Fig. 40.1
Characteristics of a shock wave: Phase 1: High pressure wave rise time from basic ambient value to a pressure value of approximately 100 MPa within <10 nanoseconds (ns). Phase 2: Wave implosion to a negative pressure value of approx. −10 MPa of within microseconds (Ueberle 1997; Wess et al. 1997; Speed 2014; Gerdesmeyer et al. 2002, 2006; Ogden and Toth-Kischkat 2001; Coombs et al. 2000; Delius et al. 1997; Coleman and Saunders 1993) (www.shockwavetherapy.org)
There are three methods for generating SW: electrohydraulic source (EH), electromagnetic (EM), and piezoelectric (PE) ones. All of them have in common that the waves are generated in water (inside the applicator), as the impedance of water and biologic tissue are similar and comparable; as a result, reflection is limited and waves are better transferred into the body (van der Worp et al. 2013).
The shock wave created by each of these three sources is primarily generated by a controlled high-voltage discharge within a three-dimensional (3D) fluid-filled chamber, causing a transient high-pressure disturbance; each discharge propagates a biphasic sonic impulse within the chamber. This sudden rise from ambient pressure within the chamber creates an extremely short duration broad frequency spectrum (16–20 MHz) SW, that within nanoseconds (ns) of the SW life cycle, creates an initial peak pressure known to rise above 100 MPa (500 bar), which negatively implodes to approximately −10 MPa within microseconds (Fig. 40.1) (Coombs et al. 2000; Delius et al. 1997; Coleman and Saunders 1993; Ogden and Toth-Kischkat 2001). For all of them, SW are produced as a consequence of a rapid increase in pressure (like a “micro-explosion”) into the water, and sooner, for medical purposes, they are concentrated or “focused” on the anatomical “target”; thanks to a parabolic lens, which directs SW, as soon as they are produced from the source (Ogden and Toth-Kischkat 2001; Gerdesmeyer et al. 2002, 2006; Speed 2014). Based on these specific technical characteristics, SW are defined also as “focused” SW (fSW), implying different treatment protocols, for the different types of sources, relatively to the number of shots and the recommended energy levels (expressed as Energy Flux Density in mJ/mm2) to be applied for the different kinds of treatments (Speed 2014).
The rapid sequence of alternating positive and negative phases, during SW propagation, is responsible of the “mechanical” effects (most probably as shear stresses) on the interfaces between different tissues, with their own specific and different densities (acoustic impedance). At higher energies, the negative (or tensile) phase of the SW curve can produce cavitation at the tissue interfaces: gas bubbles are formed and subsequently implode at higher speed, thus generating a second front of SW or microjets of fluid. Overall, these physical phenomena can be responsible, as final result, of the direct (physical) and indirect (biological) effects of SW on the treated tissues (Ueberle 1997; Gerdesmeyer et al. 2002, 2006; Ogden and Toth-Kischkat 2001).
Based on the type of tissue we have to treat (“soft tissues” or bone), it is appropriate to use one specific SW generator (lithotripter), as the energy levels requested for obtaining a certain biological effect are different: briefly, for tendinopathies, low–medium energy levels are adequate, while medium–high ones are requested for bone (Notarnicola and Moretti 2012; Notarnicola et al. 2012; Romeo et al. 2014; Haupt 1997).
Regarding the different modalities of applying SW, national and international recommendations established all essential requirements for a correct treatment (ISMST Consensus Conference, www.shockwavetherapy.org).
40.3 Radial or Pressure Waves (Radial Pulse Therapy or RPT)
In the early 2000s, some other devices featuring “ballistic” or “radial” pressure waves were introduced into the field of ESWT clinical practice. That new technology produced “pressure waves” and not properly fSW, but the term “radial shock waves” was used since that time (Lohrer et al. 2016).
