Summary
Adolescent idiopathic scoliosis (AIS) has long been understood as a three-dimensional (3D) deformity. Two-dimensional (2D) imaging techniques have historically been limited in their capacity to accurately demonstrate and quantify sagittal plane malalignment in AIS due to the distortive effects of axial rotation. This led to a historic primary focus on coronal plane alignment in the evaluation and surgical correction of AIS. Modern imaging systems capable of generating computed tomography–like upright 3D reconstructions at low patient radiation exposure have opened new avenues into the assessment of the full 3D deformity in AIS. Increasing utilization of 3D imaging systems in the clinical follow-up and perioperative planning for surgical treatment of AIS have led the Scoliosis Research Society (SRS) to establish standardized reference planes and conventions for 3D analysis. Growing numbers of analyses of AIS have evidenced a discrepancy between 2D and 3D measures, particularly of sagittal alignment. Results from these analyses have led to evolving consideration of the utility of a 3D classification scheme for use in curve evaluation and surgical planning. Concurrent developments in adult literature have repeatedly suggested the importance of sagittal balance in outcomes after spinal fusion. While our capacity to identify and quantify sagittal deformity in AIS has seen significant growth in recent years, the ability of surgeons to utilize this information to target surgical correction in the sagittal plane has lagged. Future work is necessary to utilize our growing understanding of 3D deformity to develop methods for targeted surgical correction of AIS.
Key words
three-dimensional imaging – low-dose biplanar imaging – sagittal alignment – plane of maximum curvature9 Clinical Implications of Three-Dimensional Analysis
9.1 Introduction
Adolescent idiopathic scoliosis (AIS) has long been appreciated as a three-dimensional (3D) deformity. Despite Adams first noting the rotational aspects of the deformity apparent with forward bending more than 150 years ago, characterization of the multidimensional abnormality of the spinal column has been hampered by the limited capacity of the physical examination, and in later years standard two-dimensional (2D) radiographs, to assess the axial and sagittal planes. 1 As a result, the study of AIS has historically focused on 2D alignment, primarily in the coronal plane. The development of modern axial imaging modalities such as computed tomography and magnetic resonance imaging has offered further insight into the deformity associated with AIS, but radiation exposure and cost have limited their routine utilization. Recent years have seen increasing implementation of scanning synchronized upright biplanar imaging systems which allow for the creation of 3D reconstructions of the spine (Fig. 9‑1). The low radiation dosage associated with these systems now provides the capability for routine patient follow-up with 3D imaging. The increasing availability of 3D data on patients with AIS, along with a growing emphasis on sagittal balance as an important factor for patient outcomes after spinal fusion in the adult population, has served to shift increasing focus to the sagittal and axial planes in the evaluation and treatment of AIS.

9.2 History of Radiographic Analysis
Developed in the late 19th century and in widespread use by the middle of the 20th century, the posteroanterior (PA) plain radiograph has historically been the imaging modality dominant in the study of AIS. On such radiographs, the most readily apparent feature of the scoliosis curve is the coronal plane deformity. Because of this, quantification of the coronal deformity has been historically utilized as the primary means to classify/quantify AIS curves via the coronal Cobb measurement. 2 The historical focus on this measurement has led to its use in the characterization of curve severity, risk of curve progression, standard curve patterns, and treatment recommendations. It was on the basis of these measurements that several scoliosis classification systems for AIS curves have reported different coronal curve patterns (and sagittal modifiers) to suggest fusion levels for their surgical management. 3 , 4 , 5 Though more subtle, there is also quantifiable evidence of axial plane deformity apparent on standard PA plain radiographs. While in a physiologic vertebra the spinous process rests equidistant from the pedicles on a PA radiograph, axial rotation of the vertebra in scoliosis results in an asymmetric position of the spinous process in which it translates toward the coronal curve concavity, a feature most apparent at the apical vertebra. As such, several authors have sought to use these plain PA radiographic findings to quantify the AIS deformity in the axial plane, and multiple methods for axial plane classification and quantification have been reported. 1 , 6 , 7 , 8
