and Peter J. Millett2
(1)
Steadman Philippon Research Institute, Vail, CO, USA
(2)
The Steadman Clinic, Steadman Philippon Research Institute, Vail, CO, USA
Keywords
ShoulderRotator cuffImpingementAcromionPhysical examClinical exam4.1 Introduction
Rotator cuff disease ranks among the most common musculoskeletal disorders to be encountered in clinical practice. As a result, we have witnessed a rapid evolution in diagnostic methods and treatment options for the entire spectrum of rotator cuff disorders over the past few decades.
The derangement of normal anatomy and subsequent rotator cuff impingement is often cited as the primary cause for rotator cuff disease. However, the undersurface of the coracoacromial arch may not always be the culprit in this complex array of syndromes. Traumatic events, repetitive microtrauma, and glenohumeral instability may also be causative in a large proportion of patients. These factors, among others, are important to consider when evaluating the patient with a suspected rotator cuff lesion.
Therefore, proficiency in the physical diagnosis of various rotator cuff lesions requires a solid differential diagnosis, an appreciation of normal anatomy and biomechanics and the awareness that surrounding structures involved with normal function may also contribute to pathologic conditions. This is important not only for the initial examination by the treating physician, but also for the teams of individuals who care for these patients.
4.2 Anatomy and Biomechanics
The rotator cuff is often conceptualized as being composed of four separate muscles, tendons, and insertion sites that each has its designated functions. However, in reality, although each muscle belly arises from different areas of the scapular body, their tendons converge and coalesce to form a single, continuous tendon sheet that inserts upon the greater and lesser tuberosities of the proximal humerus (Fig. 4.1). This structural configuration suggests that the individual muscles of the rotator cuff work simultaneously and in synchrony to achieve its primary function—to dynamically stabilize and compress the humeral head within the glenoid fossa [2].
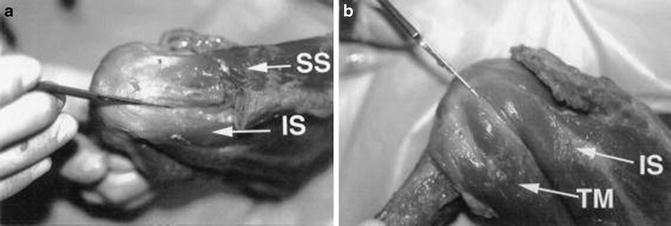
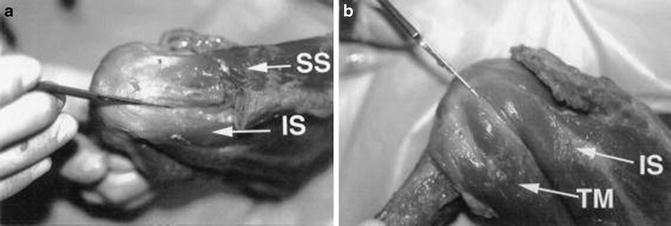
Fig. 4.1
Cadaveric dissection photographs demonstrating the coalescence of the rotator cuff tendons as they approach their respective insertion sites on the humerus. (a) View from posterosuperiorly showing the approximate interval between the supraspinatus (SS) and the infraspinatus (IS). (b) View from posteriorly showing the approximately interval between the infraspinatus (IS) and teres minor (TM). (From Dugas et al. [1]; with permission).
Maintenance of a stable fulcrum requires balanced axial and coronal plane force couples (Fig. 4.2) [3, 4]. This concept, initially developed by Burkhart [4] in 1991, is produced by the strategic anatomic positioning of the muscles around the shoulder. Specifically, the combined actions of the anterior cuff (i.e., the subscapularis) and the posterior cuff (i.e., the infraspinatus) work to compress the humeral head within the glenoid fossa due to their parallel force vectors in the axial plane. In the coronal plane, contraction of each rotator cuff muscle and the deltoid muscle also generates a net force vector that drives the humeral head medially against the glenoid. Disruption of any of these force couples, as in the case of a rotator cuff tear or deltoid weakness, can produce disordered shoulder function through a variety of mechanisms. This concept led to the biomechanical principle of concavity compression, described by Lippitt and Matsen [5] in 1993, in which the balanced, parallel force couples generated by the rotator cuff and deltoid compress the convex humeral head into the concave glenoid fossa thereby enhancing glenohumeral stability in the mid-ranges of motion. In addition to providing a stable fulcrum for motion, balanced force couples (with resulting concavity compression) improve glenohumeral stability by increasing the force and degree of humeral angulation required for the humeral head to translate over the glenoid rim in any direction (i.e., an increased balance stability angle, as discussed in Chap. 6). It is easy to imagine that disruption of axial or coronal plane force couples would result in dysfunction of the concavity compression mechanism leading to scapular dyskinesis and subsequent shoulder discomfort (see Chap. 9 for more information on scapular dyskinesis and its relationship with rotator cuff tears).
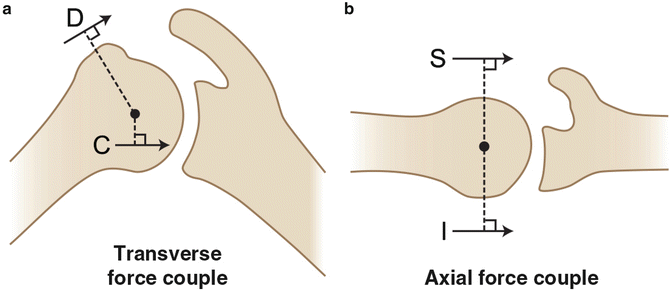
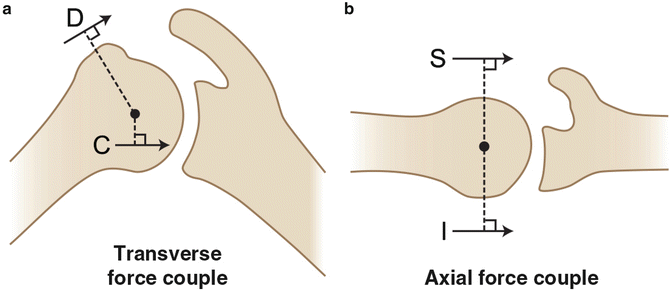
Fig. 4.2
Illustration highlighting the important force couples that help maintain concavity compression and overall glenohumeral stability. (a) The combined actions of the deltoid muscle (D) and the rotator cuff (C) make up the transverse plane force couple and pull the humeral head medially towards the glenoid fossa. (b) The combined actions of the subscapularis (S) and the infraspinatus (I) make up the axial plane force couple and also work to drive the humeral head medially towards the glenoid fossa.
The rotator cable, described by Burkhart [3] in 1993 as a part of the “suspension bridge model,” is a thickened area of tendon that extends across the supraspinatus and infraspinatus tendons which biomechanically allows their respective forces of contraction to disperse along the length of the cable, eventually concentrating at its anterior and posterior insertion sites (Fig. 4.3). The rotator cable surrounds a crescent-shaped area of tendon (i.e., the rotator crescent) that is somewhat protected from the strong forces produced by the supraspinatus and infraspinatus tendons as a result of the function of the rotator cable. The force couple principle in combination with the function of the rotator cable may provide an explanation as to why some patients are able to maintain adequate shoulder function despite the presence of a large full-thickness supraspinatus tear. However, recent evidence suggests that the load-sharing capability of the rotator cuff is diminished in the presence of a partial- or full-thickness tear which subsequently promotes tear extension [6, 7]. In other words, the defect in the cuff tendon decreases the available area required to disperse normal tensile forces produced by muscle contraction. Because these normal contraction forces must be transmitted (and re-directed) through a smaller area of intact tendon, the magnitude of stress concentration along the margins of the cuff tear increases exponentially as the size of the tear increases.
