Summary
The clinical and radiographic evaluation of the pediatric patient with spinal deformity starts with a thorough history to elucidate any signs or symptoms typical of nonidiopathic scoliosis. Following this history is the physical exam to assess for dermatologic manifestations of neuroaxis anomalies, a comprehensive upper and lower extremity neurologic exam including the abdominal reflex, and observation of the patient from behind assessing waist asymmetry, scapular prominence, shoulder heights, and the Adams forward bend. Low-dose radiographic evaluation via a slot scanner provides the surgeon with images of the entire spine in the coronal and sagittal to measure Cobb angles. This technology is used throughout the entire treatment process (observation, bracing, preoperative, postoperative) to limit radiation exposure to radiosensitive tissue in pediatric patients. Treatment algorithms are patient specific, varying based on curve type, curve magnitude, curve location, underlying diagnosis, and skeletal age. Assessment of skeletal age is critical for making informed decisions regarding starting, continuing, or stopping bracing. Various radiographic classification systems exist for assessing skeletal maturity including the Sanders classification, humeral head, distal radius and ulna, thumb ossification composite index, and Risser sign. With no perfect system, combining all the assessment tools to identify where a patient falls on the skeletal maturity timeline is essential in the clinical and radiographic assessment and treatment of idiopathic scoliosis.
Key words
atypical scoliosis – Adams forward bend test – slot scanning technology – curve flexibility – skeletal maturity4 Clinical and Radiographic Evaluation of Patients with Scoliosis
4.1 Introduction
Children present to the pediatric orthopaedic surgeon for a scoliosis evaluation for various reasons but mostly because of a school/pediatrician screening, preseason athletic physical exam, or an incidental finding. The American Academy of Orthopaedic Surgeons (AAOS), Scoliosis Research Society (SRS), Pediatric Orthopaedic Society of North America (POSNA), and American Academy of Pediatrics (AAP) all support screening examinations for spinal deformity for girls aged 10 to 12 years and boys aged 13 to 14; however, literature exists supporting arguments for and against the screening process. 1 , 2 , 3 In this chapter, we review the clinical and radiographic workup for a pediatric patient with scoliosis.
4.2 History and Clinical Presentation
Adolescent idiopathic scoliosis (AIS) is a silent pathology often going unnoticed and leading to the common presenting statement, “I’m here because someone told me I have scoliosis.” Although it is known to not cause symptoms in this patient population, many children simultaneously present with back pain. 4 Severe pain, neurologic weakness, radicular symptoms, bowel or bladder incontinence, night pain, or pain with specific activities are extremely uncommon in patients with AIS and must be explored. Understanding the other common clinical entities that cause back pain in the pediatric patient (spondylolysis, spondylolisthesis, Scheuermann kyphosis, apophyseal ring fracture, overuse syndromes, spondylodiscitis, vertebral osteomyelitis, and neoplasm) can help direct the patient encounter and sort out the non-AIS causes of discomfort. 5 A painful scoliosis should warrant inspection for possible osteoid osteoma or osteoblastoma, as this pain characteristically occurs at night and is relieved by nonsteroidal anti-inflammatories. 6 Certain athletes (weight lifters, gymnasts, cheerleaders) are at higher risk of developing spondylolysis and spondylolisthesis and thus must be questioned appropriately during the history. Other aspects of the history that may play a role in developing spinal deformity include chest wall surgery at an early age, which has an association with the development of scoliosis later in life, or cancer, as chest wall radiation and laminectomy for tumor excision can alter growth plates and/or cause muscle scarring, resulting in spinal deformity. 7 , 8 , 9 Questions regarding the history of developmental dysplasia of the hip, foot deformities, or limb length discrepancy can direct the physical exam to help elucidate potential compensatory scoliosis for an underlying different pathology. 10 A concerning fact parents may offer is that of a patient who had regressed in developmental milestones when seen in the setting of an atypical curve (left thoracic). Advanced imaging is needed to evaluate for intraspinal anomalies, including tethered cord, syringomyelia, intraspinal tumor, herniated disc, Chiari malformations, muscular dystrophy, or diastematomyelia. Table 4‑1 represents common nonidiopathic causes of scoliosis, with the associated clinical manifestations, that must be recognized as different from those of AIS.