Radial waves (RW also named as Radial Pulse Therapy or RPT) can be considered as “ordinary sound waves” differently generated: in fact, the source (or applicator) is consisting of a barrel handpiece, where, by the action of compressed air or by the influence of a magnetic field, a metallic bullet is accelerated at a very high speed. This high kinetic energy makes it to impact against the tip of the applicator itself, directly applied on the skin; energy is accumulated during run and transmitted directly to the body surface in the target area. Moreover, RW continue to propagate into the body as spherical or ball-shaped waves that are in a radial way, thus deriving the suggestive definition of radial waves (RW). The energy produced by these pressure waves is highest at the level of skin surface but diverges and weakens as it penetrates deeper. Based on the particular mechanism of generation, in Radial Pulse Therapy, the “focal point” is not targeted on the anatomical area (as occurs in fSW) but rather on the tip of the applicator; moreover, RW are not focused in depth but, due to the radial transmission, are restricted to the more superficial layers of the body to be treated. From the physical point of view, although both fSW and RW are acoustic waves, they differ in shape and, although sharing some clinical indications, nevertheless diverge for some other ones (Ogden and Toth-Kischkat 2001; Gerdesmeyer et al. 2002, 2006; Speed 2014; d’Agostino et al. 2015; Lohrer et al. 2016).
As some authors confirmed, the equipments that produce RW, in comparison with those generating fSW, are relatively smaller, cheaper, and easier to handle. Moreover, the maximum energy for RW is delivered at the applicator to skin interface, and fSW peak pressure is about 100 times higher, while the pulse duration is 1000 times shorter (Novak 2015). In any case, the clinical efficacy of RW could be demonstrated for some specific diseases, and nowadays, it is a widely accepted method in clinical practice, with comparable results, although specifically for superficial musculoskeletal disorders (Lohrer et al. 2016; Novak 2015; Foldager et al. 2012; Speed 2014).
Classically, the main differences between fSW (focused SW) and RPT (Radial Pulse Therapy) can be listed as follows (Novak 2015; Lohrer et al. 2016):
Principle of generation = pneumatic for RPT versus electrohydraulic, piezoelectric, or electromagnetic for fSW
Wavelength = 0.15 to 1.5 m (RPT) versus 1.5 mm (fSW)
Maximum pressure = 1 (RPT) versus 10–100 (fSW) MPa
Penetration depth = 2–5 cm (RPT) versus 5–20 cm (fSW)
According to what was described by Alkhamaali ZK et al., it is not correct to characterize RW as low-energy shock wave treatment and fSW as high-energy shock wave treatment. Furthermore, RPT, due to the possibility to generate cavitation, must be differentiated from vibrating devices. The capacity to create a cavitation effect has been demonstrated in new-generation RW devices and sharply separates them from certain vibrating devices that do not generate a cavitational effect (Alkhamaali et al. 2016) (Fig. 40.2).


Fig. 40.2
Divergence between a shock wave (fSW) (on the left) and a radial (pressure or pulse) wave (RPT) (on the right)
40.4 SW Mechanisms of Action: Biocellular Action and Responses
The exact mechanism of action of many clinical interventions remains incompletely elucidated, and ESWT is not excluded from being in the same category. However, advances have been made in recent years to help provide greater illumination of the actions of SW and the biocellular responses derived from this treatment over several indications, including tendinopathies (d’Agostino et al. 2015, 2016).
The science of mechanobiology helps elucidate the actions and responses of ESWT on human tissue, as it assists researchers to analyze and appreciate the effects of SW after it disperses its physical acoustic energy onto tissues and cells, in a process termed mechanotransduction (d’Agostino et al. 2015; Chiquet et al. 2009; Jaalouk and Lammerding 2009; Kolahi and Mofrad 2010). Mechanotransduction describes the processing of an extracellular stimulus (SW), where the force from the stimulus is processed and converted, resulting in a myriad of biochemical responses that influences cellular function (proliferation, migration, apoptosis, and differentiation) (d’Agostino et al. 2015; Chiquet et al. 2009; Jaalouk and Lammerding 2009; Kolahi and Mofrad 2010).
