, written by an Egyptian physician almost 5000 years ago, vividly describes the symptoms of neurologically complete injury to the cervical spinal cord—that is, paralysis and sensory loss in the arms and legs, urinary incontinence, and priapism.79,230 Later, in ancient Greece, approximately 400 BC, Hippocrates described paraplegia caused by injury or disease as being associated with paralysis, bladder and bowel dysfunction, and pressure ulcers.3 Several ancient Roman physicians also briefly described SCI, which they invariably considered to be fatal if neurologically complete, but if incomplete, reduction of spinal deformities by traction was recommended, a practice that was continued through the Middle Ages.160
During the nineteenth century, treatment of SCI continued to be conservative and without much hope for survival. A famous anecdote reflects well the prevailing attitude in those days. In 1805, Lord Nelson, the Admiral of the British Fleet, received a gunshot wound to his thoracic spine during the battle of Trafalgar, causing paraplegia. Nelson spoke with his ship’s surgeon, Mr. Beatty, and described his loss of power of motion and feeling below the chest, and then expressed his view that he would have but a short time to live. The surgeon’s reply was, “My lord, unhappily for our Country, nothing can be done for you.”215 Within a few hours Lord Nelson was dead. In 1881 the twentieth president of the United States, James A. Garfield, was shot in the spine, causing a neurologically incomplete conus–cauda equina lesion, but even with such a lesion he was dead within 3 months.161 Despite remarkable progress in medicine and surgery during the nineteenth century—for example, Pasteur’s discoveries in bacteriology, Lister’s aseptic surgical techniques, the introduction of anesthesia, and Roentgen’s discovery of x-rays—surgical interventions for spine trauma and SCI were generally discouraged.
During the early part of the twentieth century, there was little progress made in the management of SCI, and most persons with SCI died within weeks or months. Harvey Cushing observed that during World War I, 80% of all U.S. soldiers with SCI died within 2 weeks.215 Early mortality was slightly lower in the British military, but the 3-year mortality was estimated to be 80%.215 During the 1930s and 1940s, management of SCI finally started to change. During the late 1930s, Dr. Donald Munro323–325 at Boston City Hospital developed a dedicated unit for comprehensive care of persons with SCI, and by 1943, he was able to demonstrate significant drops in both morbidity and mortality, primarily by focusing on better bladder management. A few years later, in Great Britain during World War II, it was decided to congregate all casualties with SCI in special units that were supervised by an experienced physician. These units were to be sufficiently staffed by nurses and therapists, housed in facilities with rehabilitation workshops, and organized to provide resettlement and aftercare services.215 Dr. Ludwig Guttmann was placed in charge of such a unit at Stoke Mandeville, where he introduced comprehensive care and interdisciplinary rehabilitation for persons with SCI, a program that was widely modeled around the world. Within the U.S. Veterans Affairs Hospitals, Drs. Comarr and Bors introduced new methods of urologic management and rehabilitation, which quickly improved survival rates among U.S. veterans.
Model Systems of Care
Based on this hypothesis, the U.S. government funded several SCI Model Systems of Care, first in Phoenix, Arizona, in 1970, and subsequently in several other major cities around the United States. By 1982 there were 17 such systems funded by the National Institute on Disability and Rehabilitation Research (NIDRR), U.S. Department of Education. Each funded system had to meet four basic requirements. First, it had to have several integrated clinical components: emergency medical services; level 1 trauma center; comprehensive rehabilitation services for both inpatients and outpatients, including vocational and job placement services; and lifelong follow-up and health maintenance programs. Second, each funded system had to collect data on all patients served and forward these to a National SCI Model System Database. Third, each system was required to conduct research consistent with NIDRR-announced priorities. Fourth, each system had to disseminate the research and demonstration findings as widely as possible to the appropriate audiences.