The sagittal component of the AIS deformity is more difficult to appreciate on plain radiographs, even with lateral imaging. Those studying 2D radiographs of AIS were led to suspect a sagittal plane component of the deformity on the basis of the known coronal and axial plane components of AIS. With a clear coronal plane spinal curvature along with evidence of spinal column rotation, geometrically there was evidence of an additional sagittal plane deformity in AIS. A curve is necessarily longer on its convexity than on its concavity. The axial plane deformity of AIS leads to posterior element rotation toward the curve concavity, implying that the posterior elements rest within the curve concavity while the anterior column lies on the curve convexity. These findings suggest there must be an increase in anterior column length relative to the posterior column, resulting in a thoracic hypokyphosis. However, this thoracic hypokyphosis was less clear on traditional lateral 2D radiographs. This was suspected to be secondary to the axial plane component of the AIS deformity. Stagnara and coworkers sought to circumvent the distortive effects of vertebral axial rotation on sagittal plane measurement when they described the plan d’election, an oblique lateral radiograph rotated to isolate the apical vertebra en face. 9 Hayashi et al built upon this thinking and utilized a digital modification of the method of Stagnara and coworkers to measure local kyphosis at the apical five vertebrae via the plan d’election, comparing this to the value measured on a standard lateral. 10 Their results indicated that the standard lateral plain radiograph significantly overestimated the true local kyphosis. Others also expanded upon this work, utilizing rotated lateral images to identify that with increasing scoliosis severity, the measurement of thoracic kyphosis, as assessed on traditional lateral imaging, was increasingly inaccurate. 11 The loss of thoracic kyphosis associated with AIS was further postulated by Dickson et al in the Leeds Study to be the “essential lesion of progressive idiopathic scoliosis,” a concept supported by others. 12 , 13 , 14 , 15
Despite the obvious clinical and radiographic evidence of the 3D nature of AIS deformity, imaging studies utilizing plain radiographs have been hampered by the limited capacity of a 2D image to adequately characterize a 3D deformity. These limitations have undoubtedly had the most significant impact on analyses of the sagittal plane and its contribution to the deformity of scoliosis.
9.3 Three-Dimensional Reference Plane Definitions
The SRS Classification Committee has defined the reference plane definitions and conventions. The x-axis is anteroposterior (AP). The y-axis is mediolateral, and the z-axis is superoinferior.
Thus, the y–z plane is coronal (AP or PA radiograph), and the x–z plane is sagittal (lateral radiograph). The x–y plane is the axial plane (Fig. 9‑2).

In general, the global reference planes are considered those of the body (pelvis) or those of the X-rays assuming the patient is positioned perpendicular to the X-ray source. Local reference frames can also be defined for each vertebral segment with the x′–z′ plane bisecting the vertebra into medial and lateral halves. Similarly, the x′–y′ plane divides the vertebral body into inferior and superior halves. The z′-axis defined as the average of the vectors normal (perpendicular) to the inferior and superior endplates (Fig. 9‑3).

9.3.1 Coronal Plane
In the normal spine, there is little to no deformity of the spine in the coronal plane. The vertebrae may be tilted forward or backward based on the sagittal alignment, but the vertebrae are not rotated in the y–z plane. Of course, this is the plane in which we traditionally measure the Cobb angle by selecting the vertebrae most tilted from horizontal and measuring the relative rotation between them. Cases of severe kyphosis can make this a challenge, as the vertebrae may begin to appear as an axial projection in the most severe cases. When this is extreme, the y–z global plane is no longer projecting the frontal plane tilt of some of these vertebrae.
9.3.2 Sagittal Plane
The sagittal plane curvatures of the spine that normally exist, kyphosis and lordosis, are easily appreciated and accurately measured in a normal condition (nonscoliotic spine). The global x–z plane is virtually the same as the local x′–z′ reference frames of the vertebral segments. Assessing the degree of flexion or extension between two segments is projected accurately on the lateral radiograph in the normal condition of the spine. Of course, this is the only plane that has some degree of curvature normally, with a relationship between regions of kyphosis and lordosis that result in overall sagittal balance. As a scoliosis develops, there is increasing translation and rotation out of the x–z plane both from progressive axial plane rotation (apex) and coronal tilt (end vertebrae). These movements out of the x–z plane make measurements of kyphosis and lordosis from the lateral radiograph increasingly inaccurate as the scoliosis magnitude increases.