ESWT transmits a SW stimulus that has been known to induce positive biological effects on the cells and tissues, including tendons, thus restoring tissue homeostasis and ultimately function (Notarnicola and Moretti 2012; Romeo et al. 2014; Haupt 1997). This suggests that the homeostatic influence of ESWT on tissue may be considered an indirect, rather than a direct action, yet none the less, an ameliorating intervention (d’Agostino et al. 2015).
The influences of ESWT on the cellular, biochemical, and tissue levels are seen to be multiple and complex. From a cellular level, ESWT is seen to action its influence on pattern recognition Toll-like receptors (TLRs) and macrophages (Holfeld et al. 2014b, 2016; Tepekoylu et al. 2015; Davis et al. 2009; Mariotto et al. 2009; Kuo et al. 2009; Shao et al. 2010; Sukubo et al. 2015), influencing inflammation, angiogenesis, tissue perfusion, and progenitor cell expression, among other factors (Holfeld et al. 2014a; Huang et al. 2013; Tara et al. 2014; Tepekoylu et al. 2013; Visco et al. 2014; Sansone et al. 2012; Ciampa et al. 2005; Gotte et al. 2002; Davis et al. 2009). Its biochemical influences are known to act on neurotransmitters (i.e., substance P or SP and calcitonin gene-related peptide or CGRP), thus assisting in analgesic action and pain modulation even in chronic conditions (Richardson and Vasko 2002; Saggini et al. 2015). ESWT regenerative influences are known to stimulate the progenitor cells of tenocyte, fibroblasts, osteoblast, and endothelial cells, as well as inducing TGF-ß1 production (d’Agostino et al. 2015; Tara et al. 2014; Visco et al. 2014; Sansone et al. 2012; Suhr et al. 2013; Raabe et al. 2013; Kearney et al. 2012; Tamma et al. 2009; Hausdorf et al. 2011; Wang et al. 2002a, b; Tepekoylu et al. 2013), while downregulating pro-fibrotic proteins (i.e., MMP2), reducing fibrous capsule formation, and increasing fibrotic tissue resorption and remodeling (Fischer et al. 2015). These are some of the reasons why ESWT for the management of tendinopathies is gaining more consideration and acceptance.
40.5 ESWT for Tendinopathies?
Tendons, the tough connective tissue of various forms that commonly connects muscles to bone, is primarily comprised of tenoblasts (immature cells), tenocytes (mature cells), collagen, elastin and ground substances. Tendinopathies are a clinical commonality, yet the exact intrinsic pathomechanics of this condition still remains partly unclear. Tendinopathies often present with pain, degeneration, with or without swelling, and with varying levels of debilitations (Sharma and Maffulli 2005; Abate et al. 2009; Scott and Danielson 2009; Cook and Purdam 2009). The disruptions to the tendon’s regenerative process (including aberrances of resident tenocytes proliferation, collagen matrix syntheses), which combined with the ensuing hypoxia, are considered to be some of the primary causes for tendinopathies and further disease progression (Sharma and Maffulli 2005; Abate et al. 2009; Scott and Danielson 2009; Cook and Purdam 2009). Although the presence of overt inflammation has been questioned and in most cases rejected as a primary contributor (Sharma and Maffulli 2005; Abate et al. 2009; Scott and Danielson 2009; Cook and Purdam 2009), yet the presence of covet inflammation, as well as the aberrant immune activity (Agil et al. 2013; Sharma and Maffulli 2005; Rees et al. 2009; Wang et al. 2003; Tsuzaki et al. 2003a, b; Archambault et al. 2002), its role in the maintenance of tendon pathology, and its contributions toward disease progression requires greater elucidation.
The general consensus, to date, is that ESWT may be considered for tendinopathies that are resistant and indocile to conservative management. ESWT is at times recommended in combination with loading exercise routines. At the present time, there is uncertainty in some quarters as to whether ESWT (fSW) or Radial Pulse Therapy (RPT) is more suitable for the management of tendinopathies (Lohrer et al. 2016), and this therapeutic choice remains at the clinician’s individual discretion and expertise.