The SCI Model Systems have been instrumental in developing standards of care and new treatments, and conducting epidemiologic, health services, and outcomes research, as well as producing thousands of publications and training materials.133 The National SCI Database has been in existence since 1973, and captures data from an estimated 13% of new SCI cases in the United States. The SCI Model Systems have been directly and indirectly instrumental in a number of positive developments for persons with SCI. These include increased survival rates, reduced hospital lengths of stay, and a reduced number of rehospitalizations. In addition, the SCI Model Systems have led to the majority of persons with SCI being discharged home, and created a national database of approximately 30,000 persons with SCI.428,429,437
Subspecialty of Spinal Cord Injury Medicine
Most physicians providing nonsurgical care for people with SCI in the United States have been physiatrists. In the past, most such physicians developed their special knowledge over a lengthy period by providing care rather than by specific training. A creation of a subspecialty of SCI medicine was first advocated in the late 1970s and gained momentum in the early 1990s. Through the concerted efforts of many individuals and organizations, the American Board of Medical Specialties gave its approval in 1995 that such a subspecialty be established.127,128 Based on the work experience and training of candidates that is acceptable to the American Board of Physical Medicine and Rehabilitation, candidates can take a written examination. Those who pass receive a special SCI medicine subspecialty certificate. Several 1-year SCI fellowships have been established, each providing a structured training program approved by the Accreditation Council for Graduate Medical Education. Several hundred individuals have been certified in the subspecialty since the first examination was held in 1999.
Epidemiology
Numerous epidemiologic studies have been reported since the 1970s in various countries, and these reflect some variance in the incidence and prevalence of SCI. Data have been collected in the United States by the SCI Model Systems for its database since the early 1970s. The analysis of these data by the National Spinal Cord Injury Statistical Center (NSCISC) has provided extensive and reliable information. The epidemiologic data have been widely published, and current information can be easily accessed on the NSCISC website, which is updated annually.19,132,133
Incidence and Prevalence
The annual incidence of traumatic SCI requiring hospitalization in the United States is approximately 40 new cases per million population (or approximately 12,000 per year). These numbers are the most up to date available, but are based on data collected before 1980. These numbers do not include an unknown number of individuals with SCI who died before reaching a hospital.70,335 The incidence of traumatic SCI in other developed countries has been shown to be somewhat lower than that in the United States, often less than 20 new cases per million per year, perhaps partly because of the higher U.S. incidence of violence-related SCI.132,133
The exact number of persons with SCI currently alive in the United States (prevalence) is a matter of dispute. Two methods have been used to estimate the prevalence of SCI: mathematic calculation based on annual incidence and average duration, and counting the exact number of people with SCI within a geographic area and extrapolating this number to the population of the entire United States. With the use of the mathematic approach, the prevalence of SCI in the United States has been estimated to be 259,000 persons, but a recent population study sponsored by the Christopher and Dana Reeve Foundation concluded after surveying more than 33,000 U.S. households that nearly 1.3 million people in the United States live with paralysis caused by SCI.23,89
Age at Time of Injury, Gender, Ethnicity, and Marital Status
Almost all studies show that the incidence of SCI is lowest for persons younger than 15 years and highest for persons 16 to 30 years of age. After the age of 30, there is a consistent decline in incidence. The current average age at onset is reported to be 40.2 years.23 A rising percentage of older persons with new SCI has recently been observed. For example, those older than 60 years constituted 4.5% of all new patients with SCI in the mid 1970s, versus 11.5% in the mid 1990s.335 A simultaneous rise in the median age of persons with SCI also occurred from the mid 1970s to the 1990s, with a rise from 28.5 years to 35.9 years. This change could reflect the improved medical care for persons with SCI, as well as the rise in the median age of the U.S. general population from 28 years to 35 years during the same period.133
More than 80% of all SCI occurs in males, a figure that has remained essentially constant for more than 30 years in the United States.132,133,335 This gender difference is similar in other countries. Approximately two thirds of all persons enrolled in the National SCI Database are white, which is significantly less than the percentage of whites among the general U.S. population.335
At the time of SCI, 30.4% of individuals had intact marriages, 53% had never been married, and the remaining 26.6% were separated, divorced, or widowed.132 The unmarried rate is a relatively high figure, perhaps best explained by the fact that SCI disproportionately affects young people.23,132 For those married at the time of SCI, the divorce rate is increased after SCI, as compared with that in the general population, especially during the first 3 postinjury years. The annual marriage rate is also lower for single individuals with SCI than for nondisabled persons.132
Causes of Spinal Cord Injury
The most common causes of SCI in descending order of incidence are vehicular crashes (42.1%), falls (26.7%), violence (15.1%), and sports (7.6%).19 In recent years, there has been a gradual decline in SCIs related to vehicular crashes and sports, whereas those relating to falls have increased. The causes of SCI vary significantly between groups of different ethnicity, age, gender, and geography. For example, falls are the most common cause of SCI among the elderly and violence among African Americans, whereas women rarely sustain SCI as a result of gunshots, motorcycle crashes, or diving. Considering the causes of SCI, it is not surprising that relatively more injuries occur on weekends and during the summertime.