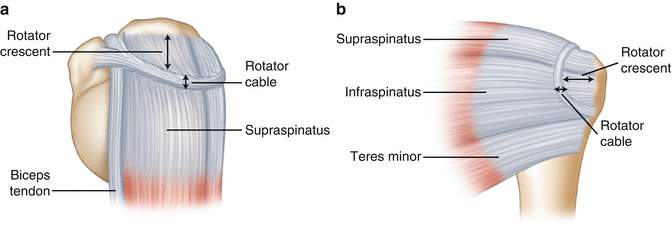
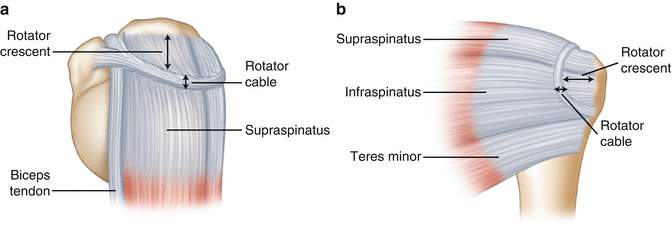
Fig. 4.3
(a) Superior and (b) posterior view illustrating the position of the rotator cable. The rotator cable is a thickened area of the rotator cuff that provides a path for force dispersion which helps to prevent tension overload within the rotator crescent (the area of tendon surrounded by the rotator cable).
Anterior or posterior extension of a rotator cuff tear can also occur as a result of the disruption of balanced force couples. A study by Hughes and An [8] found that normal supraspinatus tendons exerted a maximum force of approximately 175 N whereas normal infraspinatus tendons exerted a maximum force of greater than 900 N. This has important implications for the development and progression of rotator cuff tears—posterior extension of a tear into the infraspinatus tendon dramatically increases the force applied to the remaining intact tendon sheet which can accelerate tear progression. Because the force exerted by the infraspinatus must be similar to that of the subscapularis to maintain balanced force couples, this concept of tear extension can also be applied anteriorly into the subscapularis muscle. Thus, anterior or posterior extension of a rotator cuff tear into the subscapularis and/or the infraspinatus tendons, respectively, disrupts the balance of native force couples which also accelerates tear progression. Longitudinal (or medial) tear extension of the supraspinatus with or without retraction can also disrupt glenohumeral kinematics; however, the pathomechanism typically involves proximal humeral head migration, highlighting the importance of the rotator cuff as a dynamic depressor of the humeral head (Fig. 4.4).
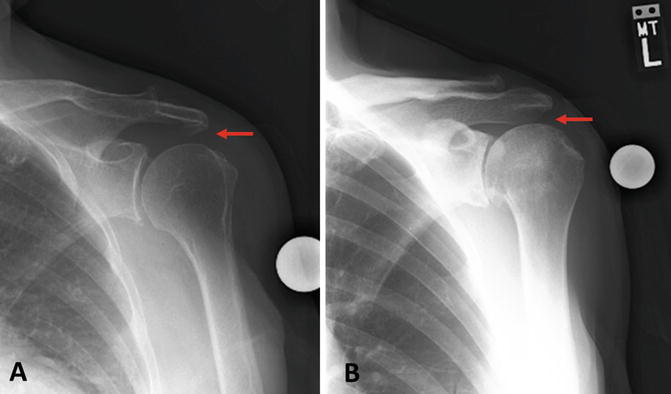
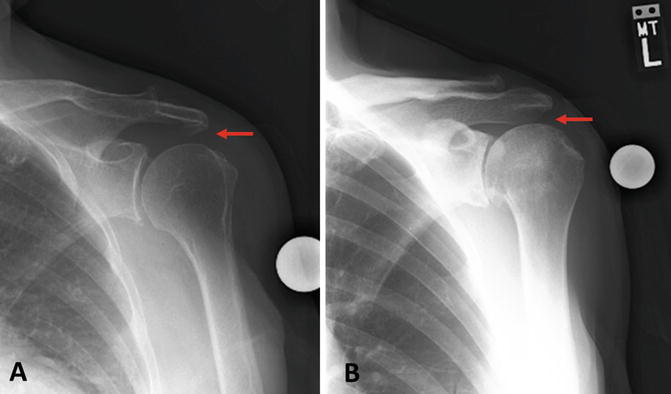
Fig. 4.4
(a) Anteroposterior (AP) radiograph demonstrating a normal acromiohumeral distance (red arrow) in a patient with an intact rotator cuff. (b) AP radiograph of a patient with a massive rotator cuff tear. Proximal migration of the humerus and a subsequent decrease in the acromiohumeral distance (red arrow) can be seen.
4.3 Subacromial Impingement
Subacromial impingement is one of the most common causes of shoulder pain encountered in clinical practice. In the past, some authors believed impingement was the result of extrinsic factors, citing various potential sources of external cuff compression [9–11]. Others believed the disorder was related to intrinsic cuff degeneration, leading to cuff weakness and proximal humeral migration followed by cuff abrasion under the acromion [12]. However, recent thinking suggests that subacromial impingement is likely multifactorial involving a combination of both intrinsic and extrinsic factors that ultimately lead to rotator cuff disease.
4.3.1 Pathogenesis Involving Extrinsic Factors
Neer [10] originally described subacromial impingement as the repeated contact between the greater tuberosity and the undersurface of the acromion and coracoacromial ligament (Figs. 4.5 and 4.6). He hypothesized that this repetitive mechanical impingement led to the development of proliferative anterolateral acromial spurs. He subsequently dissected 100 cadaveric shoulders and again revealed these traction spurs on the anterolateral acromion. With this finding, he proposed that anterior acromioplasty should be performed to prevent impingement and subsequent bursitis and rotator cuff disease. Later, realizing that subacromial impingement likely involves a continuum of disease processes, Neer [10] described three basic stages in the development of impingement syndrome. Stage I of impingement, occurring asymptomatically in patients younger than 25 years of age, involves subacromial edema, hemorrhage, and bursitis. Between the ages of 25 and 40, continued impingement results in rotator cuff fibrosis and tendinitis, eliminating the normal lubricating effect of the subacromial bursa. Beyond the age of 40 years, continued impingement becomes more symptomatic with the development of acromial spurs along with partial- and full-thickness rotator cuff tears.