Assessing the timeline of when the scoliosis was first identified (rib prominence or trunk asymmetry) to presentation can provide information regarding the rate of progression. Time relative to menarche in girls is critical, as it is related to peak height velocity (PHV) and the likelihood of deformity progression. 11 A positive family history of scoliosis may be associated with AIS as well as possible curve progression and thus should also be elucidated during the historical part of the encounter. 12 , 13 Lastly, a diagnosis of scoliosis can lead to a referral for critical evaluation by other medical subspecialists when diagnoses associated with scoliosis (e.g., Marfan syndrome, neurofibromatosis, congenital scoliosis) are made for the first time in the patient. 14 , 15 , 16
4.3 Physical Examination
A key component of the pediatric physical exam is ensuring the patient is comfortable with the physician, and attempts to ensure patient modesty are critical to gaining that trust. Observation is the first part of the exam, assessing the overall balance (head position compared to pelvis) and alignment of the patient from a distance while ambulating. With the patient’s back facing the physician, the patient’s gown is loosened but not removed so that the back can be examined from the gluteal crease to the occiput. This will allow for the assessment of shoulder balance, trunk asymmetry, thoracic or lumbar prominences, pelvic height, and muscle fullness (trapezius; Fig. 4‑1 and Fig. 4‑2). The anterior chest should be assessed for pectus deformities as well as anterior rib asymmetry. Bending the patient to the right and the left while still examining from the back allows for the assessment of the prominence reduction, therefore giving an idea regarding the flexibility of the individual deformities. Examination of the skin of the back follows for cutaneous lesions (freckling, café-au-lait spots, vascular lesions, skin dimpling, dermal sinus tracts, and/or hairy patches) all of which could be indicative of underlying intraspinal anomalies, as well as previous surgical incisions.


The neurologic exam performed on pediatric patients with spinal deformity is vital to not miss any underlying anomalies. Asking the patient to ambulate in the hallway and walk on the toes as well as the heels can bring to light subtle deficits. Upper and lower extremity muscle strength should be assessed. Sensation, tone, and reflexes need to be assessed, including the deep tendon reflexes as well as the abdominal reflex. Abnormalities in the abdominal reflex may indicate underlying intraspinal pathology. 17 Studies suggest that abdominal reflex abnormalities exist in 11% of “idiopathic” scoliosis patients with asymmetry on the convex side often suggesting the presence of a syrinx, while an absent reflex may be associated with other neuroaxis anomalies. 18 A cranial nerve exam focusing on ocular eye movements and assessment of pupillary size can also provide information regarding brain or spine abnormalities. Any abnormal neurologic finding on the physical exam, or if scoliosis of greater than 20 degrees is present prior to the age of 10 years, requires magnetic resonance imaging (MRI) evaluation. It is not uncommon for patients with presumed idiopathic scoliosis to have intraspinal pathology identified on MRI. 19 , 20
The Adams forward bend is a screening tool historically used by school nurses and pediatricians to evaluate for scoliosis at well-child visits (Fig. 4‑3). It identifies the rotation in the chest wall that occurs with scoliosis. Prominences in the upper thoracic, thoracic, and lumbar spine can be evaluated and assessed. During the Adams forward bending maneuver, flexibility can be assessed by lateral bending and checking for resolution of the prominences. At this time, a scoliometer may be applied to the patient’s back to assess rotation (Fig. 4‑3). The scoliometer is a spirit level that can quantify rotation, with research suggesting patients who have a scoliometer angle less than 5 degrees can be dismissed, while those over 7 degrees should be referred for radiographic evaluation following the screening. 21 Although the interuser reliability of the scoliometer has great variation, it is still useful as a screening tool and for assessing the progression of deformity. 22 , 23 Although with new technology radiation exposure with radiographs has been decreased substantially, the scoliometer may be used with frequent clinical examinations as a technique to prolong the time between radiographic evaluation and decrease total radiation dose.

