Fig. 8.1
(1) Windup, (2) cocking, (3) late cocking, (4) acceleration, (5) deceleration and (6) follow-through
The first 1.5 s consists of the first thee phases, and the following phase, acceleration, takes only 0.05 s. In this phase the largest change in rotation and also the greatest angular velocities occur. Because of this most injuries happen during the acceleration phase. The last two phases take about 0.35 s.
The shoulder is exposed to very high forces during the throwing motion. The speed of a pitched ball can reach up to 144 km/h bringing up angular velocities in the shoulder up to 7000°/s, and a shoulder is exposed to distractive (950 N), compressive (1090 N) and shear (400 N) forces.
The shoulder and elbow are working through dynamic as well as static stabilisers. The dynamic stabilisers of the glenohumeral joint are the rotator cuff, the long lead of the biceps and the scapulothoracic muscles. The static stabilisers are the glenohumeral capsule, the labrum and the bone. The dynamic stabilisers of the ulno-radial-humeral joint are the flexor, pronator and extensor muscles. The static stabilisers are the joint capsule and the lateral and medial ligaments and the bone. This is mainly in full extension or flexion and less in midrange. These structures can be disrupted by a traumatic event or by overuse.
There is a balance in the shoulder and elbow between stability and mobility. The shoulder needs to be mobile enough to reach extreme positions, for example, maximum exorotation, to achieve maximum velocity. On the other hand it needs to be stable enough to keep the humerus in the glenoid in place and keep a functioning kinetic chain. With every pitch there is a tremendous load on the soft tissue of the shoulder and elbow, which makes it vulnerable to injury. This balance in stability-mobility can be altered and eventually lead to injury in high repetitions and increased demands.
8.3 Specific Preventive Activities for Shoulder and Shoulder Girdle
Based on recent prospective studies, several risk factors for shoulder overuse injury have been defined in a variety of sports, including baseball, handball, rugby and tennis. In general these risk factors can be divided into three categories: (1) loss of rotational range of motion, in particular loss or internal rotation and/or total range of motion; (2) decreased rotator cuff strength, in particular in the external rotators; and (3) scapular dyskinesis. In view of these risk factors, preventive programmes should focus on restoration or maintenance of normal values of these variables, using the appropriate strengthening and stretching exercises.
8.3.1 Glenohumeral Range of Motion
With respect to range of motion, loss of internal range of motion is known to be a risk factor for chronic shoulder pain. Therefore it is advised that side differences in internal rotation ROM should be less than 18°, and the difference in total range of motion should not be more than 5°. The assessment of the ROM into rotation of the shoulder can be measured with a goniometer or an inclinometer and in many positions of the body and the shoulder. A comprehensive reliability study [31] showed high to excellent inter- and intra-tester reliability for a variety of test positions and equipment. Based on the results of this study and in view of optimal standardisation of body and shoulder position, the authors advise the following procedure: the patient is supine with the shoulder in the frontal plane and the elbow flexed 90°. The upper arm should be horizontal, if needed (e.g. in case the patient has protracted shoulders or a thoracic kyphosis), the arm is supported by a towel to reach the horizontal position. For internal rotation the examiner palpates the spine of the scapula and the coracoid. The inclinometer is aligned with the forearm (olecranon and styloid process of the ulna), and the shoulder is moved into internal rotation (Fig. 8.2). The movement reaches its endpoint when the coracoid tends to move against the palpating thumb. For external rotation the fixating hand is gently put over the shoulder top, and the shoulder is moved into external rotation, aligning the inclinometer with the forearm.