From the clinical point of view, the most important thing to be underlined is that the maximum energy in RPT is transmitted at the interface between the applicator head of the device and the skin and diminishes its energy inside the treated tissue by the square of the penetration depth (Lohrer et al. 2016). As a consequence, since the beginning, RPT was applied to tendon pathologies, primarily by their immediately subcutaneous localization and by a large area of injured tissue. Midportion Achilles tendinopathy and patellar tendinopathy seem, for example, to fulfill these criteria, and some of the authors demonstrated those can be an indication for RPT (Lohrer et al. 2016).
Based on current evidence, some of the authors in the literature stated to be unable to prefer ESWT or RPT for musculoskeletal soft tissue injuries. Conflicting evidence exists from the results of two studies that directly compared fSW to radial waves in patients affected by plantar fasciitis and patellar tendinopathy (Lohrer et al. 2016). As a whole, in clinical practice, RPT has been proven to be as effective and safe noninvasive treatment option for tendon pathologies in several randomized controlled trials. Currently there is no scientific evidence in favor of either RPT or ESWT (fSW), with respect to the treatment outcome (Speed 2014; Schmitz et al. 2015).
According to the ISMST Consensus Statement (2014), fSW should be applied by a trained physician, while radial waves can be applied, after medical prescription, by physiotherapists or allied health professionals as well, limited to superficial tendinopathies and muscle pathologies (ISMST-website: www.shockwavetherapy.org).
Regarding local anesthesia, nowadays, while still considered as helpful for bone treatments (Schaden et al. 2015; Lohrer et al. 2016), it is not indicated for soft tissue SW applications, including tendinopathies (Schmitz et al. 2015; Lohrer et al. 2016) (as stated also in the ISMST Recommendations), due to the risk of diminishing its therapeutic efficacy. Some experimental researches, in fact, seem to point out that “pain perception” induced by SW application is responsible for releasing neuropeptides (as substance P), thus determining both central and local trophic effects to increase metabolism in bradytrophic tissues (Schmitz et al. 2015; Klonschinski et al. 2011; Lohrer et al. 2016). In an experimental setting, it was demonstrated in fact that “…ESWT dose-dependently activates and sensitizes primary afferent nociceptive C-fibers, and that both activation and sensitization were prevented if local anesthesia was applied” (Klonschinski et al. 2011; Lohrer et al. 2016).
Regardless of the SW equipments, if the treatment is carried out in respect of the rules of “Good Clinical Practice” and ISMST Recommendations, by an expert operator, from a general point of view, this therapy can be considered a very safe and effective treatment (Pettrone and McCall 2005; Wang et al. 2000; Furia 2005); furthermore, no device-related problems nor systemic and/or local complications are reported (Ko et al. 2001).
40.6 ESWT for Tendinopathies: Basic Science
The safe and effective application of ESWT over a decade ago onto the plantar fascia prompted the further exploration of ESWT application in tendinopathies (Notarnicola and Moretti 2012). Seil and colleagues, in 2006, noted the success rates of tendinopathy management with ESWT ranged from 60 to 80% (Seil et al. 2006). What was noted by investigators as to the action of ESWT, when applied on tendinopathies in vivo and in vitro experimental studies, were typical changes in tendon structures in the form of transient dose-dependent inflammatory response; proliferation at tendon-bone complex, along with the release of pro-angiogenic regulatory factors (nitric oxide and vascular endothelial growth factor); and proliferating growth factors (PCNA, TGF-ß1), all assisting in tendon regeneration and functional restoration (Wang et al. 2003; Chen et al. 2004; Bosch et al. 2007, 2009; Berta et al. 2009; Frairia and Berta 2011; Chao et al. 2008; Hans et al. 2009; Vetrano et al. 2011; Leone et al. 2016; Zhang et al. 2011; De Girolamo et al. 2014). Histological examinations in animal models noted that ESWT, on pathological tendons, reduced edema, swelling, and inflammatory cell infiltration. Moreover, after ESWT exposure, the lesion site undergoes intensive progenitor tenocyte proliferation and progressive tendon tissue regeneration, together with increased expression levels in mRNA for TGF-beta1, IGF-1 expression, and collagen type I while suppressing synthesis of matrix metalloproteinase (MMPs), thus suggesting that ESWT may increase mitogenic tendon proliferative responses (Chen et al. 2004; Bosch et al. 2007, 2009; Berta et al. 2009; Frairia and Berta 2011; Chao et al. 2008; Hans et al. 2009; Vetrano et al. 2011; Leone et al. 2016; Zhang et al. 2011; De Girolamo et al. 2014; Notarnicola and Moretti 2012). Lubricin expressions were similarly increased, thus indicating modulation against tendon erosion and adhesions, while providing pain relief as well (Zhang et al. 2011).