Limb length discrepancy can induce an apparent spinal curvature that is not recognized until the child’s scoliosis evaluation at the orthopaedic surgeon’s office. Limb length discrepancy is common, often mild in nature, and rarely requires surgical intervention unless the discrepancy is greater than 2 cm. 24 Measurement of the limb length discrepancy historically was done using a conventional scanogram, but recent literature on EOS imaging suggests it is an equivalent imaging modality with lower radiation exposure. 25 A limb length discrepancy results in a pelvic obliquity that can be leveled with standing blocks. The associated spinal curvature from the limb length discrepancy has the concave aspect of the curve directed toward the higher side of the pelvis and longer extremity. Unilateral foot deformities and calf asymmetry are two other common limb abnormalities that can provide clues to the orthopaedic surgeon when evaluating for spinal pathology. 26 , 27
Evaluation at the orthopaedic surgeon’s office for scoliosis can be a frightening event for both the parents and the patient. This can be due to the exhaustive online research often detailing horror stories and pictures of outdated braces. Ultimately, the patient’s biggest concern is being held back compared to his or her peers by not playing sports or missing school, while parents fear long-term sequelae such as severe back pain as an adult, neurologic deterioration, and surgical intervention. Although the majority of children with scoliosis are treated with nonsurgical modalities, the profound effect of a brace on a teenager should not be taken lightly. However, recent literature from the BrAIST study reveals no correlation between the quality of life (QOL) or body image with brace adherence in female adolescents with AIS being treated with a brace, and this reassurance should be passed on to both patient and parents. 28
4.4 Radiographic Evaluation
Standard imaging for scoliosis allows for quantification of the curve magnitude, evaluation for nonidiopathic causes of spinal deformity, surgical planning, and evaluation of other associated spinal column pathologies, such as spondylolisthesis. 29 , 30 , 31 Minimizing radiation exposure is paramount due to the need to follow spinal deformity radiographically with multiple imaging studies through periods of growth. 32 Thus, the physicians and the technologists must be trained appropriately to safely acquire the images needed to evaluate scoliosis and decrease the need to repeat studies. EOS imaging has revolutionized spinal deformity management by bringing the concept of “as low as reasonably achievable” (ALARA) to new levels over the past decade. 33 , 34 , 35
Historically, the two most common radiographs to evaluate patients with scoliosis were standing posteroanterior (PA) and lateral views on a full-length cassette (14 × 36 in.). Both images must include the lower cervical spine with the shoulder to the pelvis allowing for assessment of balance in both the coronal and sagittal planes, spinopelvic parameters, and an assessment of skeletal maturity (Fig. 4‑4). When evaluating the PA radiograph, it should be viewed as if the physician is physically examining the patient’s back, which is the same position the spine is found when prone on the operating room table for spinal fusion. Consistency is key when obtaining serial radiographs of a spinal deformity and thus the technique, laterality, and patient position must be marked so that they can be repeated each time, as varying position or technique could affect a decision on curve progression. If the patient cannot stand, then an erect sitting radiograph is an acceptable alternative. This is key if the patient has a large limb length discrepancy, is minimally ambulatory, or is wheelchair bound. Special radiolucent chairs and protocols exist to allow evaluation of the nonidiopathic patient radiographically with the EOS imaging to keep radiation exposure ALARA. 36 Gravity is known to change the radiographic appearance of a spinal deformity, and this must be considered when assessing curve magnitude and balance from a study taken in the supine position. Blocks should be placed under the short limb in the setting of a limb length discrepancy to level the pelvis; if the discrepancy is too large for blocks, then the X-ray may be performed in the sitting position. Regardless, iliac wings must be level and visualized in their entirety (Fig. 4‑4a).

Position of the extremities at the time of radiographic evaluation of the spine has undergone extensive research since the introduction of the EOS imaging technology as well as the renewed interest in assessing skeletal age on a single spine radiograph. 37 , 38 , 39 , 40 On the coronal EOS evaluation, placement of the hands in a staggered position around the head allows for simultaneous Sanders classification assessment at the time of curve magnitude evaluation (Fig. 4‑5). Other possible positions include arms adducted at the side with the forearms supinated for the evaluation of the proximal humerus physis. 37 For the lateral X-ray, literature exists supporting placing the hands clasped on the clavicles and being forward flexed to 30 degrees to limit disrupting the sagittal balance and spinopelvic alignment while ensuring evaluation of the entire spinal column. 39 However, critical radiographic evaluation of the patients regardless of the view is a standard process allowing for comparison between patients and a single patient’s serial imaging.