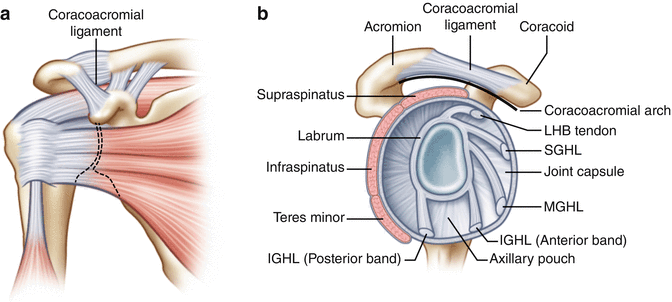
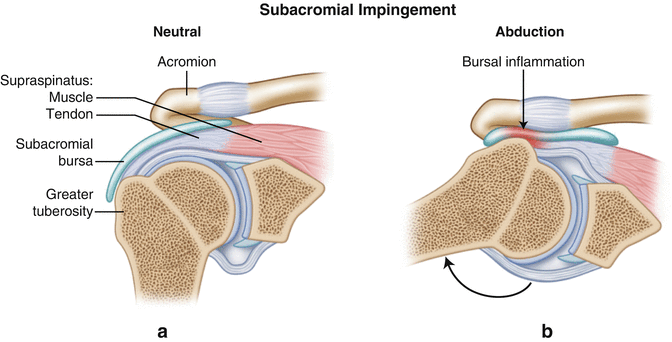
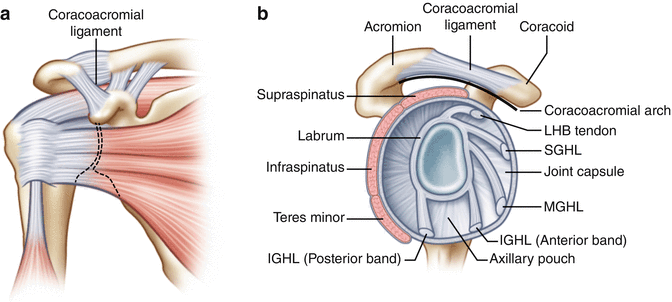
Fig. 4.5
(a) Anterior view of the coracoacromial ligament with the rotator cuff musculature passing closely beneath. (b) Sagittal view of the coracoacromial arch which is made up of the anterolateral acromion, coracoacromial ligament, and the posterior aspect of the coracoid. With the humeral head removed, the rotator cuff musculature can be seen traveling closely beneath the coracoacromial arch.
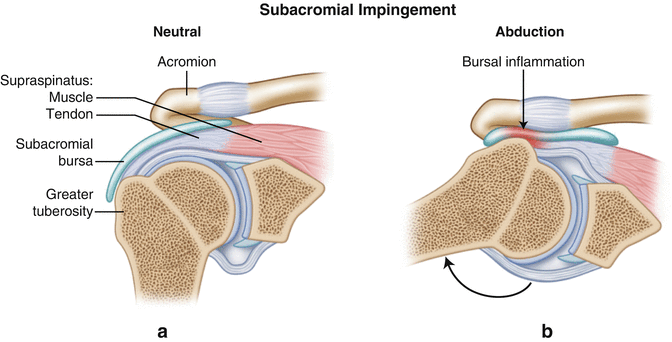
Fig. 4.6
(a) Anteroposterior (AP) cross-section view of the shoulder illustrating the position of the supraspinatus muscle-tendon unit and the subacromial bursa relative to the inferior acromion when the humerus is in a neutral resting position. (b) When the humerus is elevated, the supraspinatus and subacromial bursa can make contact with the undersurface of the acromion, often resulting in rotator cuff pathology with impingement-like symptoms.
Neer’s description of the stages of impingement syndrome is one of the most popular pathomechanistic explanations behind the development of chronic rotator cuff disease. While there are several studies that support this mechanism [11, 13, 14], the precise etiology and location of subacromial impingement is debatable.
4.3.1.1 Coracoacromial Ligament
The coracoacromial ligament originates from the distal-lateral extension of the coracoid process and travels posterosuperiorly to insert upon the anterolateral margin of the acromion [15, 16]. As part of the coracoacromial arch, this ligament is commonly described as being involved with rotator cuff impingement lesions due to the proximity of the cuff tendons that pass closely beneath, especially as the arm is elevated. The coracoacromial ligament has a number of anatomic variations [17–19]; however, only those variations that involve a distinct anterolateral and posteromedial bundle are likely to be related to rotator cuff impingement and subsequent tearing. In a cadaveric study, Fremery et al. [20] found that shoulders with rotator cuff tears and clinical evidence of impingement had stronger, thicker anterolateral bands compared to shoulders with unrelated issues. Evidence of traction spur formation within the anterolateral band has also been found which further implicates its involvement with the development of impingement [21]. Chambler et al. [22] suggested that arm abduction results in increased tension of the coracoacromial ligament which may provide an explanation for the development of traction spurs in these patients. A more recent cadaveric study by Yamamoto et al. [23] found that the superior cuff made contact with and, in fact, generated increased tension through the coracoacromial ligament during range of motion testing in a series of normal, healthy shoulders. This finding may provide at least one possible explanation behind the development of traction-type spurs on the anterolateral acromion with advancing age, potentially leading to extrinsic compression of the superior cuff tendons. However, whether or not the thickness of the anterolateral band is a cause or effect of rotator cuff disease has not been elucidated.
4.3.1.2 Os Acromiale
The acromion is also subject to developmental abnormalities as a result of failed fusion of secondary ossification centers. This failed fusion results in a defect known as an “os acromiale” and occurs in approximately 8 % of the population where 1/3 of these individuals are affected bilaterally [24]. Os acromiale is a mobile accessory ossicle that, when unstable and pulled inferiorly by contraction of the deltoid with arm elevation, has been associated with the development of identifiable impingement lesions and pain at the top of the shoulder (Fig. 4.7). However, this relationship has been refuted on several occasions [25–29]. In addition, surgical treatment strategies for os acromiale that involve increasing the volume of the subacromial space has not resulted in an improvement in clinical outcomes [26]. Further study is therefore needed to clarify the effects of os acromiale on normal rotator cuff tendons.
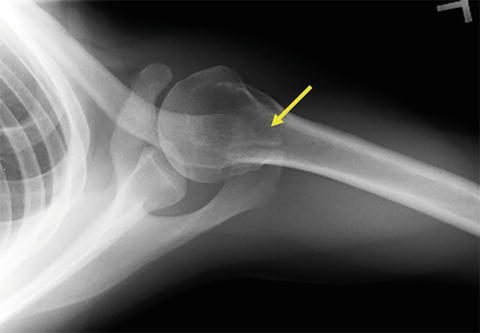
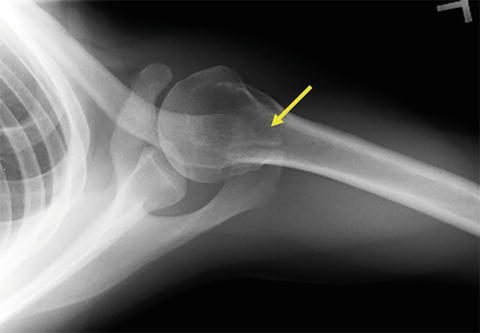
Fig. 4.7
Axillary radiograph demonstrating os acromiale (yellow arrow). This patient presented to our clinic with impingement-like symptoms.
4.3.1.3 Acromial Morphology and Glenoid Version
The anterior aspect of the acromion may, in itself, be a potential site of rotator cuff abrasion and subsequent tearing regardless of the presence or absence of space-occupying traction spurs associated with the coracoacromial ligament. In 1991, Bigliani et al. [30] described the three most common acromial morphologies as flat (type I), curved (type II), and hooked (type III), citing the hooked acromion as most relevant to the development of subacromial impingement (Fig. 4.8). In a cadaveric study, Flatow et al. [13] demonstrated that the type III acromion has an increased propensity to make contact with the rotator cuff tendons when compared to the other acromial morphologies that were previously described by Bigliani et al. [30]. Wang et al. [31] found that acromial morphology influenced the success of conservative management for rotator cuff tears. In their study, the majority of patients with either a type I or II acromion responded favorably to conservative management. In contrast, more than half of those patients with a type III acromion failed nonoperative treatment and required subsequent surgical intervention. Unfortunately, these authors did not report their findings at the time of surgery. In another study, Gill et al. [14] found that acromial morphology was an independent factor associated with the development of rotator cuff pathology using multivariate logistic regression analysis. Additionally, Natsis et al. [32] found a statistically significant increase in the rate of anterolateral acromial spur formation in those with a type III acromion. They concluded that a type III acromion with anterolateral spur formation was contributing factor associated with the development of rotator cuff impingement and tearing. In 2012, Hamid et al. [33] arrived at similar conclusions. However, despite these results, there are also a number of studies that refute the oft-cited correlation between the type III acromion and the development or progression of rotator cuff tears [17, 31, 34–38]. As a result of this conflicting data, further study is needed to determine if acromial morphology, as described by Bigliani et al [30], is truly associated with the development of symptomatic subacromial impingement and rotator cuff tears.