Very interestingly, in 2015, in humans, by using microdialysis, Waugh CM and coauthors examined the real-time biological response of healthy and pathological tendons to SW exposure, reporting no statistical differences between the biological tissue response to SW in healthy and pathological tendons. In addition, the biological response to this treatment could be differentiated between possible responders and nonresponders based on a minimum fivefold increase in any inflammatory marker or MMP from pre- to post-ESWT. Based on their research, they suggested that the mechanical stimulus provided by ESWT might aid tendon remodeling in tendinopathy, by promoting the inflammatory and catabolic processes that are associated with removing damaged matrix constituents. The failed response of some individuals may help to explain why ESWT does not improve symptoms in all patients and provides a potential focus for future research (Waugh et al. 2015).
Again, more recently, Leone et al. showed that ESWT is able in vitro to accelerate the differentiation of human primary-cultured tendon stem/progenitor cells, describing how cultures of tenocytes, derived from ruptured tendons, were more susceptible to SW mediated activation, thus suggesting a possible recruitment of undifferentiated progenitors at the site of injury. They were able to demonstrate that SW induce cell proliferation, although in different stages of differentiation and presenting a heterogeneous natural differentiation potential, probably initiated by tendon injuries. Therefore, the authors concluded that aligned with some other reports, the clinical benefits of ESWT can be attributed to the mechanical stress, promoting the in vivo regenerative capacity of the tissue. Moreover, they showed for the first time that ESWT significantly enhances hTSPCs-induced differentiation, as indicated by cytochemical and molecular findings. Taken together, previous reports and the present observations seem to corroborate the long-held idea that ESWT may enhance recruitment, proliferation, and differentiation of tissue-derived stem cells. Leone et al. propose that such treatment, applied on injured tendons, is able to accelerate stem cell differentiation—probably through the release of different cytokines and growth factors—mainly toward tenocytic lineage, contributing to tendon repair. Further investigations will certainly help to better clarify that ESWT clinical benefits are amenable to mechanisms triggering tendon healing promoted by resident stem cells recruitment (Leone et al. 2016).
In addition to all these tissue-specific trophic actions of SW, it is interesting to remember that from a general point of view, ESWT may influence the regulation and modulation of both overt and covert inflammatory markers, including TLRs, macrophages, cytokines, and tumor necrosis factor-alpha (TNFα), thus helping to restore homeostatic return of tendon turnover (Holfeld et al. 2014b, 2016; Tepekoylu et al. 2013, 2015; Davis et al. 2009; Mariotto et al. 2009; Kuo et al. 2009; Shao et al. 2010; Sukubo et al. 2015; Lowbenwein et al. 2015).
