and Peter J. Millett2
(1)
Steadman Philippon Research Institute, Vail, CO, USA
(2)
The Steadman Clinic, Steadman Philippon Research Institute, Vail, CO, USA
Keywords
ShoulderRange of motionPhysical examClinical exam2.1 Introduction
Range of motion evaluation is a critical component of physical examination for any joint. However, the shoulder is unique in that it provides a large arc of motion in three-dimensional space which presents certain challenges for the treating physician. Determining which motions are clinically significant is perhaps the most important aspect of the range of motion examination. The clinician must then use this information to determine which static and dynamic factors are involved in the patient’s pathologic processes. An understanding of the basic concepts and current evidence surrounding shoulder range of motion testing is the cornerstone for an accurate and efficient physical examination.
2.2 Glenohumeral Motion
Knowledge of a few basic concepts of glenohumeral motion is required to understand, perform, and interpret many physical examination maneuvers. As such, there exists an internationally standardized nomenclature through which basic glenohumeral motions are described to allow for reliable and reproducible communication between clinicians, physical therapists, and anyone else involved in the patient’s care.
In 1923, Silver [1] made the first attempt to create a standard language for the measurement of shoulder motion. He described a “zero point” for each joint from which various motions would be measured. Cave and Roberts [2] followed in 1936 by suggesting that shoulder measurements should be made with the humerus at the side and the elbow flexed to 90°. Importantly, Cave and Roberts [2] were also the first to recommend measurement of the joints above and below the affected joint with a goniometer (discussed below).
Later in the century, further progress was made in the development of a standard nomenclature. Both the American Medical Association (AMA) [3] and the American Academy of Orthopaedic Surgeons (AAOS) [4] created committees that would eventually come to an agreement regarding this language by the early 1960s. The resulting publications defined the most important shoulder motions as flexion, abduction, adduction, extension, and internal and external rotation both with the arm at the side and at 90° of abduction.
When considering various shoulder positions, it is most useful to first note the position of the humerus alone rather than noting the position of the rest of the extremity, such as the elbow and hand. For example, pronation of the forearm does not constitute internal rotation of the shoulder in many cases. Similarly, supination of the forearm does not constitute external rotation of the shoulder. Thus, it is most important to examine the scapulohumeral relationship without regard to the rest of the extremity when evaluating shoulder motion.
2.2.1 Forward Flexion
Forward flexion of the shoulder is defined as elevation of the humerus in front of the body in the sagittal plane (Fig. 2.1). This motion is typically governed by contraction of the anterior fibers of the deltoid muscle and weakness is often a key indicator for several different pathologies. Although the position of the elbow joint was never specified by the AMA [3] or AAOS [4] back in the 1960s, most practitioners measure flexion range of motion with the elbow extended. However, there are some other maneuvers that can be used specifically to measure deltoid strength. For example, having the patient make a fist and push anteriorly against the examiners hand would also activate the deltoid and simulate a forward elevation motion without fully elevating the shoulder overhead (see Chap. 3). This method is particularly useful when evaluating deltoid strength either before or after an arthroplasty procedure where full elevation may not be possible.
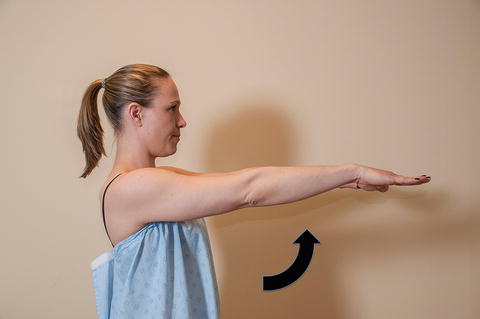
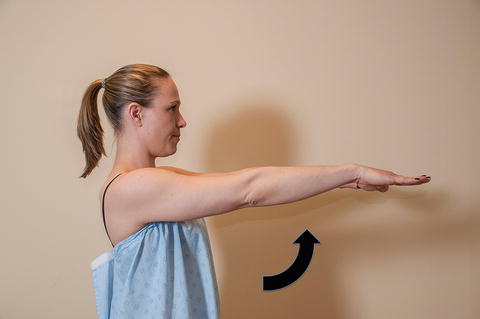
Fig. 2.1
Demonstration of forward flexion in which the humerus is elevated in front of the body (curved arrow).