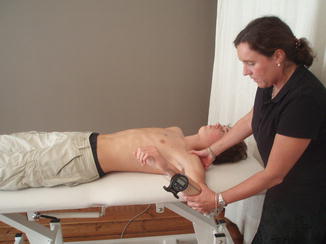
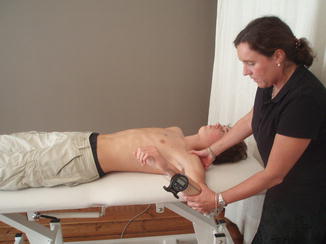
Fig. 8.2
Measurement of internal rotation of the shoulder, using a digital inclinometer
Given the evidenced impact of posterior shoulder tightness on shoulder kinematics, increasing posterior shoulder flexibility is advisory when mobility deficits exceed the limits associated with increased injury risk. Both the cross body stretch (Fig. 8.3) and the sleeper stretch (Fig. 8.4) can be recommended to decrease posterior shoulder tightness. It was shown that a 6-week daily sleeper stretch programme (3 reps of 30 s) is able to significantly increase the acromiohumeral distance in the dominant shoulder of healthy overhead athletes with GIRD. Additional joint mobilisation performed by a physiotherapist has a small but nonsignificant advantage over a home stretching programme alone. No difference in mobility gain was seen after angular (sleeper stretch and horizontal adduction stretch) and non-angular (dorsal and caudal humeral head glides) joint mobilisation by a physiotherapist. Muscle energy techniques (hold-relax) during the sleeper stretch and the horizontal adduction stretch have proven useful to immediately increase internal rotation range of motion.
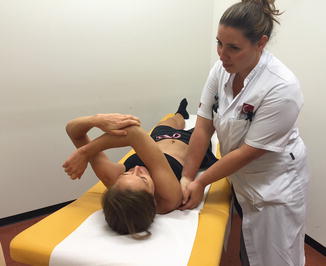
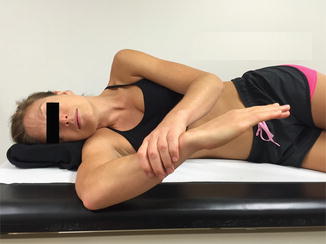
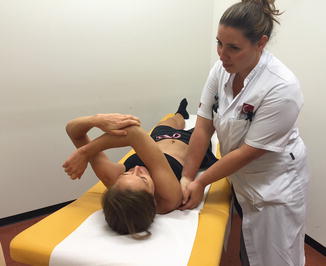
Fig. 8.3
Cross body stretch
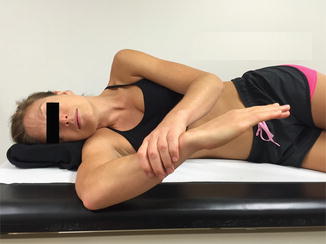
Fig. 8.4
Sleeper stretch
8.3.2 Rotator Cuff Strength
Regarding rotator cuff strength, it is generally recognised that overhead athletes often exhibit sport-specific adaptations leading to a relative decrease of the strength of the external rotators. Absolute side differences as well as muscle balance ratio between external and internal rotators have been found to be altered. In general, with respect to cut-off values distinguishing a healthy shoulder from a shoulder at risk, an isokinetic ER/IR ratio of 66 % or an isometric ER/IR ratio of 75 % (when measured in neutral position) is advised [31], with a general rotator cuff strength increase of 10 % of the dominant throwing side compared to the nondominant side. Recently, focus has shifted from isometric or concentric to eccentric muscle strength of the rotator cuff. In particular the eccentric strength of the external rotators is of interest. These muscles function as a decelerator mechanism during powerful throwing, serving or smashing.
In view of the importance of eccentric rotator cuff strength in relation to injury-free overhead throwing or serving, it is imperative that strength is assessed on a regular basis in healthy as well as injured players. With respect to the isometric strength measurements, hand-held dynamometry (HHD) has attracted more and more interest during the last years due to the more practical, less expensive and user-friendly advantages over the more advanced and expensive isokinetic devices (Fig. 8.5).