40.7 Brief Overview of ESWT in Tendinopathies
Plantar heel pain syndrome. Ogden et al. (2002) conducted a meta-analysis of 20 studies ranging from 1990 to 2000, with a sample size totaling 1655 patients (Ogden et al. 2002). Briefly, one study utilized ballistic RPT, and the others utilized fSW. 18 studies reported success rate ranging from 61 to 88%, all with minimal to no adverse effects. Always Ogden and coauthors, in 2004, published a double-phased double-blind placebo-randomized controlled trial, with a total of 285 patients, where n = 144 were allocated ESWT, (n = 141) and placebo at follow-up (3 months), 67% (ESWT) recorded complete healing compared to 42% some improvement (control). No adverse effects were noted (Ogden 2004; Ogden et al. 2004). Since that time until recent years, many articles were published in the literature on this topic; some of them reporting controversial results about the efficacy of SW in clinical practice. Finally, in 2015, Gollwitzer and coauthors published a randomized, controlled, multicenter study whose objective was to test whether focused extracorporeal shock wave therapy is effective in relieving chronic heel pain diagnosed as plantar fasciitis. The results provided proof of the clinically relevant effect size of focused extracorporeal shock wave therapy without local anesthesia in the treatment of recalcitrant plantar fasciitis, with success rate between 50 and 65% (Gollwitzer et al. 2015).
Achilles tendinopathy (AT). Gerdesmeyer et al. (2015), published a review of six separate studies on chronic Achilles tendinopathy (AT) carried out between 2005 and 2008. Four studies utilized fSW, and two other ones utilized RPT. The authors concluded that both of them were effective for the treatment of AT. There were two reports of mild adverse effect out of a total sample size of 347 across all six studies. There was a consensus that treatments should be carried out without local anesthesia in order to obtain optimal results (Gerdesmeyer et al. 2015).
Patellar tendinopathy. Leal et al. recently reviewed 15 studies from 2000 to 2015, where both fSW and radial waves were utilized for the treatment of chronic patellar tendinopathy (CPT). The author’s conclusions were that ESWT provided good results for the treatment of CPT, where pathological pain scores reduced with functional improvement. They found that there were insufficient grounds to recommend RPT for treating CPT. The authors recommended that ESWT was unsuitable in acute cases of patellar tendinopathy. No significant adverse effects were reported (Leal et al. 2015b).
Lateral epicondylitis. Thiele et al. (2015) reviewed two set of studies allocated into two groups: Group 1 negative results reporting and Group 2 positive results reporting. The studies that described negative results were seven in total, with a total sample size of 700 patients, with a date ranging from 2002 to 2008. The studies that reported positive outcomes were ten in total, with a total sample size of 758 patients, with a date ranging from 1996 to 2012. The authors’ comments were as follows: where negative or inconclusive reporting was the conclusion of each of the study, it was noted that recommended treatment guidelines and protocols of the ISMST were not followed. Some of the flaws noted in these instances were the use of incorrect SW energy levels, inclusion of acute conditions, and control groups receiving ESWT. This highlights the necessity for clinicians to obtain adequate training for the use of ESWT. The overall conclusion was that there was adequate evidence to support the use of ESWT for chronic later epicondylitis. The note to clinicians assessing clinical evidence is to ensure that recommended treatment parameters were being correctly utilized and not to just focus on the general study design. ESWT is effective for lateral epicondylitis where recommended guidelines are applied (Thiele et al. 2015).
Shoulder tendinopathies. Moya et al. (2015) reviewed 17 studies ranging from 1999 to 2013, assessing both calcific (n = 11) and noncalcific shoulder (n = 6) pathologies utilizing both fSW (n = 15) and radial waves (n = 2) (Moya et al. 2015). Some studies compared ESWT to surgical intervention, where investigators found no difference in the outcome between surgical intervention and ESWT. In the studies investigating efficacy of SW for calcific shoulder pathology, all investigators (n = 10) reported significant improvement of pathology after ESWT. The one investigator utilizing rWT similarly reported positive outcomes as well. In the studies investigating efficacy of SW on noncalcific shoulder pathology, one investigator found some short-term benefit, while all other investigators reported no significant change to report. From a general point of view, the authors concluded that (Moya et al. 2015):
SW can be considered an efficacious and efficient alternative to surgery for calcific rotator cuff (RC) tendinopathy, thus a treatment of choice for this pathology, as well as an effective treatment modality when other conservative methods have failed.
Clinical efficacy of SW in noncalcific shoulder tendinopathy is still controversial but with promising perspectives.