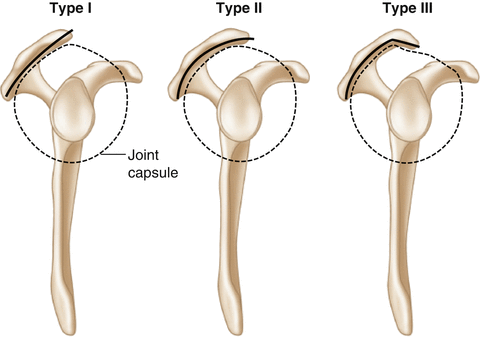
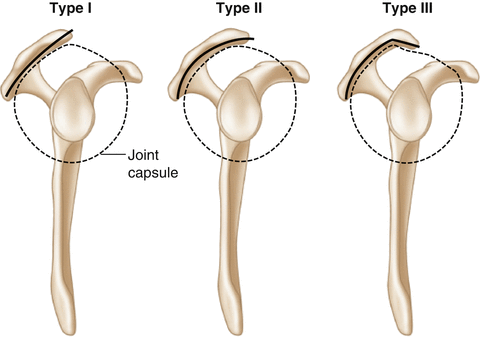
Fig. 4.8
Illustration of the three types of acromial morphologies as described by Bigliani et al. [30]. Type I: Flat acromion. Type II: Curved acromion. Type III: Hooked acromion. Patients with a type III hooked acromion may have an increased propensity to develop subacromial impingement and rotator cuff disease.
More recently, other acromial morphologies have been also been described—namely, the convex acromion (type IV; as described by Vanarthos and Monu [39] as an addendum to the original classification system developed by Bigliani et al. [30] Fig. 4.9) and the keeled acromion (originally described by Tucker and Snyder [41] Fig. 4.10). While the type IV acromion has not been implicated as an anatomic factor associated with rotator cuff disease, the keeled acromion may be involved to some extent. The keeled acromion, which was described as a central spur (or convexity) located on the undersurface of the acromion, was significantly associated with the presence of both partial- (bursal-sided) and full-thickness rotator cuff tears in the original study published by Tucker and Snyder [41]. Several recent studies have also suggested that a steep acromial slope may be another factor associated with the development of impingement lesions [34, 42–44]; however, further study needs to be conducted to substantiate these claims.
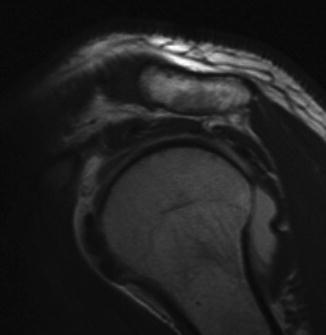
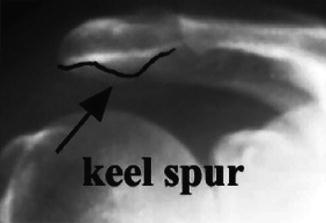
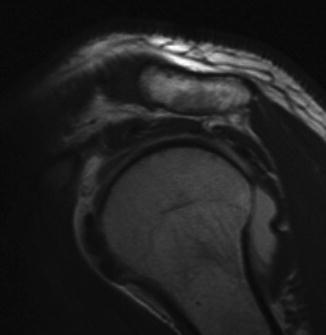
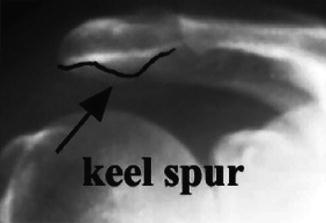
Fig. 4.10
Anteroposterior (AP) radiograph demonstrating a keeled acromion as described by Tucker and Snyder [41]. This acromial morphology may have some involvement in the development of subacromial impingement, although further study is needed to substantiate this claim (From Tucker and Snyder [41]; with permission).
Excessive lateral extension of the acromion, which is best quantified through calculation of the acromial index (Fig. 4.11), has also been reported as a potential contributor to the development of rotator cuff impingement and tearing. Some investigators report that decreased coverage of the humeral head by the acromion (i.e., a decreased acromial index) may allow the humeral head to utilize the anterolateral acromion as a fulcrum or lever to aid in glenohumeral elevation, possibly causing abrasion of the cuff tendons and subsequent rotator cuff tearing [45, 46]. Nyffeler et al. [47] suggested that a large acromial index may result in a more superiorly directed force vector produced by the middle fibers of the deltoid, potentially leading to superior migration of the humeral head which, in turn, decreases the available space for the cuff tendons to pass beneath the acromion. This theory has been partially validated since other more recent studies have found statistically significant associations between increased acromial indices and the presence of rotator cuff tears [31, 33, 34, 47–50]. Although the acromial index appears to play some role in the development of rotator cuff disease, additional studies are needed to fully elucidate the exact pathomechanisms behind this phenomenon.
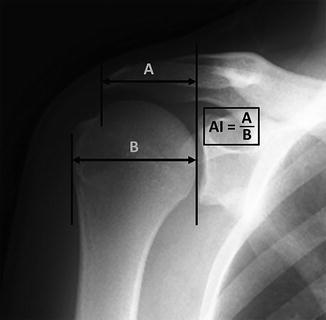
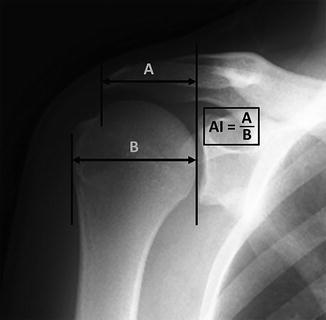
Fig. 4.11
True anteroposterior (AP) radiograph demonstrating measurement of the acromial index. A first line is drawn connecting the superior and inferior rims of the glenoid and extended superiorly such that the line completely crosses the acromion. A second line is drawn vertically that corresponds with the most lateral extent of the acromion. The distance between the first line and the second line is labeled “A.” A third line is drawn vertically that corresponds with the most lateral extent of the greater tuberosity. The distance between this third line and the first line is labeled “B.” The ratio of A/B is equal to the acromial index. Theories exist that rationalize both increased and decreased acromial indices with rotator cuff disease; however, further study is needed to elucidate the precise role of the acromion in the development of rotator cuff disease.