9.3.3 Transverse or Axial Plane
The third plane that 3D reconstructions make viewable is the transverse or axial plane. Whether viewing from above (top down) or below (bottom up), this is the “view” we have never seen on plain radiographs. The vertebrae most deviated in the axial plane tend to be the apical vertebrae. They are translated in the mediolateral direction as seen in the frontal plane, translated AP as seen in the lateral plane, and rotated in the axial plane. The axial rotation is easiest to conceive for the apical vertebra, as this vertebra lies in the transverse plane and thus is easily measured relative to the pelvis. Right thoracic curves have a clockwise rotation in the axial plane when viewed from above.
The axial rotation deformity can also be described between adjacent vertebrae rather than relative to a reference frame of the pelvis. Intersegmental rotation is greatest between segments in the region between curves. For example, in a double major curve pattern such as a Lenke 3C, the greatest rotation between vertebrae occurs within the thoracolumbar region just above and below the neutral vertebra. This may seem counterintuitive, as the neutral vertebra is defined as the least axially rotated vertebra (Fig. 9‑4).

9.3.4 Plane of Maximum Curvature
Given the rotation of the spine associated with scoliosis, there may be a plane different from the global reference planes in which the maximal deformity resides. Normally, the plane of maximum curvature (PMC) is the sagittal plane, as this is normally the only plane in which the spine has a curve. The PMC is defined by three vertebrae of a curve, the two end vertebrae and the apical vertebra. The centroid or middle of these three vertebral bodies can be used to define a plane. This plane has a 3D orientation in space, and the curvature of the spine can be projected into this plane and quantified. In the hypothetical thoracic curve case in which these three vertebrae are within the global coronal plane (the apical vertebra has translated laterally and anteriorly such they all line up on the lateral image), the coronal plane would also be the PMC. Usually, the PMC lies somewhere between the global sagittal plane (normal spine) and the coronal plane. In severe cases with marked rotation and lordosis, the PMC may rotate even past the coronal plane. It is important to differentiate rotation of the PMC and axial rotation of individual vertebrae. The PMC is a means of defining the direction of displacement of the apical vertebra in the axial plane (Fig. 9‑5).

9.3.5 The Role of MRI and CT Imaging in AIS
The advent of modern axial imaging systems allowing for full 3D evaluation of the spine has afforded new opportunities to study the pathology of AIS. The most well-established of these modalities are CT and MRI.
Despite their widespread use in the radiologic assessment of spinal pathology, CT and MRI continue to have a narrow range of indications in the pediatric AIS population. CT offers excellent visualization of bony anatomy and is widely available, but in pediatric and adolescent patients the imaging comes at the cost of much higher doses of radiation. Consequently, the use of CT in scoliosis is largely restricted to the workup of patients with atypical presentation or in whom plain radiographic imaging is concerning for a congenital vertebral abnormality. MRI avoids the radiation risks associated with CT imaging but remains both expensive and time-consuming. While MRI has seen increasing utilization in the preoperative workup of atypical scoliosis to evaluate the spinal cord, it remains impractical for use in routine follow-up and, as such, is largely unavailable for analyses of the 3D shape of AIS curves in large cohorts or longitudinal studies. Though useful in the workup and preoperative planning for nonidiopathic scoliosis, CT and MRI have had a limited clinical impact on the operative or nonoperative management of AIS. These methods are also largely limited to supine or prone (non–weight-bearing) images. There are some upright MRI systems, but their availability remains limited. Despite relatively narrow indications, several studies have been reported utilizing these imaging modalities. Further discussion of these analyses will follow in Chapter 22.
9.3.6 Synchronized Biplanar Radiographic 3D Reconstruction
Recent development and clinical implementation of microdose radiation 3D imaging systems have begun to revolutionize the radiologic assessment of AIS. These systems work by obtaining scanning synchronized biplanar radiographs of the entire spine (or even the entire body) in a standing patient. The paired 2D radiographs are exported for software analysis. Surface maps are then created of each vertebra on the synchronized PA and lateral images. These surface maps are subsequently utilized to generate a 3D reconstruction of the vertebrae and intervertebral discs based on known normative vertebral body anatomy (Fig. 9‑6). Analyses have shown that measurements taken from these 3D reconstructions are accurate within 2 mm of analogous vertebral shape measurements from CT scans, and the radiation dosage of synchronized biplanar radiographic 3D reconstruction is greater than 100-fold less. 16 , 17 , 18 Consequently, these imaging systems not only allow for 3D imaging without associated radiation risks but they also allow such 3D imaging to be performed in the standing position routinely at initial evaluation as well as subsequent follow-ups without exposing patients to significant radiation risk.