Neurologic Level and Extent of Neurologic Deficit
According to the National SCI Database, tetraplegia is more common than paraplegia (50.5% vs. 44.1%). These are subdivided into the following neurologic categories: incomplete tetraplegia (30.1%), complete tetraplegia (20.4%), complete paraplegia (25.6%), and incomplete paraplegia (18.5%). Recent trends show an increase in incomplete tetraplegia and a slight reduction in complete paraplegia.23
Length of Stay, Rehospitalization, and Discharge Destination
The average length of stay for patients with SCI has declined dramatically over the years, according to the National SCI Database. This is true for both acute and rehabilitation hospitalizations, from 25 acute days in 1974 to 12 days in 2008, and from 115 rehabilitation days to 37 days.23 The number of days hospitalized is greater for persons with complete SCI compared with those with incomplete SCI. The number of rehospitalizations during the first year has steadily decreased as well.175 Among persons listed in the National SCI Database and discharged alive, 88.3% went to a private noninstitutional residence, 5% went to group living situations, 5.1% went to nursing homes, and the remaining 1.6% went to acute hospitals. Approximately 3% died during the initial hospitalization.132
Life Expectancy and Causes of Death
Life expectancy for persons with SCI has increased steadily for many decades but still remains below that of able-bodied individuals. The mortality rate is highest during the first postinjury year, at 6.3%, but declines significantly thereafter.132,140 The first-year mortality rate declined by 67% from the 1970s to the 1990s.132,138 Significant predictors of mortality include being older, male, injured by acts of violence, neurologically complete, and ventilator dependent, and having a high neurologic level.138 Additional factors that affect longevity after the first postinjury year include low life satisfaction, poor health, emotional distress, functional dependency, and poor adjustment to disability.256 The NSCISC website provides annual updates on life expectancy after the onset of SCI, based on neurologic level and ventilatory dependency (Table 55-1). These life expectancy estimates do not include many important variables that can also significantly affect survival, such as gender, ethnicity, preexisting medical conditions, access to medical and nursing care, and social support.
Table 55-1 Life Expectancy (Years) for Persons With Spinal Cord Injury Surviving at Least 1 Year Postinjury
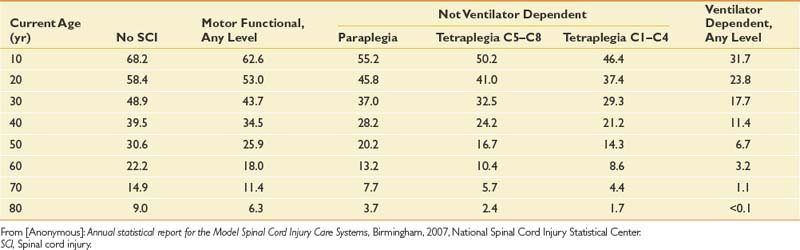
Diseases of the respiratory system, especially pneumonia, are the leading cause of death both during the first postinjury year and during subsequent years (Table 55-2). The second most common cause of death, “other heart disease,” is thought to reflect deaths that are apparently caused by heart attacks in younger persons without apparent underlying heart or vascular disease and cardiac dysrhythmia.132 Interestingly, diseases of the genitourinary system are currently the cause of death in only 3.7% of patients with SCI. In the past, renal failure was by far the leading cause of death after SCI. This is truly a great testament to the advances in urologic management during the past several decades.
Table 55-2 Primary Causes of Death
Primary Cause of Death | Percentage |
---|---|
Diseases of the respiratory system (70% pneumonia) | 22 |
Other heart disease (likely overreported representing poor quality of cause-of-death data after spinal cord injury) | 12 |
Infective and parasitic diseases (94% septicemia usually associated with pressure ulcers or urinary tract or respiratory tract infections) | 10 |
Hypertensive and ischemic heart disease | 8 |
Neoplasms | 7 |
Diseases of pulmonary circulation (96% pulmonary emboli) | 5 |
Diseases of the genitourinary system | 4 |
Suicides | 4 |
Other causes | 28 |
From [Anonymous]: Annual statistical report for the Model Spinal Cord Injury Care Systems, Birmingham, 2007, National Spinal Cord Injury Statistical Center.