Although the available evidence does not allow conclusions of the effectiveness in frozen shoulder, preliminary studies encourage to continue with larger series of patients.
Promising results on other shoulder diseases have been reported, but it lacks solid evidence.
Although the persistence of calcification may be associated with good clinical outcome, complete resorption has better results than partial disappearance or persistence.
Both high- and low-energy SW result effective, although more sessions in the second case are required.
RPT would seem to be effective in calcium deposit resolution as well.
The absence of dense calcification rim around the RC is a good predictor of treatment outcome with SW.
The mechanism of calcium absorption has not yet been elucidated, although some authors proposed neo-lymphangiogenesis as a possible biological substrate (Moya et al. 2015).
Moreover, in calcium deposit dissolution after SW exposure, a possible beneficial interference with some inflammatory pathways could not be excluded and would imply further investigations. This is particularly evident, when considering the influence of SW in the mechanism of innate immunity and self-healing processes, that regulate pro-resolving and pro-regenerative reactions to pathogens and some other noxae (d’Agostino et al. 2015; Holfeld et al. 2014b; Sukubo et al. 2015). In fact, as it has been recently demonstrated for the first time in human histological samples, in calcific tendinitis lesions of the shoulder, there seems to be a significant increase in neovascularization and neoinnervation, along with an eightfold increase in mast cells and macrophages. The findings are consistent with the hypothesis that in calcific tendinitis, the calcific material is inducing a vigorous inflammatory response within the tendon with formation of new blood vessels and nerves (Hackett et al. 2016), and these data surely provide a glimpse on future therapeutic perspectives in calcific tendinopathies.
40.8 Comparison of ESWT with Some Other Therapies
When comparing some alternative therapeutic procedures with ESWT, the greater efficacy of the bio-mechanotransductive properties of ESWT is clearly more evident. Ozturan et al. (2010) compared the short-, medium-, and long-term effects of corticosteroid injection, autologous blood injection, and ESWT in epicondylitis for example. Corticosteroid (CS) injection showed a higher success rate in the short term, while autologous blood injection and ESWT were more efficacious over the long term; there was a higher percentage of recurrence after CS injection. These authors reported a success rate of 50% relative to CS therapy, 83.3% to autologous blood injection, and 89.9% to ESWT (Ozturan et al. 2010). It is pertinent to mention that in another study for the treatment of tendinopathies, ESWT proved to be an effective and noninvasive therapeutic intervention that reduced the need for surgery (Radwan et al. 2008); it was shown to be similarly a treatment of choice for patients where local steroid injections are ineffective or contraindicated (Lee et al. 2012). Rebuzzi and coauthors reported, in a retrospective study comparing arthroscopy and ESWT after a 2 year follow-up, that ESWT was the better treatment option for calcifying tendonitis of the shoulder (Rebuzzi et al. 2008). We found in more recent scientific literature for various tendinopathies that certain agents such as hyaluronic acid injection have been already compared to SW efficacy rates for Achilles tendinopathy (Lynen et al. 2016) and painful noncalcific rotator cuff tendinopathy (Frizziero et al. 2016). Moreover, according to some of the authors, the use of ultrasound-guided percutaneous lavage (UGPL) for calcific shoulder tendinopathies would seem similarly less effective than previously considered (Arirachakaran et al. 2016).