Visualization of congenital anomalies and assessment for anteriorly wedged vertebrae, as in Scheuermann kyphosis, at the apex of a sagittal plane deformity are identified on the lateral X-ray (Fig. 4‑6). A single spot lumbar spine X-ray is rarely needed if the long lateral X-ray is performed correctly when attempting to evaluate for spondylolysis or spondylolisthesis in a patient with possible spinal deformity and back pain (Fig. 4‑7). An AP Stagnara view, a plain radiograph performed with the X-ray beam perpendicular to the patient’s rib cage at the apex of the deformity, can help assess pedicle morphology that is obscured by rotation on the PA radiograph. Another additional plain radiograph study is the Ferguson view (Fig. 4‑8) of the lumbosacral junction to assess low lumbar hemivertebrae and sacral obliquity. The X-ray beam is oriented perpendicular to the estimated sacral inclination allowing for AP visualization of the sacrum, which normally requires about 30 degrees of cephalad angulation of the beam.



Curve flexibility, including its radiographic assessment, is a large part of evaluating and managing spinal deformity. It assists largely with surgical planning (instrumentation levels, osteotomy location/type) and prediction of correction. A large volume of literature has reported on the various techniques to perform flexibility assessments, and debate remains regarding supine versus prone, fulcrum versus lateral bending, and traction awake versus under general anesthesia for which the best information can be reproducibly acquired. 41 , 42 , 43 , 44 , 45 Side bending and fulcrum bending radiographs require patient participation; fulcrum bending requires less patient participation, but appropriate bolster position by the technologist and standardization of both techniques is notoriously difficult (Fig. 4‑4). Each curve type also responds differently to the various flexibility imaging studies. For example, the main thoracic curve has maximum flexibility assessed via fulcrum bending X-ray, while upper thoracic and thoracolumbar/lumbar are best assessed with a side bending X-ray. 41 Traction and prone push under general anesthesia offer additional information on the correction of the main curve and response of the nonstructural curve but should not be done other than at the time of operative intervention due to the need for an additional anesthetic and its effect on a child’s developing brain. On large, stiff curves, traction intraoperatively prior to the start of surgical intervention can help the decision-making regarding a need for an anterior release. 45 Researchers are currently evaluating whether soft-tissue texture on advanced imaging can be used to help assess flexibility in spinal deformity. 46 When assessing for flexibility of a hyperkyphotic segment, a backward bend over a bolster is appropriate with the patient in a recumbent position (Fig. 4‑6b).
EOS imaging: In 1992, Georges Charpak won the Nobel Prize in physics for his work on the gaseous X-ray detector that later led to the development of the EOS imaging system. EOS uses a thin fan-beam collimation technique to reduce radiation scatter exposed to a patient compared to conventional techniques while simultaneously producing a high-quality image. The EOS imager is set up like a phone booth; the child stands in a slotted area with a wall on the side and in front containing the imaging technology, allowing for simultaneous biplanar image acquisition over the course of approximately 7 seconds. EOS imaging is calculated by exposing a patient to six- to sevenfold less radiation than a conventional X-ray and is currently recommended for use in the preoperative and postoperative setting. 47 , 48 Recent reevaluation of the imaging modality resulted in a “micro-dose” protocol that allowed for similar pre-/postoperative radiographic measurements at a 45-fold decrease in radiation exposure. 33 Given this technology and the ALARA concept, EOS is rapidly becoming the standard of care. 34 , 49 , 50 This chapter may be generalized for surgeons and institutions where EOS is unavailable. Further support for the use of EOS technology comes in its ability to three-dimensionally (3D) reconstruct the spine and assess the axial plane, without the radiation dose of a CT scan or the loss of the impact of gravity seen in recumbent imaging. 51 , 52 EOS 3D reconstruction can also be used postoperatively to assess direct vertebral rotation (DVR), a technique employed to improve axial correction in spinal deformity (Fig. 4‑9). 53

When EOS imaging is not possible or available, traditional techniques to decrease radiation dose to radiosensitive tissues must be followed: PA radiographs, gonadal shields that protect the gonads but visualize the triradiate cartilage, posterior breast shields, and, most importantly, limiting radiographic testing unless truly necessary. Another technique to decrease radiation scatter includes utilizing an antiscatter grid, which absorbs scattered radiation and may even improve the quality of the bone imaging. Beyond EOS imaging, educating the community referring to the spinal deformity surgeon can limit the amount of inadequate and unnecessary radiographic studies performed. 54 The advent of digital radiography and electronic medical records allow for improved physician-to-physician communication, limits repeat imaging studies, and even improves the quality of the images by being able to change imaging intensity on the screen. Digital radiographs were found to be similar in efficacy to plain radiographs when assessed for ability to measure Cobb angles which further supports the transition to digital imaging and EOS technology when treating pediatric spinal deformity patients. 55