Anatomy, Mechanics, and Syndromes of Traumatic Injury
Because the bony vertebral column elongates more than the spinal cord during embryologic development, the spinal cord terminates at the level of the L1–L2 intervertebral disk. Due to natural variation, the spinal cord termination can be as high as the T12 or as low as the L3 vertebral body. The individual spinal cord segments do not line up with the corresponding bony levels of the same number (Figure 55-1). This is especially evident in the lower thoracic and lumbar spine, where the L1–L5 spinal segments are adjacent to the T11–T12 vertebrae, and the S1–S5 spinal segments are adjacent to the L1 vertebra. This concept can also be used when evaluating radiologic studies to correlate the neurologic level of injury (NLI) to the appropriate bony level of damage (e.g., a T11 burst fracture with cord compression would be expected to cause an NLI at L1 or L2 rather than at T11).
The tapered end of the spinal cord, which contains the sacral cord segments, is called the conus medullaris. The collection of long lumbar and sacral roots found in the canal, distal to the conus medullaris, is called the cauda equina, because it resembles a horse’s tail. The meninges of the spinal cord include the pia matter, a vascular membrane covering the spinal cord, the arachnoid membrane, and the dura mater. The subarachnoid space, also called the intrathecal space, contains cerebrospinal fluid (CSF). The CSF pushes the arachnoid directly against the dura mater. The caudal margin of the dura mater and arachnoid, the inferior extent of the intrathecal space, is the second sacral vertebrae (Figure 55-2). The spinal epidural space is located between the dura mater and the periosteum of the vertebral bodies, and contains an internal vertebral venous plexus, fat, and loose areolar tissue.
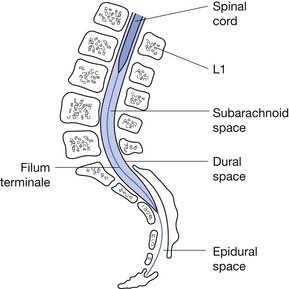
FIGURE 55-2 A sagittal schematic showing the relationship between the dura, subarachnoid space, and the epidural space.
(Redrawn from Pansky B: Review of gross anatomy, ed 5, New York, 1984, Macmillan, with permission of Macmillan.)
A cross-sectional view of the spinal cord (Figure 55-3) reveals a central butterfly-shaped region of gray matter consisting of neuronal cell bodies, their processes, supporting glial cells, and small blood vessels surrounded by white matter consisting of neuronal fiber tracts and supporting glial cells. The gray matter is subdivided into two horns on each side called the anterior (ventral) and posterior (dorsal) horns. The posterior horn contains cell bodies of sensory neurons, whereas the anterior horn contains cell bodies of interneurons and motor neurons.
The white matter is subdivided into three columns on each side called the anterior, lateral, and posterior columns. The columns are further subdivided into tracts. The gracilis tract, located in the medial posterior column, contains fibers from the T7–S5 dermatomes that relay touch, vibration, and position sense. The cuneatus tract, located in the lateral posterior column rostral to T6, contains fibers from dermatomes above T7 that relay touch, vibration, and position sense. These tracts, comprising the posterior columns, ascend ipsilaterally to the medulla. The lateral spinothalamic tract, located peripherally in the lateral column, contains fibers that relay pain and temperature sensations; this tract ascends contralaterally to the thalamus. The lateral corticospinal tract is located centrally and posteriorly in the lateral column. This tract contains fibers, most of which emanate from the motor cortex, that are responsible for voluntary and reflex movement. Approximately 90% of the corticospinal fibers cross midline in the caudal medulla, forming the pyramidal decussations, and descend contralaterally in the lateral corticospinal tract to terminate on interneurons and α- and γ-motor neurons in the spinal cord. The remaining corticospinal fibers, located in the medial anterior column, do not cross midline in the medulla but descend ipsilaterally in the anterior corticospinal tract. These fibers ultimately cross midline segmentally near their terminations on interneurons and alpha and gamma motor neurons in the spinal cord. About 55% of the corticospinal fibers terminate in the cervical cord, 20% in the thoracic cord, and 25% in the lumbosacral cord.461 A Brown-Séquard syndrome refers to an injury of the spinal cord in which one side is damaged more than the other (Figure 55-4), resulting in relatively greater ipsilateral weakness and position sense loss, but with contralateral pain and temperature sensation loss.