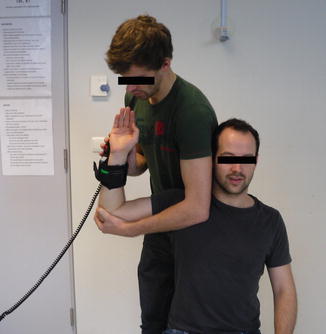
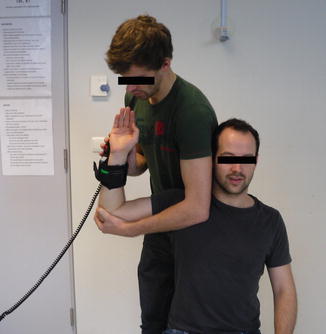
Fig. 8.5
Eccentric testing protocol, using an HHD
Recently, a new testing protocol was published, showing that the use of a hand-held dynamometer (HHD) measuring eccentric external rotator strength has excellent intra-tester (ICC = 0.88) and good inter-tester (ICC = 0.71) reliability, as well as concurrent validity (compared to an isokinetic device, Pearson correlation = 0.78). During the procedure, the patient is seated with gentle support of the arm by the tester; the tester brings the shoulder from 90° abduction–90° external rotation (throwing position) to 90° abduction–0° external rotation, loading the external rotators eccentrically (Fig. 8.4). A large normative database on 200 overhead athletes (volleyball, tennis and handball) was recently set up and shows an average normalised eccentric external rotator strength (N/kg) of approximately 2, with significant side differences in favour of the dominant sides and significant higher values for handball and tennis compared to volleyball.
Numerous exercises have been described to strengthen the rotator cuff muscles, including concentric, isometric, eccentric and plyometric exercises. In view of the eccentric component of the function of the external rotators, the sport-specific exercises for overhead athlete players should focus on three areas:
1.
Accentuate the eccentric phase and “avoid” concentric phase, in order to load the muscles based on their eccentric capacity. Figure 8.6 shows an example of an eccentric exercise for the external rotators in general in an abducted position.
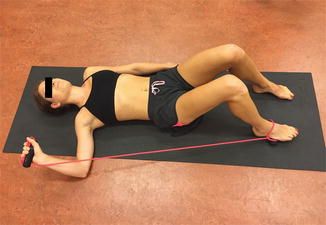
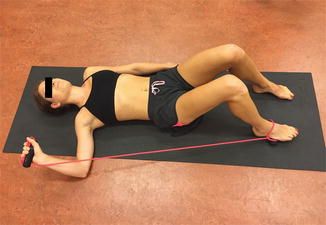
Fig. 8.6
Eccentric exercise for the external rotators in an abducted position
2.
Slow exercises for absolute strength and fast exercises for endurance and plyometric capacity. Endurance and plyometric capacity may be exercised using weight balls, in which the patient is instructed to “catch” the ball (Fig. 8.7), as described by Ellenbecker and Cools.
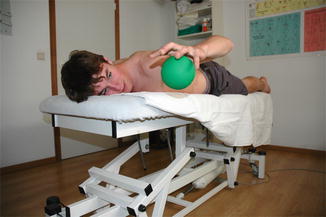
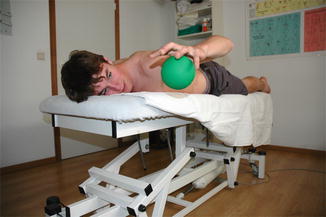
Fig. 8.7
“Catching” exercise, using a Plyoball
3.
Exercise highlighting the “stretch-shortening cycle” of throwing. Specific devices can be used to train the stretch-shortening cycle, such as XCO® trainer (Fig. 8.8).
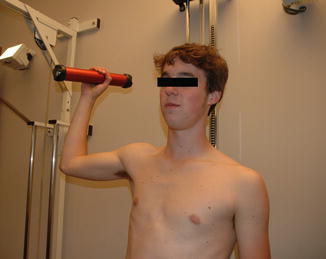
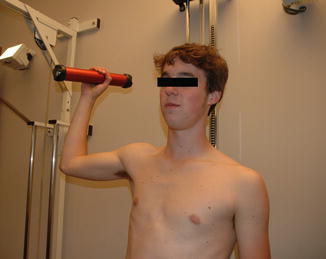
Fig. 8.8
Stretch-shortening cycle exercise, using the XCO© trainer
8.3.3 Scapular Dyskinesis
Evidence supporting cut-off values for prevention of injury or return to play after injury with respect to scapular function is scarce. A number of studies used visual observation as a criterion, whereas others provide objective data on healthy athletes as a reference base for return to play. In general, visual observation is performed either by using the yes/no method (scapular dyskinesis or not), a method proven to be reliable and valid, on the condition that the examiner/therapist is educated in a standardised manner, or by categorising the scapular dysfunction into different types, based on the specific position of the scapula. However, the latter method was shown to have acceptable intra-rater, but low inter-rater reliability. A statement saying that scapular behaviour should be symmetrical in overhead athletes is not supported by research data, on the contrary. In volleyball, as well as in handball players, asymmetry was found in resting scapular posture. Therefore clinicians should be aware that some degree of scapular asymmetry may be normal in some athletes. It should not be considered automatically as a pathological sign but rather an adaptation to sports practice and extensive use of the upper limb.