Computed tomography (CT) scans have a limited role in preoperative evaluation of patients with idiopathic scoliosis. When working up a patient with congenital scoliosis, rib anomalies, Marfan syndrome, neurofibromatosis, or Larsen syndrome, it provides additional information regarding fused segments and pedicle size and helps with surgical fixation assessment for the deformity correction. Patients evaluated for spondylolysis can be imaged with a CT scan to identify the pars defect (Fig. 4‑10); however, recent literature and a recommendation from the Scoliosis Research Society Evidence-Based Medicine Committee indicate a trend toward using MRI as the advanced study of choice in this setting to limit ionizing radiation. 56 , 57 Although indications for CT scan use preoperatively remain limited, CT utility in the operating room for navigated (O-arm) or robot-guided instrumentation is increasing yet still in early stages of widespread adoption. 58 , 59 , 60 Intraoperative use provides the surgeon with the axial, sagittal, and coronal plane trajectories of each vertebra, assisting with pedicle screw insertion. Significant concern exists regarding intraoperative use of the O-arm given that the ionizing radiation dose was found to be higher than when using C-arm fluoroscopy (although this depends on fluoroscopy time). 61 , 62 , 63 However, the counterargument remains the perceived safety benefit and includes a theoretical decrease in instrumentation malposition, neurologic injury, and need for reoperation.

MRI technology is critical to the evaluation of patients with spinal deformity. Beyond its ability to detail the soft-tissue envelope and bone, an MRI helps assess for syringomyelia, Chiari malformation, tethered cord, and spinal dysraphism, whose collective incidence in AIS has been reported in the literature to vary from 2 to 28%. 64 , 65 , 66 , 67 , 68 , 69 Determining who should have a preoperative MRI is a difficult task when managing pediatric spinal deformity. Classic teaching suggests that the indications for MRI include true hyperkyphosis, left thoracic curves, abnormal curve patterns, back pain, abnormal neurologic exams, and patients presenting before the age of 10 years. Some newer studies report not using hyperkyphosis as a potential indicator for MRI; however, an adequately powered study has yet to be performed to identify radiographic risk factors for neural axis abnormalities in patients with scoliosis. 69 , 70 Given the data supporting a rate of approximately 1:5 patients with juvenile/infantile idiopathic scoliosis having a neuroaxis anomaly, MRI should be considered part of the routine evaluation. 71 , 72 Research supporting MRI in all idiopathic scoliosis, regardless of age, sex, curve type, or clinical exam, has been published arguing that it not only is informative of the neuroaxis but also aids in preoperative planning. 73 Thus, the debate persists whether to have every patient with AIS undergo MRI prior to surgical intervention, as this is not cost-effective, but it can help identify neuroaxis pathology that can complicate and increase the risk of spinal deformity correction. 74 Diagnosing the intraspinal pathology prior to surgical intervention allows for the consultation of a neurologist or neurosurgeon for imaging review as well as the potential need for intervention. Neuromonitoring and neurologic complications increase with deformity correction in the setting of a neuroaxis pathology. 69 An added benefit of the MRI is that it simultaneously assesses the spinal column as well as the kidneys, and no report exists documenting any additional renal anomalies on ultrasound that were not previously already seen by MRI. 75 Postoperatively, if a patient will need to continue to have MRI evaluations, implants that are more MRI compatible can be employed. Additionally, when concerned about infection, the MRI allows for the assessment of fluid collections, osteomyelitis, and epidural abscess and is the imaging modality of choice over a nuclear imaging study with its associated radiation.
Surface topography recently has gained traction as a potential tool to identify scoliosis and monitor scoliosis for progression. A study by Knott et al demonstrated the need for fewer radiographs when X-rays were used in conjunction with surface topography imaging in patients with curves less than 25 degrees. 76 The study also demonstrated a correlation between quantifications of spinal deformity with surface topography and surface scanning, suggesting utility as a radiation-free means of diagnosing and monitoring spinal deformity.