There has long been a hope that the knowledge gained by “seeing” the scoliotic spine in 3D would allow a classification system to be developed that would comprehensively characterize scoliosis into 3D curve patterns. The SRS convened the 3D Classification Committee which worked for several years to establish such a classification system. 19 The differences between 2D and 3D measures of scoliosis can be substantial. 20 , 21 The most notable difference is the sheer number of potential 3D measurements (>500 output variables) that can be made in 3D; in fact, there are hundreds that can be made 2D as well. However, with time and experience, we have learned what variables matter most to our clinical decision-making. There is no question: curves that classify as similar in 2D may look very different when assessed in 3D. Several studies have suggested 3D subtypes of the Lenke 1A curve, and another pointed out differences in the Lenke 6C curve type. 22 , 23 , 24 , 25 , 26
Beyond allowing for 3D visualization of the spine, these reconstructions also afford opportunities to perform measurements of vertebral and spinal curve anatomy within anatomic planes of the vertebrae rather than only on the basis of external coronal, sagittal, and axial planes determined by the radiographic source and patient positioning. 27 The axial or top-down view has been extensively studied in AIS. Alterations in the mediolateral position and AP position of a vertebra are depicted with the axial view. In addition, the axial rotation can be better viewed in this plane. Illés et al described an anteriorly directed vector for each vertebra segment that presents a visual of the axial plane rotational deformity associated with scoliosis (Fig. 9‑7). 28 Work by Newton et al utilized such measurements to define a “3D sagittal plane” in which measurements of thoracic kyphosis and lumbar lordosis are calculated based on the local 3D reference planes of each vertebral segment. The summation of these segmental measurements taken from each vertebral body over a region of interest, thereby eliminating the distortion caused by axial rotation/coronal tilt that has hampered traditional lateral imaging, provides a more realistic evaluation of the deformity within the “3D sagittal” plane. 29 These authors differentiated between “global reference frame” measurements, analogous to those taken from a traditional plain radiograph (Fig. 9‑8), and “local reference frame” measurements, generated from segmental summation in anatomic vertebral planes (Fig. 9‑8). These “local” measurements are reported to correspond to a “derotated” and coronally corrected model of the spine.


(b) The pairs in this image are the same as in a, except each pair has been rotated in space to present a true lateral perspective in each of the local reference frames of the vertebrae pairs. In this view, the true flexion–extension (kyphosis–lordosis) at each level is evident.
(c) 3D representation of the sagittal alignment that was created by “restacking” each vertebrae pair from b while maintaining the local kyphosis/lordosis at each segment. This restacked segmental image allows visualization of the sagittal plane deformity of thoracic hypokyphosis that is common in AIS.
3D imaging systems offer a multitude of benefits applicable to surgical treatment and research analysis of AIS. 3D reconstructions allow surgeons to visualize scoliosis deformity as it is in vivo, facilitating opportunities for preoperative planning and postoperative correction assessment. 30 , 31 The capacity for virtually “derotating” the axial plane deformity to allow measurements in anatomic planes of the vertebrae offers the opportunity to study the sagittal plane component of AIS without interference or distortion of axial rotation that has historically plagued analyses of sagittal deformity in AIS (Fig. 9‑8a–c). Additionally, the limited radiation associated with these reconstructions facilitates accurate 3D imaging analysis over long-term follow-up, creating opportunities for longitudinal studies of the change in the scoliosis deformity over time.
Biplanar imaging systems are becoming more popular due to the reduced radiation compared to standard digital radiography as well as the 3D reconstruction capability. However, the systems are more expensive than traditional digital radiographic systems and their availability is largely limited to centers with high-volume spinal deformity (and/or lower limb deformity).