Several studies measured scapular upward inclination in healthy overhead athletes. These data may be used as a reference base and cut-off values for correct scapular positioning in several elevation angles. In general, a large variety is found in scapular upward inclination in the mid range of motion (probably due to a large variation between individuals); however, in full elevation, most studies suggest that upward inclination should be at least 45–55°.
For the scapular muscles, proper inter- and intramuscular balance should be assessed. Isokinetic ratio protraction/retraction is shown to be 100 % in a healthy population, with slight changes in overhead athletes, in case of throwing athletes in favour of the protractors. In bilateral sports (swimming, rowing, gymnastics), there should be no side differences in scapular muscle strength, and in one-handed overhead sports, an increase of scapular muscle strength of 10 % is advised on the dominant side. In particular, the lower trapezius and serratus anterior should receive special attention, since these muscles are shown to be susceptible to weakness in injured athletes.
Once deficits and imbalances in scapular behaviour are assessed, an intervention programme to restore flexibility and muscle performance needs to be installed. Recently, a science-based clinical reasoning algorithm was published guiding the clinician into the different steps and progression. The main goals are (a) to restore flexibility of the surrounding soft tissue of the scapula, in particular the pectoralis minor, levator scapulae, rhomboid and posterior shoulder structures, and (b) to increase scapular muscle performance around the scapula, focusing on either muscle control and inter- and intramuscular coordination or muscle strength and balance. Exercises to restore scapular muscle balance have been shown to increase isokinetic protraction and retraction strength, increase external rotator strength of the shoulder and alter EMG activity of the scapular muscles in favour of efficient muscle recruitment during a loaded elevation task. In general focus should be put on training the lower and middle portions of the trapezius (Fig. 8.9) and training the scapular muscles in higher elevation angles, relevant to the specific sport (Fig. 8.10).
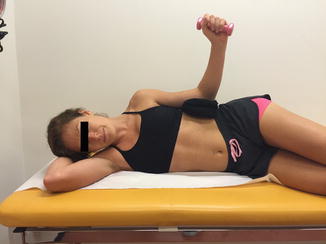
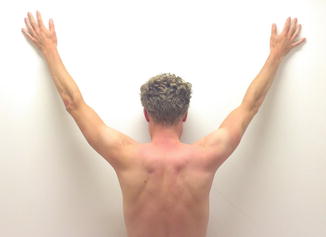
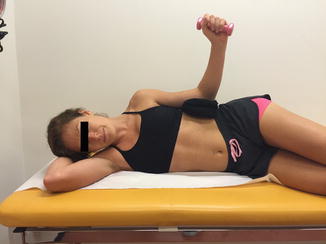
Fig. 8.9
Exercise for lower and middle portions of the trapezius
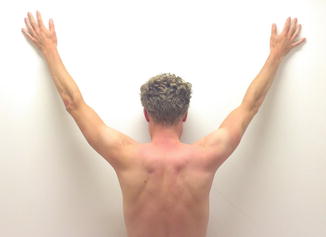
Fig. 8.10
Exercise for the scapular muscles in higher elevation angles
8.4 Specific Preventive Activities for Elbow
Elbow injuries occur throughout many but most in overhead sporting activities [21, 22]. The increase in the number of participants in sports as baseball, tennis, football, javelin throwing and volleyball has probably resulted in a rise in the incidence of elbow injuries in recent decades.