Radiographic measurements: John Cobb in 1948 described a technique to measure the magnitude of frontal plane deformity in scoliosis. He described using the end plates to draw parallel lines of the upper and lower most involved vertebrae when comparing to the horizontal and measuring the angle between them (Fig. 4‑11). New technology allows for Cobb angle measurements to be made on the computer with a 3- to 5-degree measurement error, similar to that reproducibility from plain X-rays. 77 , 78 The Cobb angle concept applies to the sagittal plane to assess lordosis of the lumbar spine or kyphosis in the thoracic spine. EOS imaging does not preclude one from reliably measuring Cobb angles, even at the newest “microdose” protocol level. 50

Balance, in both the coronal and sagittal plane, is a key component when it comes to managing spinal deformity. The SRS defines coronal balance as the relationship of the C7 plumb line (C7PL), a vertical line from the center of the body of the seventh cervical vertebra, and the center sacral vertical line (CSVL), a vertical line bisecting the sacrum. If the distance between the two lines is less than 2 cm (negative value denotes C7PL to the left of CSVL), then the patient is considered balanced in the coronal plane (Fig. 4‑12). In the sagittal plane, balance is assessed by the relationship between a vertical line from the body of the seventh cervical vertebra and the posterior superior corner of the sacrum. A positive sagittal balance is defined as a distance greater than 2 cm from the posterior superior corner of the sacrum and the anterior C7 vertical plumb line. Until recently, the spinal deformity community believed that the sagittal plane did not play a role in clinical outcomes in AIS. 79 We cannot simply transpose the recommendations for the sagittal plane that we have from the adult spinal deformity literature, as we have minimal healthy pediatric control data. 80 Balancing thoracic kyphosis with lumbar lordosis remains key, as does not ending a fusion distal to a hyperkyphotic segment in the upper thoracic spine. 79 The most common sagittal plane abnormality remains thoracic lordosis or thoracic hypokyphosis (Fig. 4‑12). Surgical techniques that lengthen the posterior column and shorten the anterior column restoring thoracic kyphosis and thus lumbar lordosis must be employed in addition to correcting the coronal plane deformity in AIS. 81 Along with sagittal plane restoration, research is currently ongoing to identify the role of spinopelvic alignment in pediatric spinal deformity given its significant role in the adult population. 82 , 83

Scoliosis is a 3D deformity with rotation in the axial plane in addition to the coronal and sagittal planes, but this is extremely difficult to quantify on plain X-ray. Classic Nash and Moe grading and the Perdriolle method for rotational assessment are being replaced by EOS technology because of its ability to quantify spinal deformity in the axial plane for each vertebra. 47 , 84 , 85 , 86 CT-based methods historically provided more information regarding rotation than the Nash–Moe and Perdriolle methods but come at the expense of radiation exposure and are also obtained in a supine position. With the advent of slot scanning technology, CT scans are of limited use in pediatric spinal deformity. Slot scanning technology uses simultaneous biplanar image acquisition to provide the surgeon not only with frontal and lateral radiographs but also axial plane reconstruction of the entire spine. 3D EOS reconstruction can also be performed postoperatively for the evaluation of apical vertebral rotation correction, an important factor for successful outcomes. 84 One argument for CT technology as a part of the preoperative evaluation in pediatric spinal deformity is the success of recent patient-specific 3D-printed guides to assist in instrumentation for complex pathology. 87 Computer algorithms reconstruct and print a 3D model based on the CT images, allowing for surgical guides to be manufactured, sterilized, and attached to the patient’s spine intraoperatively following exposure to direct pedicle screw instrumentation.
The Lenke classification system has become the language with which spine surgeons communicate, using it to discuss curve type, surgical approach, fusion levels, surgical outcomes, and many other aspects of spinal deformity care (Fig. 6‑3 in this book). 88 In brief, the curve types include (1) main thoracic, (2) double thoracic, (3) double major, (4) triple major, (5) thoracolumbar/lumbar, and (6) thoracolumbar/lumbar–main thoracic with a sagittal plane (–, N, +) and lumbar spine (A, B, C) modifier, and are detailed in Chapter 6. Recognizing curve patterns that do not fit this classification system helps identify patients who need an additional workup for neuroaxis anomalies.

When reviewing a spine X-ray prior to surgical intervention, careful documentation of the number of rib-bearing vertebrae and the number of lumbar vertebral bodies is critical for safe and accurate preoperative planning.