A corticospinal neuron is known as an upper motor neuron (UMN). The motor neuron to which it synapses in the spinal cord, which exits the spinal cord to innervate muscle, is known as a lower motor neuron (LMN). If damage to the UMNs and LMNs within the spinal cord is localized to a few segmental levels anywhere rostral to the conus medullaris (see below), a constellation of signs and symptoms develops, often called the UMN syndrome. This includes loss of voluntary movement, spasticity, hyperreflexia, clonus, and development of Babinski’s sign.265 If, in addition, there is damage to a significant number of LMNs below the level of injury, loss of voluntary movement occurs without the subsequent development of the other components of the UMN syndrome. Examples of this, defined as LMN injuries, include an SCI caused by an extensive vascular insult to the spinal cord, an injury occurring at the conus medullaris, or an injury occurring at the cauda equina. The conus medullaris syndrome refers to an injury of the sacral spinal cord and the lumbar nerve roots within the spinal canal (Figure 55-5), resulting in an areflexic bladder, bowel, and lower limbs. Conus medullaris lesions localized to the proximal sacral cord can occasionally show a preserved sacral reflex, such as the bulbocavernosus reflex. The cauda equina syndrome refers to an injury to the lumbosacral roots within the spinal canal (Figure 55-6), resulting in an areflexic bladder, bowel, and lower limbs.
After an acute UMN-predominant SCI, initial development of the UMN syndrome is delayed by a process called spinal shock, whereby there is a transient suppression and gradual return of reflex activity below the level of injury. Ditunno et al.150 have proposed a four-phase model of spinal shock. During phase 1, occurring 0 to 24 hours postinjury, there is motor neuron hyperpolarization, manifesting clinically as hyporeflexia. During phase 2, occurring on days 1 to 3 postinjury, there is denervation supersensitivity and receptor upregulation, manifesting clinically with reflex return. During phase 3, occurring 1 to 4 weeks postinjury, there is interneuron synapse growth, manifesting clinically as early hyperreflexia. And finally, during phase 4, occurring 1 to 12 months postinjury, there is long axon synapse growth, manifesting clinically as late hyperreflexia.
Blood is supplied to the spinal cord through two posterior spinal arteries, a single anterior spinal artery, and several segmental radicular arteries (Figure 55-7). The posterior spinal arteries branch from the vertebral arteries and travel along the posterior surface of the spinal cord to supply the posterior one third of the spinal cord. Two anterior spinal arteries also branch from the vertebral arteries, but these quickly unite to form a single artery that travels along the anterior surface of the spinal cord to supply the anterior two thirds of the spinal cord. The anterior spinal artery and the posterior spinal arteries are dependent on contributions from the segmental radicular arteries along the spinal cord to maintain an adequate blood supply to the spinal cord. The segmental radicular arteries travel through the intervertebral foramina from the aorta and divide into anterior and posterior branches that eventually anastomose with their respective spinal arteries. These radicular arteries are not all identical in size or distribution. In the upper thoracic region between T1 and T4, there is little overlap between radicular arterial supplies. Between T12 and L2, there is an anterior radicular artery that is more dominant than its neighbors, called the artery of Adamkiewicz. This artery, usually found on the left side of the body, is an important blood supply to the caudal two thirds of the spinal cord. On reaching the anterior surface of the spinal cord, the artery of Adamkiewicz divides into a small ascending and larger descending branch. The latter travels down to the level of the conus medullaris, where it forms an anastomotic circle with the terminal branches of the posterior spinal arteries. The regions between T1 and T4, and T12 and L2 are areas particularly prone to ischemic damage, because of the importance of individual radicular arteries. The ischemic damage often affects the anterior portion of the spinal cord more than the posterior portion because of the nature of the single anterior and dual posterior blood supplies. In this situation the corticospinal and spinothalamic tracts are affected, while the gracilis tract is often spared. This leads to a syndrome of paraplegia, with loss of pain and temperature sensation, and relative sparing of touch and position sensation, called the anterior cord syndrome (Figure 55-8).
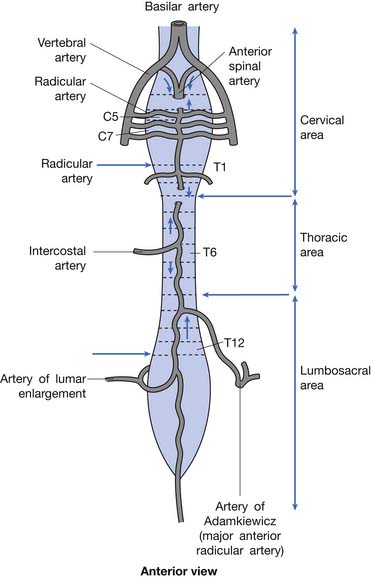
FIGURE 55-7 The spinal cord blood supply.
(Redrawn from Pansky B, Allen D, Budd G: Review of neuroscience, ed 2, New York, 1998, McGraw-Hill, with permission of McGraw-Hill.)