2.2.2 Abduction
Abduction of the shoulder occurs when the humerus is elevated in the coronal plane such that the extremity points directly laterally (also known as straight lateral abduction) (Fig. 2.2). This position should be differentiated from abduction within the scapular plane which places the humerus in approximately 20–30° of forward angulation, also termed “scaption” (Fig. 2.3). This slight forward angulation facilitates examination such that surrounding soft tissues are similarly lax on both the anterior and posterior sides of the joint. For example, examination of the patient with straight lateral abduction of the shoulder would place an increased stress on anterior structures relative to posterior structures (Fig. 2.4). Thus, any evaluation of the capsular structures may produce inaccurate results when the humerus is abducted in the coronal plane. For this reason, appropriate glenohumeral and scapulothoracic resting positions should be utilized such that accurate assessment can be achieved (the scapular plane and the glenohumeral resting positions are discussed in more detail below).
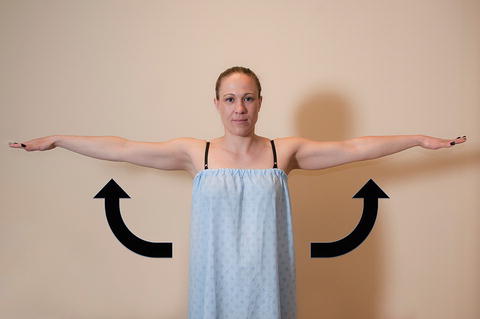
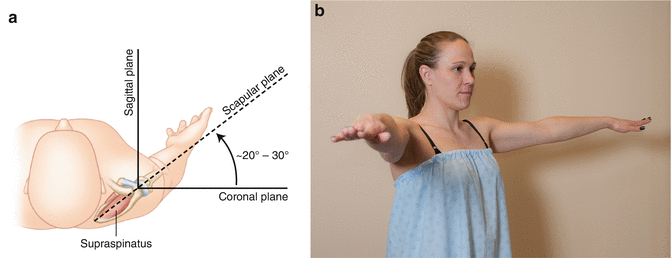
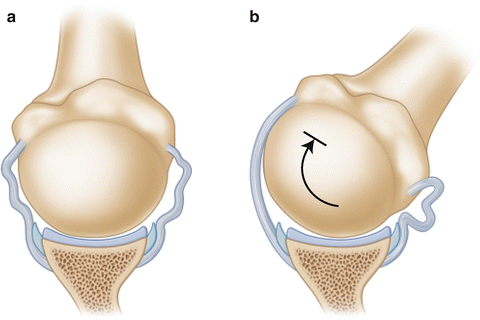
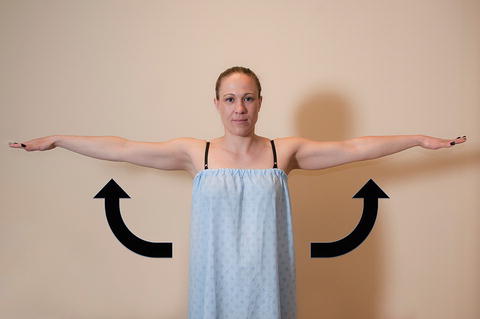
Fig. 2.2
Demonstration of humeral abduction in which the humerus is elevated in the coronal plane (curved arrows).
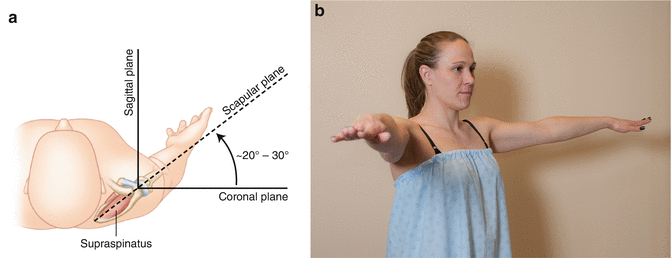
Fig. 2.3
(a) Illustration depicting the orientation of the scapular plane in which the humerus is elevated with approximately 20–30° of forward angulation relative to the coronal plane. (b) Demonstration of humeral abduction within the scapular plane.