Inclination of the glenoid in the coronal plane has also been associated with the development of rotator cuff tears on several occasions [51–53]. Tétrault et al. [52] performed measurements using magnetic resonance imaging (MRI) to determine the orientation of the supraspinatus muscle fibers relative to the glenoid surface. In that study, the mean angle formed between the supraspinatus tendon and the glenoid surface was approximately 80° in the coronal plane. The authors suggested that a decrease in this angle (i.e., increased upward tilt of the glenoid) may result in a more vertically oriented force vector produced by the supraspinatus, thus preferentially pulling the humeral head superiorly [52]. If this theoretical mechanism is factually correct, the supraspinatus tendon could then make contact with the acromion, possibly leading to the cascade of events commonly associated with rotator cuff disease. A similar mechanism may occur when considering glenoid anteversion and retroversion in which tearing of the subscapularis and infraspinatus is observed, respectively [52]. Although at least one study found that surgically decreasing the glenoid inclination angle may decrease the measured amount of superior humeral head translation with passive abduction [49], none of the more recent imaging studies have shown significant associations between any type or degree of glenoid version and the presence of rotator cuff lesions, regardless of location of the tear or the tendon involved [54, 55].
The critical shoulder angle (CSA) (recently described by Gerber et al. [56] and Moor et al. [57, 58]) is another radiographic measurement purported to have an association with rotator cuff tears or osteoarthritis. The CSA is obtained from true anteroposterior (AP) radiographs and takes into account both the acromial index and the degree of glenoid inclination in the scapular plane (Fig. 4.12). The original developers of this measurement have suggested that the CSA may have an ability to predict the future development of degenerative rotator cuff tears (when the CSA > 35°) and glenohumeral osteoarthritis (when the CSA < 30°). However, a well-designed prospective study would be needed to confirm these claims given the current lack of conclusive clinical data suggesting any association between either the acromial index or glenoid inclination and any shoulder pathology.
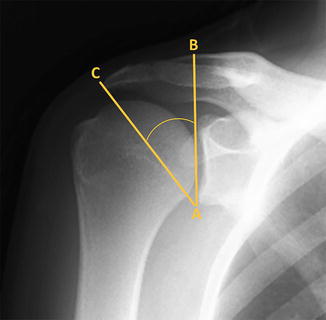
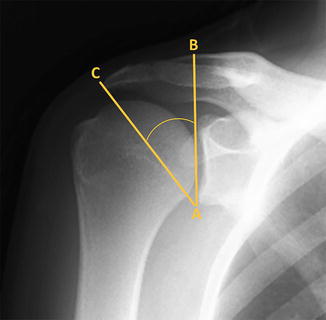
Fig. 4.12
True anteroposterior (AP) radiograph demonstrating measurement of the critical shoulder angle. Line “AB” is first drawn, which connects the most lateral points of the superior and inferior glenoid rim. Line “AC” is then drawn, which connects the previously drawn point “A” to the most lateral point of the acromion (designated as point “C”). The angle formed between lines “AB” and “AC” represents the critical shoulder angle. According to Moor et al. [57] an angle of less than 30° increases the risk for osteoarthritis whereas an angle of greater than 35° increases the risk for degenerative rotator cuff tears.
4.3.2 Pathogenesis Involving Intrinsic Factors
While extrinsic factors probably have some role in the development of subacromial impingement, many authors believe that the initiation and progression of rotator cuff disease primarily occurs as a result of intrinsic cuff degeneration. They argue that degenerative changes and/or traumatic injuries weaken the contractile strength of supraspinatus muscle which predictably leads to superior humeral head migration and cuff impingement beneath the acromion with humeral elevation. Spurring of the anterolateral acromion and erosion of the greater tuberosity are then observed (due to repeated reciprocal contact) along with rotator cuff degeneration.
The deterioration of tendon quality due to advanced age is often implicated as one of the primary causes of rotator cuff weakness, potentially resulting in proximal humeral head migration, subsequent bursal irritation and cuff tendinopathy. While the incidence and severity of rotator cuff disease has been found to increase with age on several occasions, Ogata and Uhthoff [59] found that acromial osteophytes were not always present in older patients. Further, those who did have acromial osteophytes actually had articular-sided partial-thickness rotator cuff tears (as opposed to bursal-sided tears). However, a more recent study identified the presence of anterolateral acromial spurs as an independent risk factor for the development of rotator cuff disease [33]. Further research is needed to identify and elucidate the roles of mechanical compression and intrinsic tendon degeneration on the progression of rotator cuff disease.
The tenuous microvascular blood supply to the supraspinatus and infraspinatus tendons has also been suggested as a possible intrinsic factor related to the development and progression of certain rotator cuff tears [12, 35, 60–65]. Lohr and Uhthoff [12] identified an area along the edges of supraspinatus tears in which no vessels were present, suggesting that spontaneous healing of a torn rotator cuff tendon is probably not feasible without surgical intervention. Ling et al. [63] studied the vascular supply of the supraspinatus tendon in 22 adults using scanning electron microscopy. They described a hypovascular zone on the surface of the supraspinatus tendon, confirming the findings that had been reported by others (Fig. 4.13) [62, 64, 65]. More recently, Brooks et al. [35] found that both the supraspinatus and infraspinatus tendons were hypovascular in the most distal 15 mm of their respective insertion sites on the greater tuberosity. This area of the insertional footprint, termed the “critical zone” by Moseley and Goldie [64] in 1963, may have an increased propensity to develop partial- or full-thickness rotator cuff tears as a result of poor tendon nutrition and a limited capacity for spontaneous healing. Although several studies have revealed evidence of apoptosis and hypoxia in rotator cuff tendons with visible tears and impingement lesions [18, 66–68], some authors believe these findings are the result of cuff degeneration rather than the cause of cuff degeneration [69].
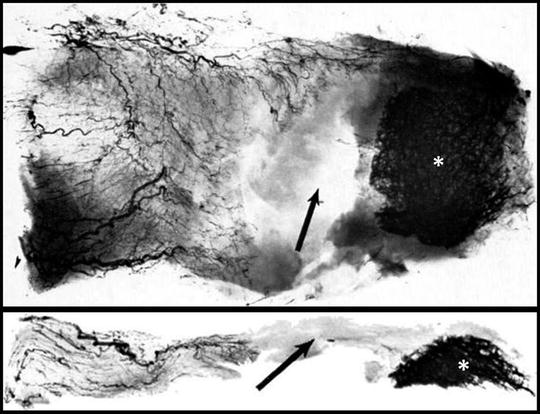
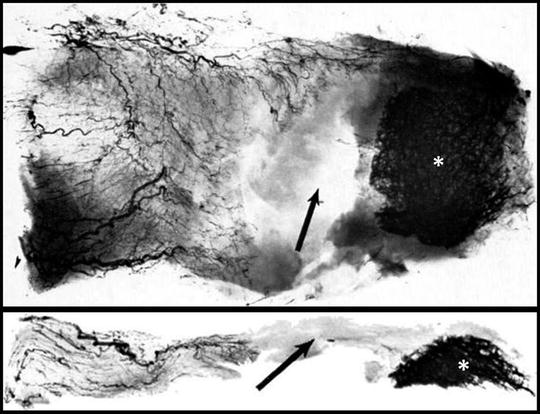
Fig. 4.13
(a) Axial slide showing the microvascular pattern of the supraspinatus tendon. (b) Coronal slide showing the microvascular pattern of the supraspinatus tendon. Arrows correspond to the region of avascularity and asterisks indicate the location of the supraspinatus footprint on the greater tuberosity. (From [65] with permission).