Pathophysiology of Acute Spinal Cord Injury
The secondary injury cascade is a term that refers to a series of biochemical processes that occur after an SCI, and that tend to cause further neuronal damage beyond the mechanical damage caused at the moment of impact. Ischemia of the gray matter at the site of injury occurs almost immediately after SCI. This ischemia appears to result from vasoconstriction of blood vessels supplying the cord, and is mediated by the rapid release of various vasoactive substances such as serotonin, thromboxanes, platelet-activating factor, peptidoleukotrienes, and opioid peptides after SCI.341,393 Ischemia is followed by the development of edema at the site of injury.334 At a cellular level, there is a marked rise in intraneuronal calcium concentrations. This begins within minutes after injury, reaching a peak at about 8 hours postinjury and remaining elevated for at least 1 week.319 Elevated levels of excitatory amino acids, such as glutamate and aspartate, acting at their receptors have been noted to play a role in increasing the intracellular calcium concentrations.100,275 Intracellular calcium facilitates the activation of phospholipases A2 and C, which leads ultimately to the production of free radicals and free fatty acid metabolites, which cause damage to local cell membranes.78,167,370 There is also a rapid rise in potassium in the extracellular space, directly related to cell membrane damage, which causes depolarization of other neuronal cells and conduction block.479Microhemorrhages appear in the central gray matter at the site of impact. Iron in this hemorrhaged blood catalyzes the peroxidation of lipids, leading to further tissue damage as well as catalyzing the further production of oxygen free radicals.393
Initially, neutrophils migrate to the site of injury, where they can contribute to cellular injury by producing lysosomal enzymes and oxygen radicals. These are followed by macrophages that phagocytose cell debris.351 Schwann cells appear that modify myelin sheaths and produce neurotrophic factors, while fibroblasts produce basic fibroblast growth factor and promote angiogenesis and neovascularization at the site of injury.61,271 Small CSF-filled cysts eventually form that are partially surrounded by demyelinated nerve fibers. These coalesce over months and can give rise to myelomalacia and syringomyelia.281 Demyelination of white matter tracts begins within 24 hours of injury and increases thereafter, with Wallerian degeneration occurring by 3 weeks.460 Remyelination seems to occur after SCI, although in an inadequate fashion, with abnormally short internodal distances and notably thin myelin.62,172
Spinal Mechanics and Stability
There is no universally accepted definition of spinal stability. White and Panjabi467 defined clinical instability as “the loss of the ability of the spine under physiologic loads to maintain relationships between vertebrae in such a way that there is not initial damage or subsequent irritation to the spinal cord or nerve roots and, in addition, there is no development of incapacitating deformity or pain due to structural changes.” A commonly accepted model for thoracolumbar stability, which is often used in the middle and lower cervical spine as well, was developed by Denis129,130 and modified by Ferguson and Allen.173 The model divides the spine into three columns: anterior, middle, and posterior (Figure 55-9). The anterior column is composed of the anterior longitudinal ligament, the anterior two thirds of the vertebral body, and the anterior two thirds of the annulus fibrosis or disk. The middle column is composed of the posterior one third of the vertebral body, the posterior one third of the annulus fibrosis, and the posterior longitudinal ligament. The posterior column is composed of the pedicles, facet joints, laminae, supraspinous ligament, interspinous ligament, facet joint capsule, and ligamentum flavum. When the integrity of the middle and either the anterior or the posterior column is affected, the spine is likely to be unstable.129,130 The columns can be compromised by either fracture or ligamentous disruption. Gunshot wounds, because of the nature of the injury, can affect more than one column and the spine can still remain stable.262 It should also be noted that SCI can occur without obvious radiographic findings.
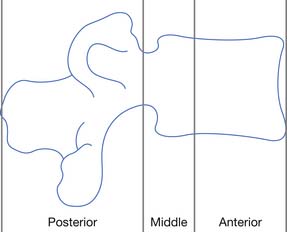
FIGURE 55-9 The three-column concept of spinal anatomy.
(Redrawn from Ferguson RL, Allen BL Jr: A mechanistic classification of thoracolumbar spine fractures, Clin Orthop 189:77-88, 1984, with permission.)