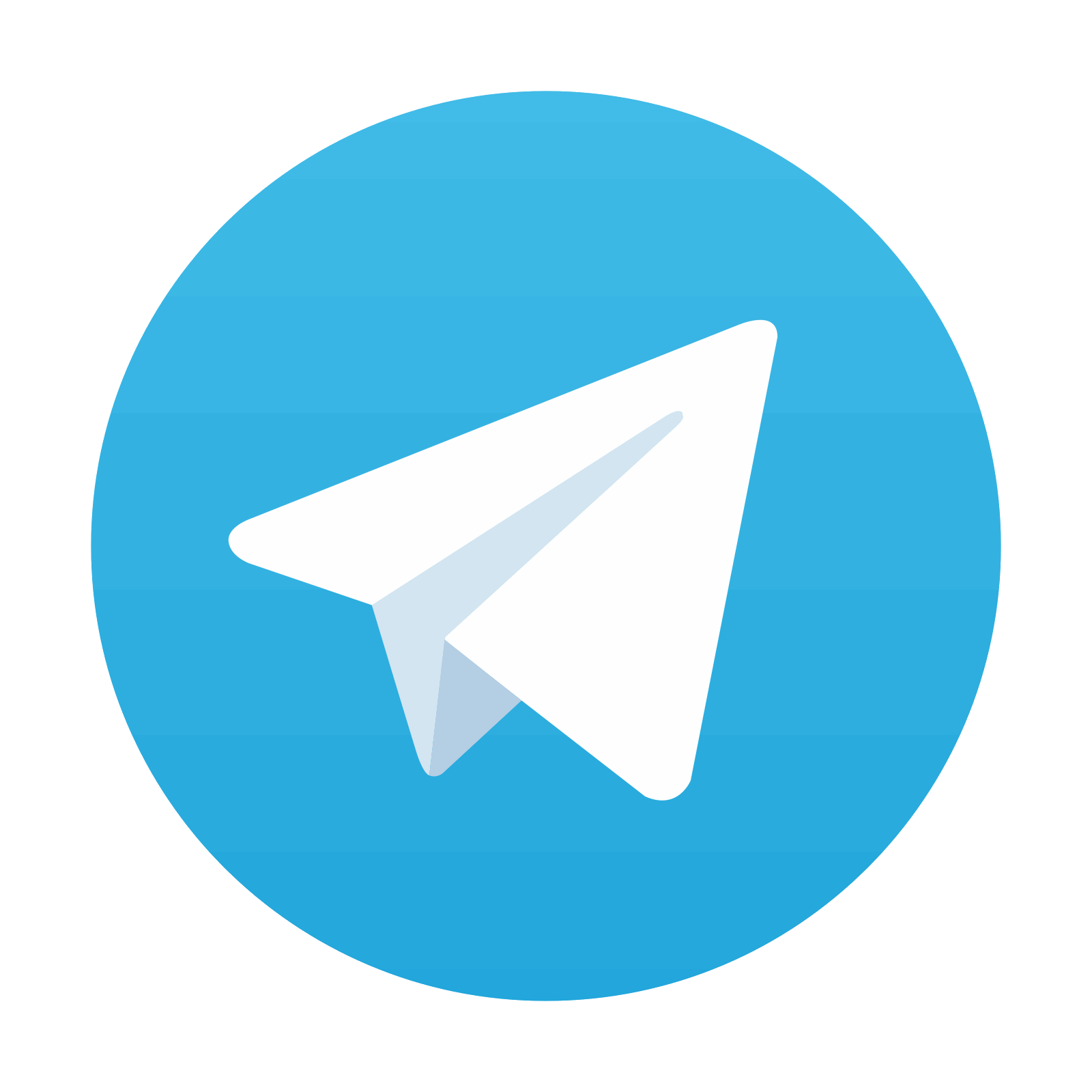
Stay updated, free articles. Join our Telegram channel

Full access? Get Clinical Tree
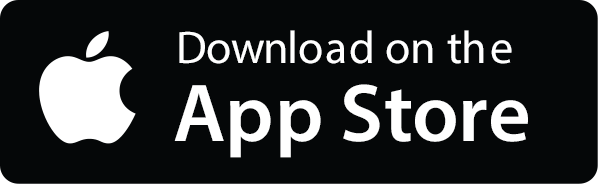
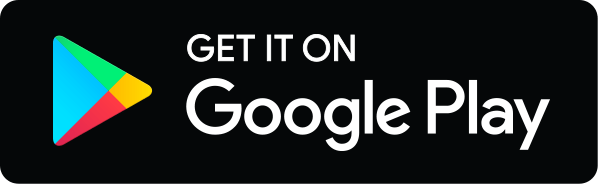