4.3.3 Physical Examination
Numerous studies have evaluated the utility of physical examination tests to reliably identify subacromial impingement. The Neer impingement sign [10], Hawkins–Kennedy test [70], and the painful arc sign [71] are the most useful and most widely utilized tests for the detection of subacromial impingement. Tenderness to palpation at the location of Codman’s point (described below) may also suggest rotator cuff impingement. Because pathologies other than impingement can produce impingement-like signs and symptoms during the physical examination, sensitivity and specificity data for these tests are variable [72–78]. Therefore, consideration of all available information, including other clinical tests and historical features, is necessary to synthesize an accurate physical diagnosis in every patient. When the diagnosis is in question, it is often useful to inject a local anesthetic into the subacromial space before repeating each test. This method is typically used to identify whether the patient’s subjective weakness is primarily due to guarding or due to actual muscle weakness. The relief of impingement-like signs and symptoms that were present before the injection usually confirms the diagnosis. This technique is usually referred to as the Neer impingement test which should not be confused with the Neer impingement sign (described below).
4.3.3.1 Neer Impingement Sign
The Neer impingement sign, first described by Neer [10] in 1972, is elicited by passive and maximal forward elevation of the humerus and stabilization of the scapula with the examiner’s contralateral hand (Fig. 4.14). Stabilization of the scapula is essential to maximize the utility of the test since upward rotation of the scapula (and therefore the acromion) with forward elevation will decrease the likelihood of reproducing cuff impingement under the acromion. Reproduction of the patient’s symptoms is indicative of a positive test. Several investigators have evaluated the clinical efficacy of the Neer impingement sign in its ability to accurately diagnose subacromial impingement (Table 4.1) [72, 73, 75, 76, 78, 80].
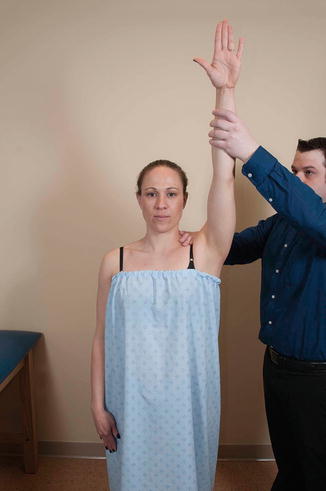
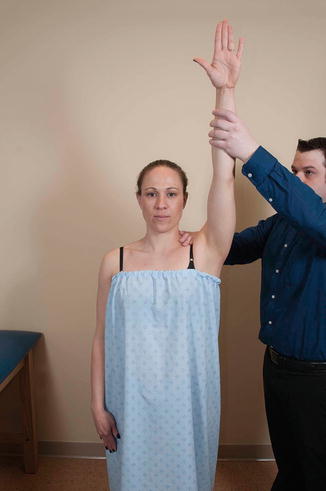
Fig. 4.14
Neer impingement sign. The examiner stabilizes the scapula with one hand and uses the other hand to passively forward-flex the humerus to a point of maximal elevation. Reproduction of the patient’s symptoms with this maneuver may suggest the presence of subacromial impingement.
Table 4.1
Diagnostic efficacy of the Neer impingement sign in isolation
Diagnostic efficacy of the Neer impingement sign in isolation | |||||||
---|---|---|---|---|---|---|---|
Investigators | Maneuver | Pathology | Standard | LR+ | LR− | Sensitivity (%) | Specificity (%) |
Silva et al. [78] | Neer | SIS | MRI | 0.98 | 1.1 | 68 | 30 |
Fodor et al. [73] | Neer | SIS | Ultrasound | 10.8 | 0.48 | 54 | 95 |
Michener et al. [76] | Neer | SIS | Arthroscopy | 1.76 | 0.35 | 81 | 54 |
Kelly et al. [79] | Neer | SIS | Ultrasound | 0.62 | 3.80 | 62 | 10 |
Chew et al. [72] | Neer | SIS | Ultrasound | 1.60 | 0.60 | 64 | 61 |
Toprak et al. [80] | Neer | SIS | Ultrasound | 1.67 | 0.38 | 80 | 52 |
Both Fodor et al. [73] and Kelly et al. [79] used ultrasonic evaluation to determine the sensitivity and specificity of the Neer sign in the diagnosis of subacromial impingement. Interestingly, although each study reported similar sensitivity values, their specificity values were divergent (95 % and 10 %, respectively). These results highlight the significant variability that may exist in the performance and interpretation of physical examination findings, specifically with regard to subacromial impingement syndrome. Nevertheless, Hegedus et al. [81] attempted to account for various confounding factors and study quality in a recent meta-analysis. In that study, the overall calculated sensitivity of the Neer impingement sign was 72 % while its overall specificity was approximately 60 %. Clearly, this maneuver is not adequate to diagnose subacromial impingement in isolation; however, combination of its results with those obtained from the Hawkins–Kennedy test and the painful arc sign (described below) are likely to improve diagnostic accuracy.
4.3.3.2 Hawkins–Kennedy Test
The Hawkins–Kennedy test [70] was first described in 1980; however, it was not originally thought to be as reliable or reproducible as the Neer impingement sign. A positive Hawkins–Kennedy test is the result of greater tuberosity contact on the undersurface of the coracoacromial ligament that is thought to reproduce symptoms related to subacromial impingement. To perform this test, the shoulder is brought to 90° of abduction in the scapular plane with the elbow also flexed 90°. From this position, the humerus is slowly and maximally internally rotated (Fig. 4.15). Reproduction of the patient’s symptoms (typically pain over the anterior shoulder) is deemed a positive test and may be indicative of superior cuff impingement. Numerous studies have evaluated the efficacy of the Hawkins–Kennedy test in its ability to diagnose subacromial impingement with variable results (Table 4.2) [72–76, 78, 80, 82]. The meta-analysis by Hegedus et al. [81] reported similar overall sensitivities and specificities for both the Neer impingement sign and the Hawkins–Kennedy tests.
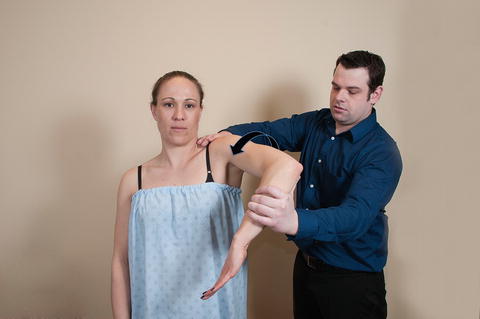
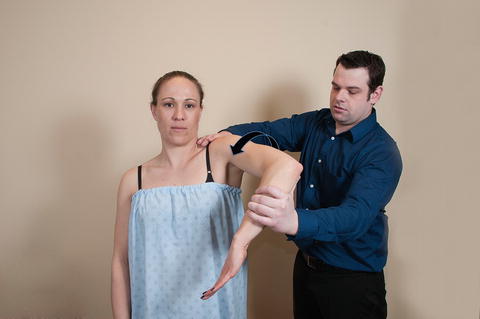
Fig. 4.15
Hawkins–Kennedy test. The examiner passively abducts the humerus in the scapular plane to approximately 90° with the elbow also flexed to 90°. The examiner then internally rotates the humerus which is thought to induce impingement between the greater tuberosity and the undersurface of the acromion. Reproduction of the patient’s symptoms with this test may be suggestive of subacromial impingement.