Fractures or dislocations in the thoracic and lumbar spine most commonly involve the T12 and the L1 vertebrae, respectively. Common mechanisms include compression-flexion, distraction-flexion, translation, and torsion-flexion.386 Axial loading of a flexed spine can cause several different patterns of injury depending on the vector of force. There might be only compression of the anterior column leading to a compression fracture or, with a greater compressive force, compression of the anterior column with distraction of the posterior elements. If the vector of force causes the axis of rotation to be anterior to the vertebral body, a Chance-type distraction can occur with distraction of all three columns, through the bony vertebra alone (Figure 55-10), through the ligamentous structures alone, or through a combination of bony and ligamentous structures. In addition, there can be compression of all three columns with retropulsion of the middle column into the spinal canal. The latter often causes SCI. Translation of adjacent vertebrae, as occurs for example when a person falls from a height and strikes part of the torso on an immovable object, is the injury pattern most likely to cause SCI. If there is translation more than 25% of the width of a vertebra, ligamentous structures in all three columns are probably disrupted.195,228 Compression and rotation of the anterior column, and distraction and rotation of the posterior column, cause a torsion-flexion injury where the facets and the anterior longitudinal ligament are usually disrupted, and SCI is likely.
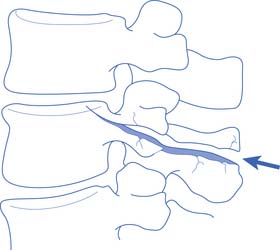
FIGURE 55-10 A Chance fracture.
(Redrawn from Schultz RJ: The language of fractures, Baltimore, 1990, Williams & Wilkins, with permission of Williams & Wilkins.)
A Jefferson fracture, originally described by Sir Geoffrey Jefferson,236 is a burst fracture of the atlas (C1 vertebra). This is caused by axial compression, which can occur, for example, when a football player spears another player with his helmet (Figure 55-11). A hangman’s fracture is a traumatic spondylolisthesis of the axis (C2 vertebra). It is caused by bilateral fractures through the pars interarticularis of the axis that result from hyperextension and axial compression, as can occur in an abrupt deceleration when a person’s forehead strikes the windshield. A fracture of the odontoid process of the axis can be caused by hyperflexion, hyperextension, or excessive lateral bending. The traditional classification of odontoid fractures includes three types.16 Type 1 is a fracture through the tip of the odontoid, type 2 is a fracture through the base of the odontoid, and type 3 is a fracture that extends from the base of the odontoid into the axis proper.
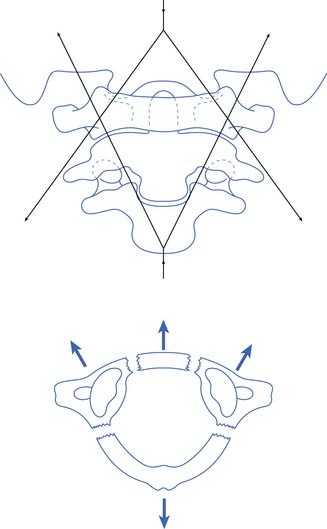
FIGURE 55-11 A Jefferson fracture. A comminuted fracture of the ring of C1.
(Redrawn from Schultz RJ: The language of fractures, Baltimore, 1990, Williams & Wilkins, with permission of Williams & Wilkins.)
Hyperflexion of the subaxial cervical spine (C3–C7) can cause an anterior subluxation, a simple compression fracture, bilateral facet dislocations, a flexion teardrop fracture, or a clay shoveler’s fracture. A flexion teardrop fracture is characterized by retropulsion of the larger portion of a vertebral body into the spinal canal, detached from an anterior fragment (teardrop); it is associated with posterior facet and ligamentous disruption (Figure 55-12). Flexion teardrop fractures are often associated with an anterior cord syndrome, if not a complete SCI. A clay shoveler’s fracture is an avulsion fracture of the spinous process of C6, C7, or T1. It is not typically associated with neurologic injury. Hyperflexion with rotation often causes a unilateral facet dislocation.
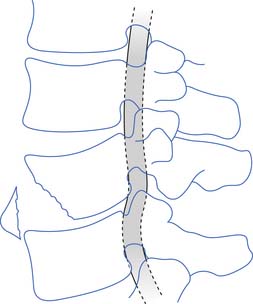
FIGURE 55-12 A flexion teardrop fracture. The spinal cord is compressed by the posteroinferior aspect of the vertebrae.
(Redrawn from Schultz RJ: The language of fractures, Baltimore, 1990, Williams & Wilkins, with permission of Williams & Wilkins.)