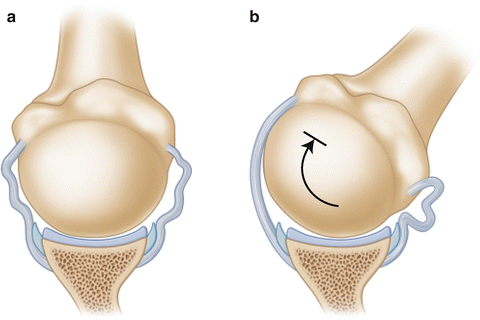
Fig. 2.4
Illustrations depicting the change in capsular tension when the humerus is elevated in the (a) scapular plane and (b) the coronal plane. Abduction in the scapular plane allows for accurate range of motion estimation because both the anterior and posterior capsular structures are similarly lax. Abduction in the coronal plane, on the other hand, can be regarded as a position of extension in which anterior capsular structures become more tight when compared to posterior capsular structures. Measuring range of motion or joint laxity in this position may produce inaccurate results.
2.2.3 Extension
Extension of the shoulder typically refers to any position in which the humerus rotates beyond the scapular plane (Fig. 2.5). This can be achieved with the humerus at the side or elevated. With the arm at the side, the humerus can extend posteriorly in a limited capacity. Straight lateral abduction (also known as “horizontal abduction”) as discussed above is also considered a position of extension since the humerus would be angulated posterior to the plane of the scapula. Similarly, the humerus can also extend posteriorly when the arm is overhead. For example, throwing athletes require combined overhead extension and external rotation to achieve maximal torque and potential energy. Sometimes, this motion sequence can lead to pathologic problems such as symptomatic internal impingement, SLAP tears, and scapular dyskinesis.
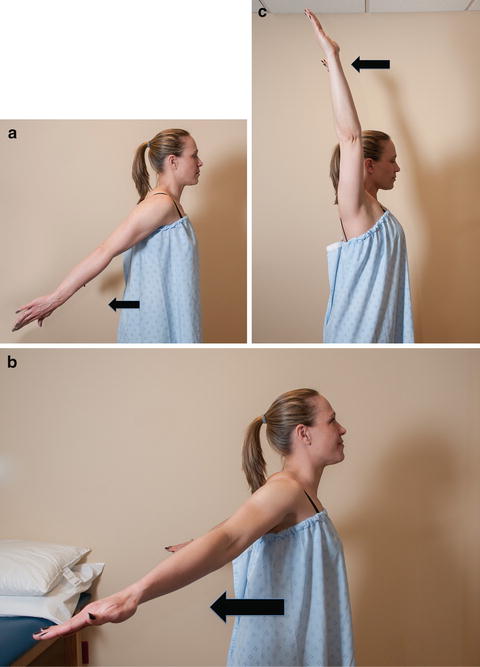
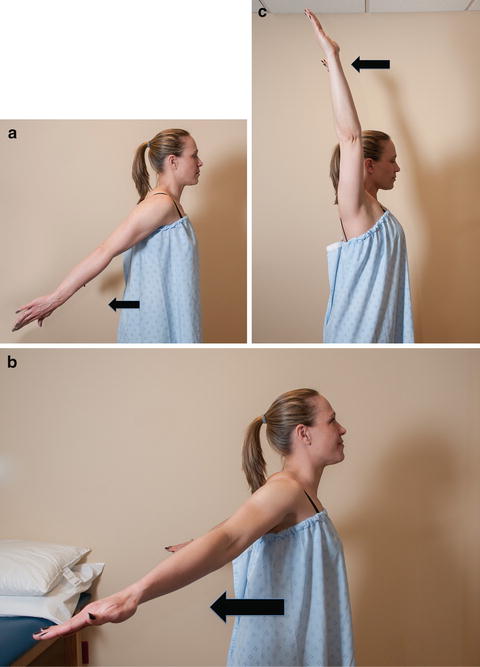
Fig. 2.5
(a) Extension with the arms at the side (arrow). (b) Extension with the arms abducted (arrow). (c) Extension with the arms overhead (arrow).