The incidence of shoulder and elbow injury in baseball is related to the number of years of participation, as well as to the age of athlete; elbow pain is reported in 20 % of the 8–12-year-old group, 45 % of the 13–14-year-old group and 58 % of high school and college athletes [17, 18]. The majority can be classified as either overuse or acute traumatic injuries.
The forces commonly encountered at the elbow in sports are best documented in the overhead-throwing athlete. The overhead-throwing motion can be divided in six phases as described earlier: (1) windup, (2) early cocking, (3) late cocking, (4) acceleration, (5) deceleration and (6) follow-through [5, 6]. This motion especially during the late cocking and early acceleration phase and in the follow-through phase generates valgus and extension forces. Valgus stress can reach 64 Nm, with compression forces in the radiocapitellar articulation as high as 500 N and extension velocities in excess of 3000° per second. These combined forces have been termed valgus extension overload (VEO) and are believed to be responsible for most injury patterns seen in the throwing athlete [7]. These forces consist of lateral compressive forces (radial head/capitellum), large tensile forces that occur in the medial structures (UCL, pronator/flexor muscle mass, medial epicondyle) and shear stresses that occur in the posterior compartment (olecranon tip, olecranon fossa). These forces can finally result in attenuation or rupture of the UCL, flexor pronator tendinopathy, formation of olecranon tip osteophytes or loose bodies, olecranon stress fractures and osteochondral defects of the capitellum [8, 14, 15]. Most scientific research focusing on preventive measures for overuse elbow problems is performed in baseball players (by Glenn Fleisig and colleagues) so the advices discussed below are mostly based on baseball players.
8.4.1 Quantity
The risk factor with the strongest correlation to elbow injury is the amount of pitching. Based on the research of Olsen et al., there they concluded that averaging more than 80 pitches per game almost quadrupled the chance of surgery (OR 3.83) and pitching competitively more than 8 months per year increased the odds of surgery by fivefold (OR 5.05). A pitcher who regularly pitched with his arm fatigued was 36 times as likely to have an injury which required surgery (OR 36.18). Little League and high school pitchers who also pitched for travel teams or showcases had increased risk of elbow injuries. Studies show that it is not uncommon for youth pitchers to play 70 games or more per year, often playing more than 8 consecutive months [23]. The rise in overuse elbow injuries in young pitchers corresponds with the extended competitive baseball of recent times.
8.4.2 Biomechanics
Maximum values of internal rotation torque of the shoulder and varus torque of the elbow are produced near the time of maximum external rotation to decelerate shoulder external rotation, prevent elbow valgus opening and initiate shoulder internal rotation. Tension in the UCL absorbs about half the varus torque in this position. Pitchers at the youth level have greater inconsistency in their biomechanics from pitch to pitch [12]. Improper pitching mechanics can lead to increased elbow varus torque and consequently increased risk of elbow injury.
Changes in other parts influence the elbow biomechanics during the throwing motion: trunk range of motion, late arm rotation, excessive shoulder external rotation, passive total shoulder rotation, excessive elbow flexion and improper shoulder abduction and trunk tilt [9, 10, 13, 16]. The study of Huang and colleagues demonstrated that youth baseball players with a history of elbow pain threw with a more extended elbow at maximum shoulder external rotation and greater lateral trunk tilt at ball release [11]. But there is not known if the pitchers with an injury history demonstrated the error prior to the time of injury or if the error developed after the injury. Improving elbow and body biomechanics can increase a pitcher’s chance of staying healthy [24].