Table 4.2
Diagnostic efficacy of the Hawkins–Kennedy test in isolation
Diagnostic efficacy of the Hawkins–Kennedy test in isolation | |||||||
---|---|---|---|---|---|---|---|
Investigators | Maneuver | Pathology | Standard | LR+ | LR− | Sensitivity (%) | Specificity (%) |
Silva et al. [78] | H–K | SIS | MRI | 1.23 | 0.65 | 74 | 40 |
Fodor et al. [73] | H–K | SIS | Ultrasound | 6.50 | 0.31 | 72 | 89 |
Michener et al. [76] | H–K | SIS | Arthroscopy | 1.63 | 0.61 | 63 | 62 |
Kelly et al. [79] | H–K | SIS | Ultrasound | 1.48 | 0.52 | 74 | 50 |
Chew et al. [72] | H–K | SIS | Ultrasound | 1.30 | 0.40 | 87 | 32 |
Salaffi et al. [77] | H–K | SIS | Ultrasound | 2.50 | 0.51 | 64 | 71 |
Fowler et al. [74] | H–K | SIS | Arthroscopy | 2.10 | 1.60 | 58 | 72 |
Toprak et al. [80] | H–K | SIS | Ultrasound | 1.26 | 0.70 | 67 | 47 |
4.3.3.3 Painful Arc Sign
The painful arc sign [71] is elicited with resisted abduction of the shoulder in the scapular plane (20–30° of forward angulation) with the elbow in full extension (Fig. 4.16). Pain with this maneuver is another possible indicator of subacromial impingement, especially when used in combination with the tests described above. Although this test is not very sensitive in isolation, it does boast a modest specificity as reported by Hegedus et al. [81] (Table 4.3). Therefore, since both the Neer impingement sign and the Hawkins–Kennedy test each has low specificity values, addition of the painful arc sign (which has a higher reported specificity) may improve overall diagnostic accuracy when all three maneuvers are used in a composite exam to diagnose subacromial impingement.
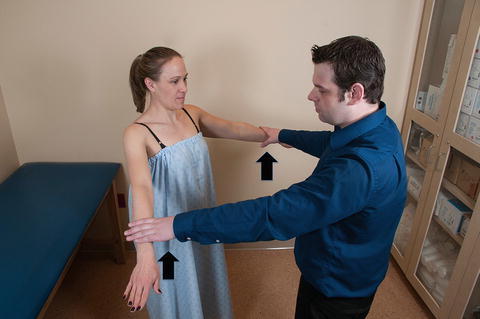
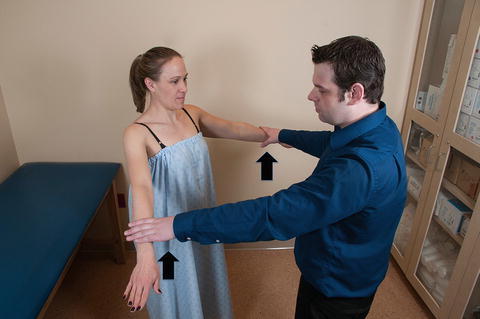
Fig. 4.16
Painful arc sign. In this test, the patient attempts to abduct the humerus within the scapular plane against resistance provided by the examiner. Weakness or pain with this maneuver may indicate the presence of subacromial impingement, although other tests are needed to confirm this finding. In this image, both arms are tested simultaneously which may be more sensitive for the detection of more subtle pathology.
Table 4.3
Diagnostic efficacy of the painful arc sign in isolation
Diagnostic efficacy of the painful arc sign in isolation | |||||||
---|---|---|---|---|---|---|---|