4.5 Assessment of Skeletal Maturity
Pediatric spinal deformity worsens as the child grows; thus, understanding where the patient is along the growth continuum is critical to the treatment algorithm employed by the surgeon. Recently, numerous growth centers and classification systems have been created to best classify PHV, as this time period is critical for spinal deformity progression. Classically, the iliac apophysis (Risser sign), triradiate cartilage (open vs. closed), and bones of the hand (Greulich and Pyle) were used to determine skeletal maturity. Newer techniques evaluating the thumb, hand/wrist, ulna, and humeral head have all been described. 11 , 37
The Risser sign was first described in 1958 by Joseph C. Risser as he observed the start of ossification of the iliac apophysis correlating with the time of spinal maturation. 89 His classification system composes 5 grades: 0, no apophyseal ossification; 1, lateral 25%; 2, lateral 50%; 3, lateral 75%; 4, 100% apophyseal ossification; and 5, fusion of apophysis to the ileum (Fig. 4‑13). This system is convenient, as it is easily identifiable on a PA scoliosis screening X-ray. In 1984, Lonstein and Carlson 90 correlated curve progression with the Risser sign, Cobb angle, and chronological age, linking spinal deformity with skeletal maturity. Limitations do exist given that the PA view results in poorer image quality at the apophysis compared to an AP; significant pelvic tilt can distort the apophyses; and, most importantly, that PHV occurs prior to grade 1. 91 In girls, it takes approximately 1 year to progress from Risser 0 to Risser 4 and, at that point, little skeletal maturity remains; while in boys this process may take longer, and significant amounts of growth may remain beyond Risser 4. 92

The triradiate cartilage can also be evaluated on the AP EOS or a conventional full-length standing spine X-ray (Fig. 4‑12). This cartilaginous component of the pelvis ossifies at various time points by gender: in females usually around the onset of menarche while in boys at the beginning of puberty. It can be used as a marker of skeletal immaturity while still open. Surgeons historically worry less about the crankshaft phenomenon when performing posterior spinal fusion surgery in adolescents whose triradiate cartilage is closed, as this is often an indicator of being beyond PHV. 93 Some surgeons use the timing of the olecranon apophysis fusing as a marker of the end of growth after triradiate closure. 94
Immense study on the ossification of hand and wrist has resulted in our ability to correlate the degree of skeletal maturity well with radiographic images of the distal upper extremity. Greulich and Pyle cataloged this process in their book Radiographic Atlas of Skeletal Development of the Hand and Wrist. The Tanner–Whitehouse method evaluates the individual bones of the finger but is quite tedious to use in a practical clinical setting. In the day of advanced analytics, we can hopefully look forward to an electronic EOS image being scanned and matched to an X-ray from the Greulich and Pyle atlas to get an immediate exact skeletal age. Sanders in 2008 compacted the hand/wrist ossification progression into eight stages that are easily identifiable, have excellent intraobserver reliability, and highly correlate with scoliosis behavior (Fig. 4‑14). 95 The stages are as follows: (1) Juvenile slow (digital epiphysis not covered); (2) preadolescent slow (all digital epiphysis covered); (3) adolescent rapid early (digit capping with 2–5 metacarpal epiphyses wider than metaphyses); (4) adolescent rapid late (any distal phalangeal physis closure beginning); (5) adolescent steady early (all distal phalangeal physes closed); (6) adolescent steady late (middle or proximal phalangeal physeal closure begins); (7) early mature (distal radial physis open only with metaphyseal scars present); and (8) mature (distal radius physis closed). 95 Beyond Sanders, other classifications have been created, tested, and validated including a distal radius and ulnar (DRU) classification by Keith Luk, a humeral head (HH) classification by Brian Smith, and a thumb ossification composite index (TOCI) by Jack Cheng. 37 , 96 , 97 , 98 , 99 Conversations about treatment recommendations should pool all available information on skeletal age when counseling patients and parents with pediatric spinal deformity, as clearly we have yet to identify one best system (Fig. 4‑15).