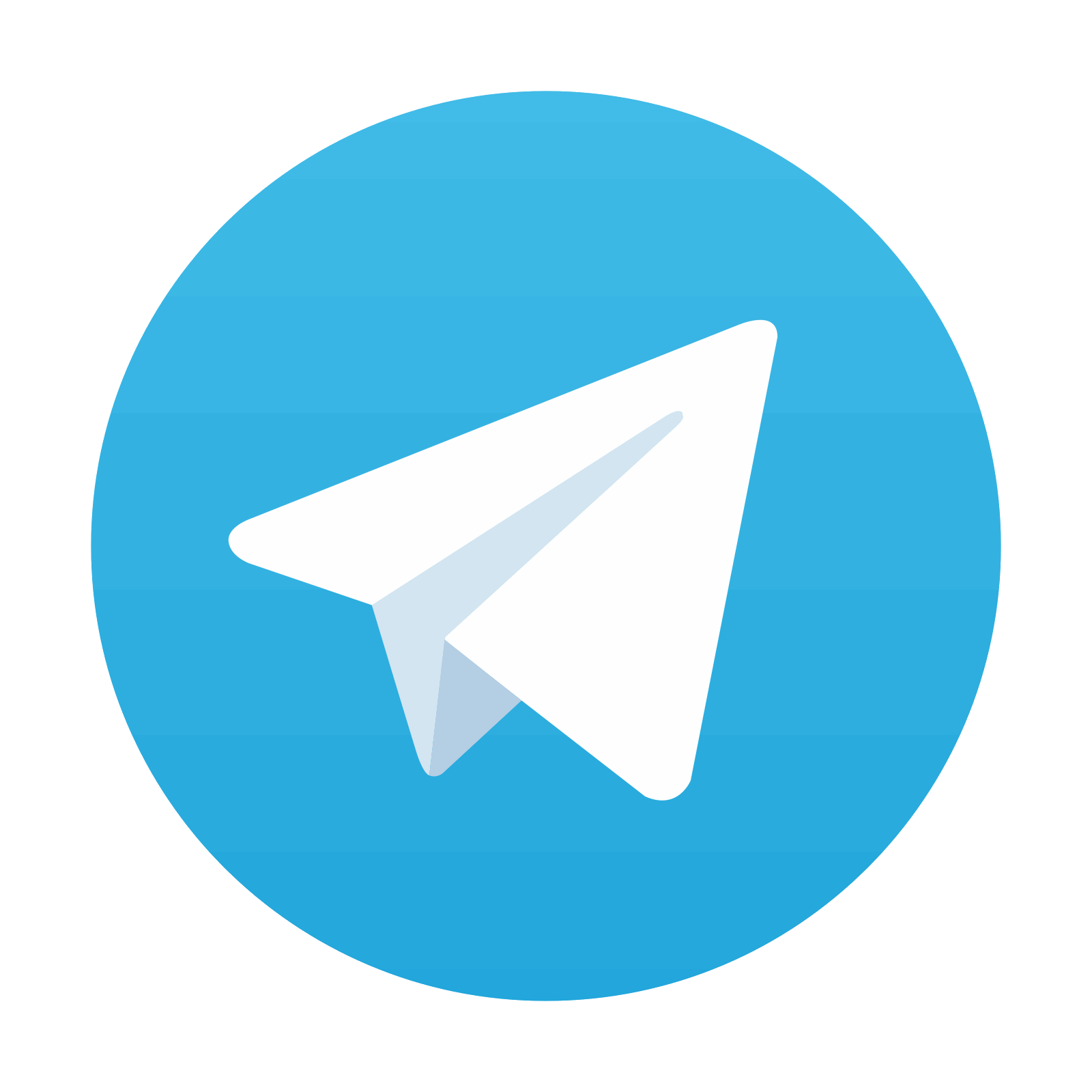
Stay updated, free articles. Join our Telegram channel

Full access? Get Clinical Tree
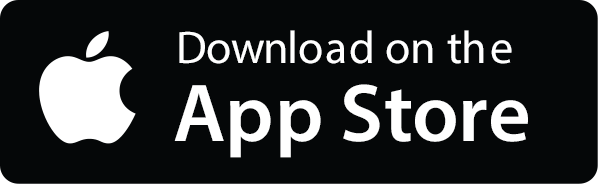
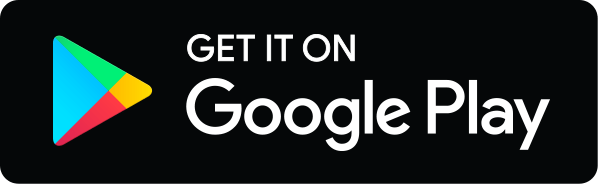