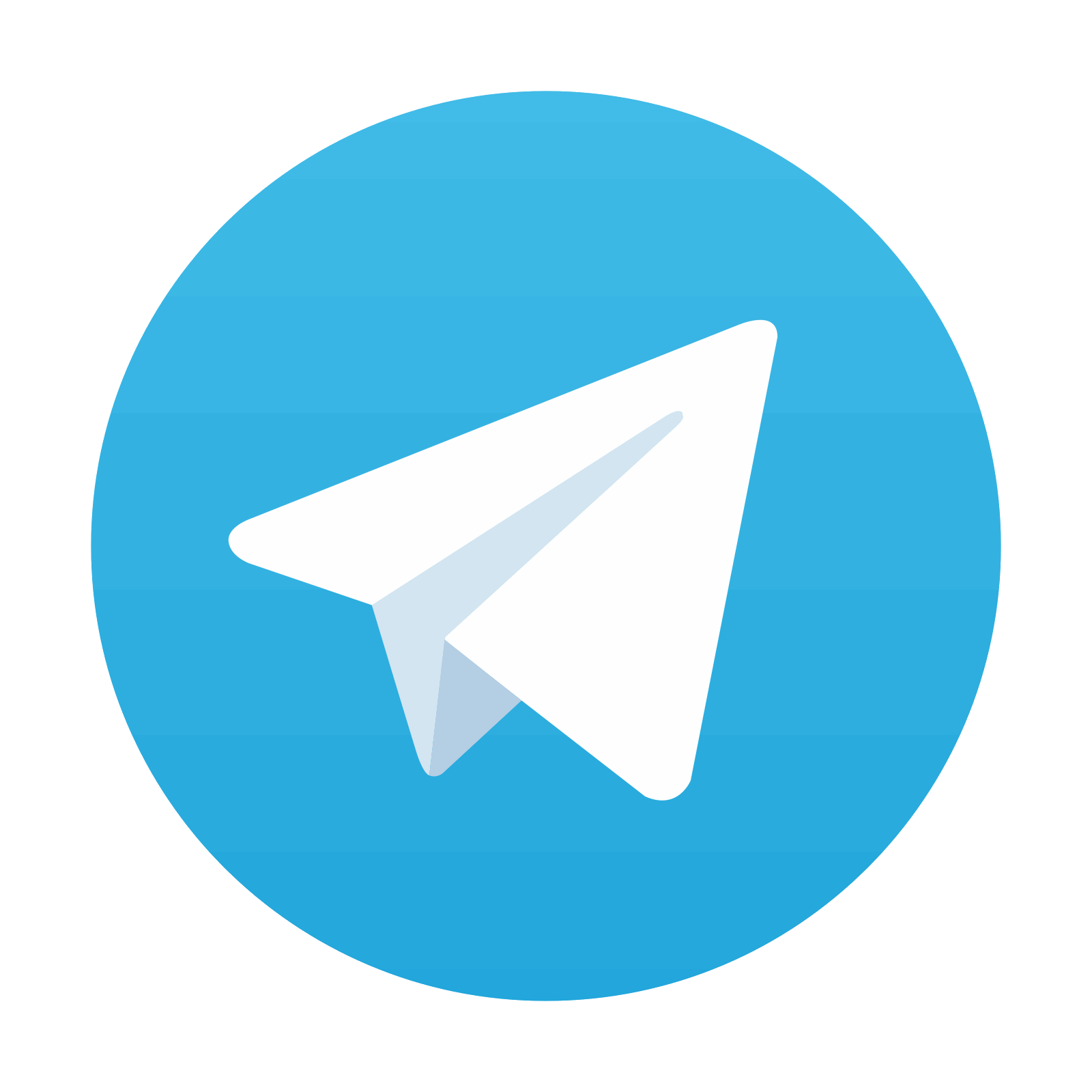
Stay updated, free articles. Join our Telegram channel

Full access? Get Clinical Tree
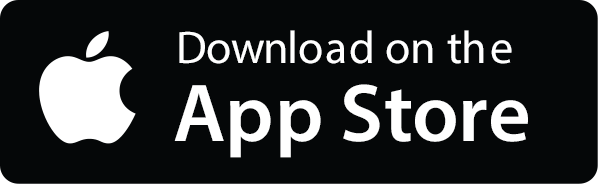
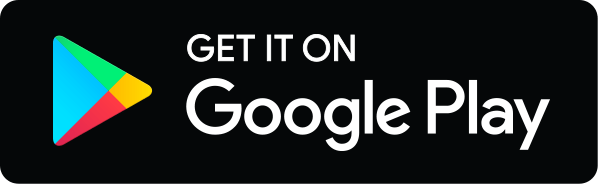