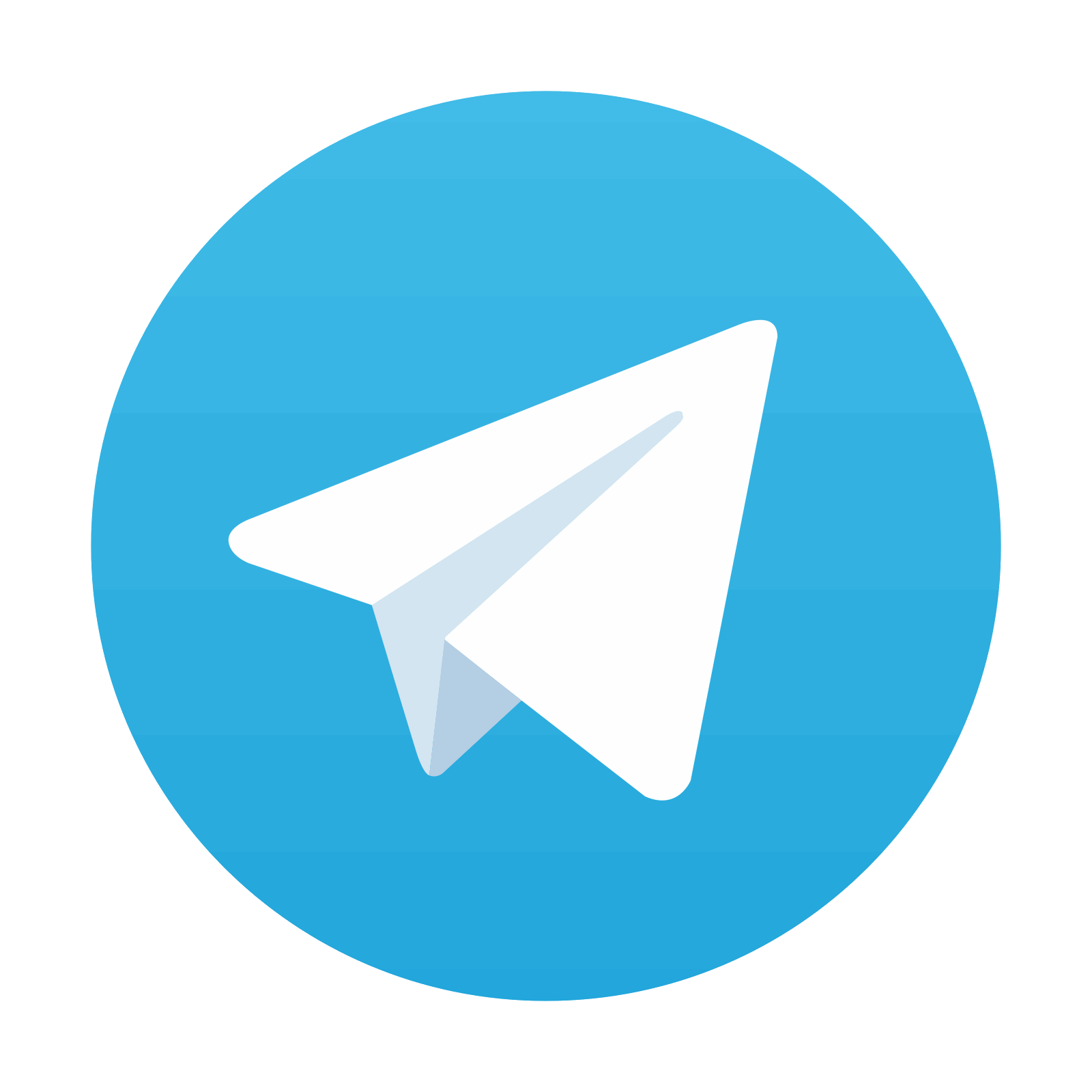
Stay updated, free articles. Join our Telegram channel

Full access? Get Clinical Tree
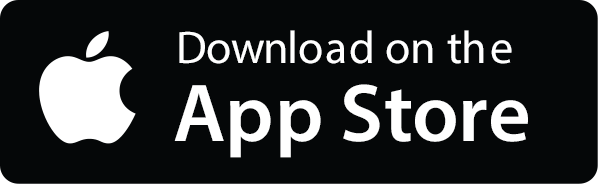
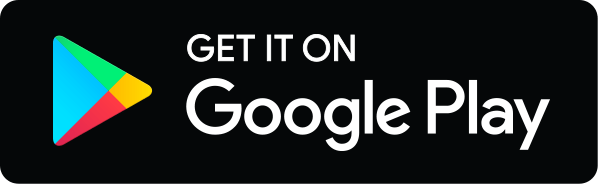