Investigators | Maneuver | Pathology | Standard | LR+ | LR− | Sensitivity (%) | Specificity (%) |
Fodor et al. [73] | Painful arc | SIS | Ultrasound | 3.40 | 0.41 | 67 | 80 |
Michener et al. [76] | Painful arc | SIS | Arthroscopy | 2.25 | 0.38 | 75 | 67 |
Kelly et al. [79] | Painful arc | SIS | Ultrasound | 0.59 | 1.40 | 49 | 33 |
Chew et al. [72] | Painful arc | SIS | Ultrasound | 3.70 | 0.40 | 71 | 81 |
4.4 Subcoracoid Impingement
Subcoracoid impingement is a potential cause for anterior shoulder pain as a result of compression of the subscapularis between the posterolateral edge of the coracoid process and the lesser tuberosity of the humerus [83–86]. In contrast to subacromial impingement which likely involves a multitude of intrinsic and extrinsic factors, most authors agree that many subscapularis tears associated with a narrowed coracohumeral interval (i.e., the distance between the coracoid tip to the crest of the lesser tuberosity measured by axial MRI scans) are most likely caused by external tendon compression (Fig. 4.17) [86, 88].
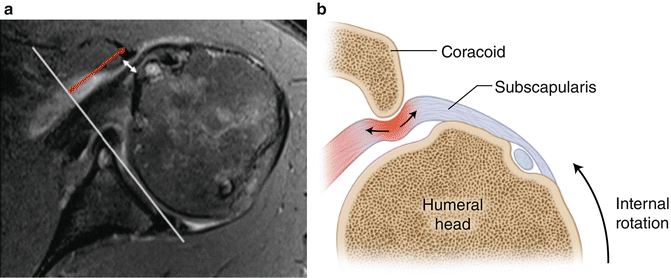
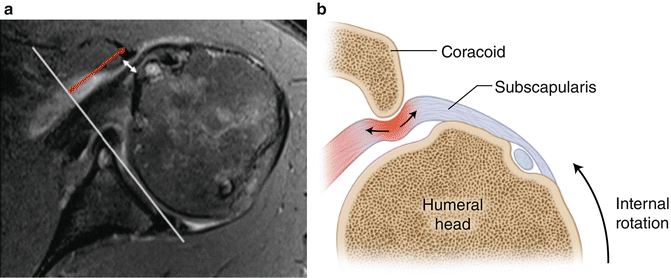
Fig. 4.17
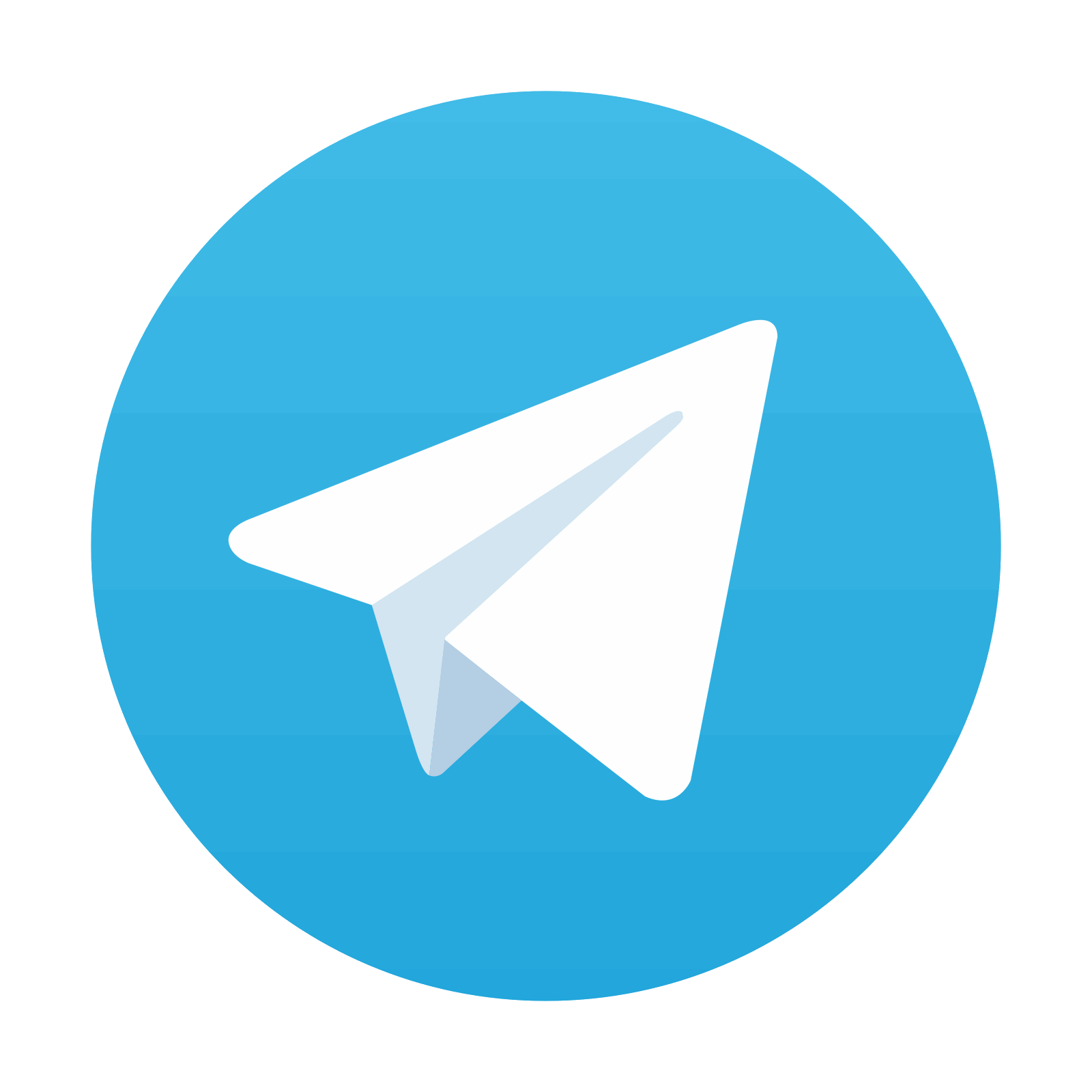
(a) Axial MRI slice demonstrating measurement of the coracoid index and the coracohumeral interval with the humerus internally rotated. The white line connects the anterior and posterior glenoid rim. The double-headed red arrow lies perpendicular to the white line and travels to the most lateral tip of the coracoid process. The distance traveled by the red arrow represents the coracoid index. The double-headed white arrow represents the distance between the lesser tuberosity and the most posterior aspect of the coracoid process. The distanced traveled by the white arrow represents the coracohumeral interval. (b) Illustration depicting the mechanism of impingement between the lesser tuberosity and the posterior aspect of the coracoid. When the humerus is internally rotated, the coracoid induces a “roller wringer” effect on the subscapularis tendon which induces stretching and tearing of the tendon when the coracohumeral interval is narrowed [87].
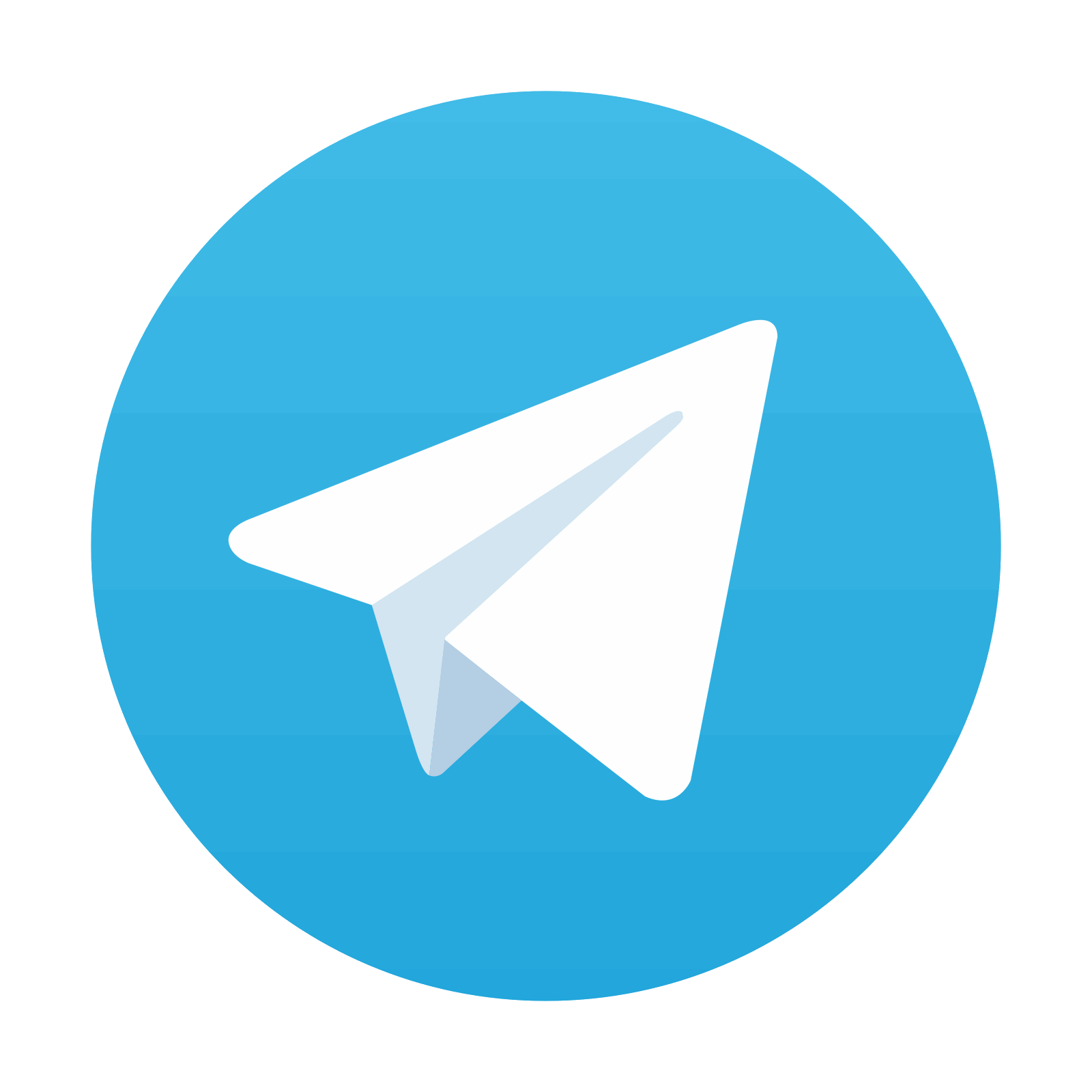
Stay updated, free articles. Join our Telegram channel

Full access? Get Clinical Tree
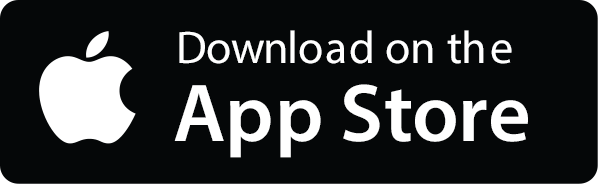
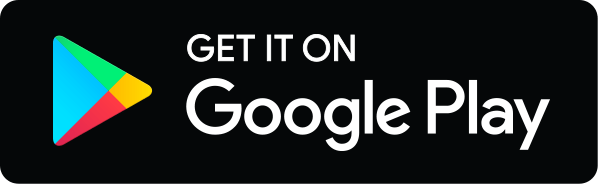