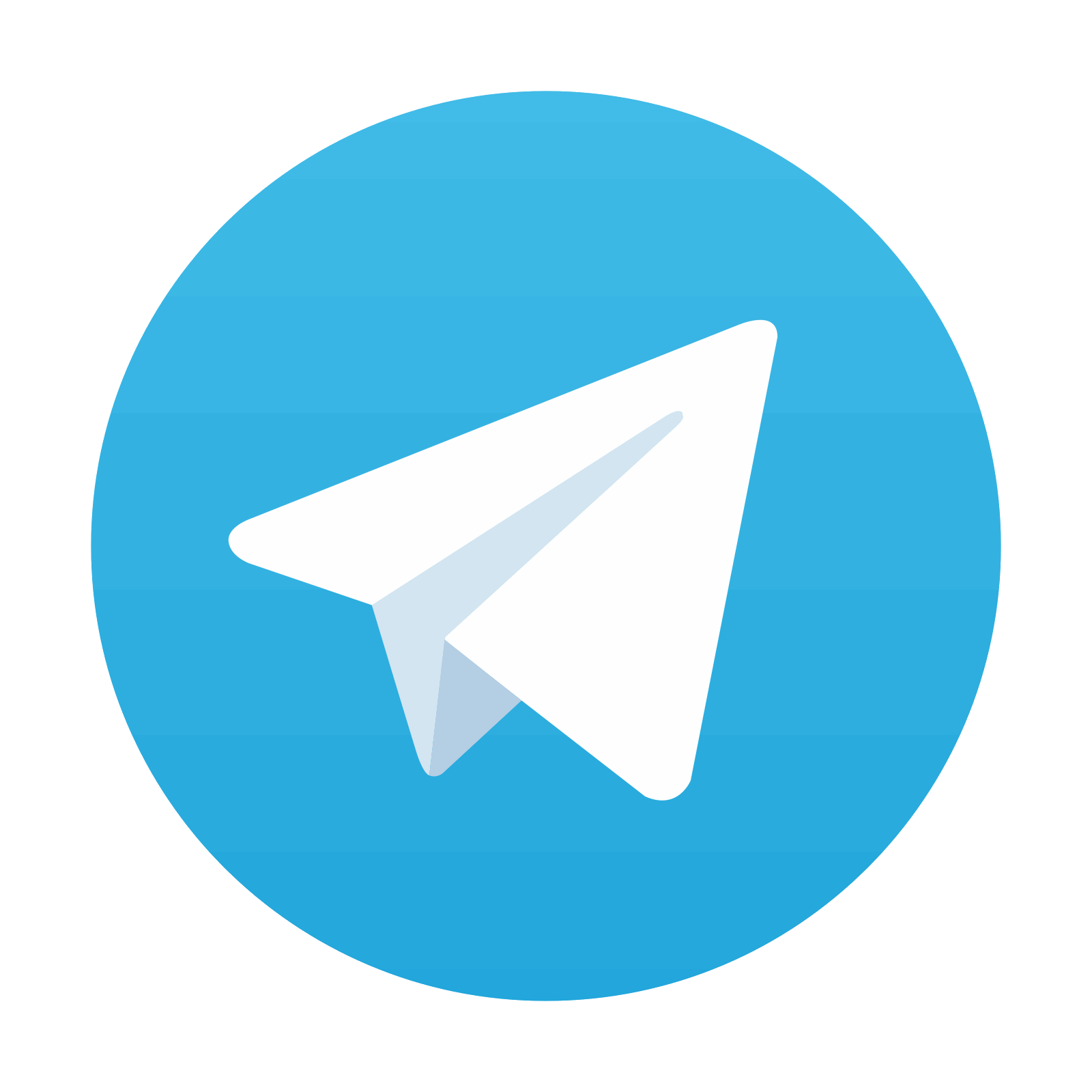
Stay updated, free articles. Join our Telegram channel

Full access? Get Clinical Tree
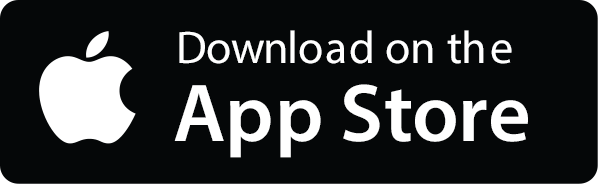
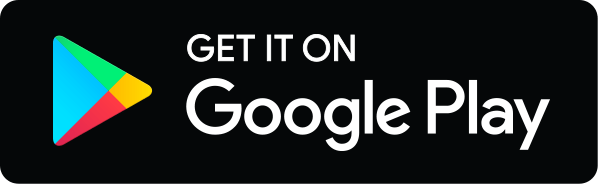