2.2.4 Internal Rotation
Internal rotation describes motion around a center of rotation such that the angular motion vector points towards the midline. For example, when viewing a right shoulder from the superior to inferior direction, a counterclockwise rotation of the humerus would be referred to as internal rotation (Fig. 2.6). Internal rotation with the arm in a position of 90° of abduction or 90° of flexion uses the same concept. The humerus rotates in the same direction in each case, regardless of the position of the elbow, forearm, or hand. Internal rotation can also be measured with the arm in the adducted position. In this case, the patient will attempt to reach as far up the spinal column as possible while the clinician determines the most superior spinal level that the patient can reach (Fig. 2.7). This position maximizes internal rotation and has historically been a standard measure for internal rotation capacity. However, this method of measurement has recently been called to question since the vertebral level to which one reaches may be influenced by elbow, wrist, and hand motion rather than isolated internal rotation of the humerus [5].
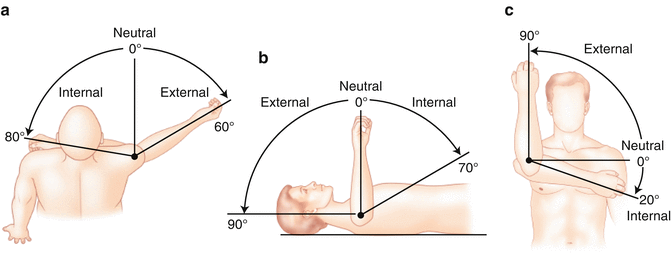
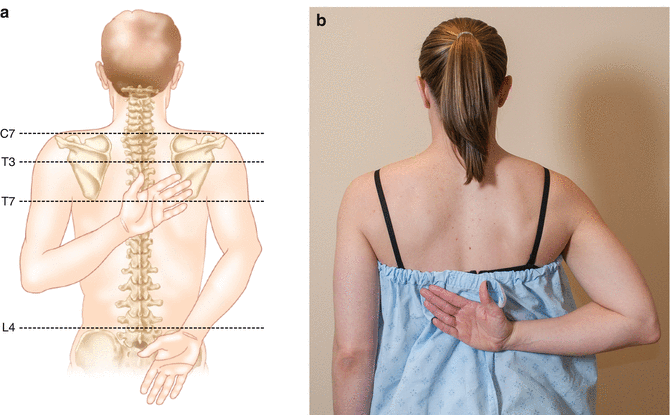
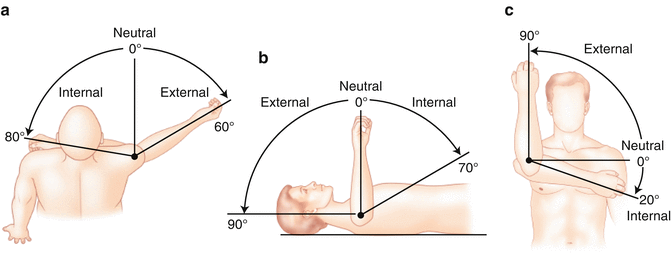
Fig. 2.6
Illustrations depicting glenohumeral internal and external rotation (a) with the arm at the side, (b) with the arm in straight lateral abduction, and (c) with the arm flexed.
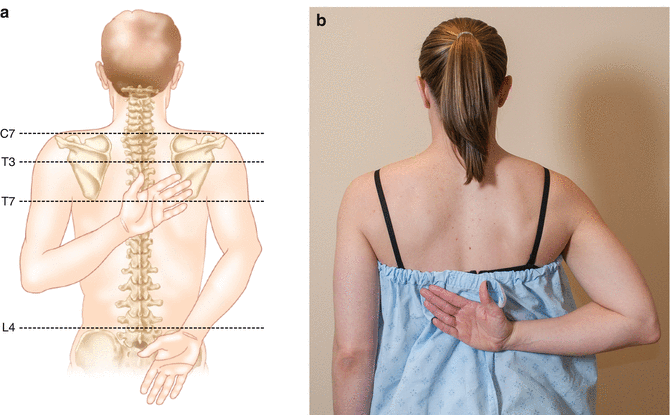
Fig. 2.7
(a) Illustration demonstrating the measurement of internal rotation according to vertebral levels. (b) Demonstration of the positioning for the measurement of internal rotation according to vertebral levels (curved arrow).
2.2.5 External Rotation
When viewing a right shoulder from superiorly to inferiorly, external rotation would be defined as clockwise rotation of the humerus away from the midline (see Fig. 2.6). Again, similar to internal rotation, the rotational moment about the humeral anatomic axis does not change whether the humerus is abducted or flexed—it is only the scapulohumeral angle that changes.
2.2.6 Adduction
Shoulder adduction can also be described with the arm at the side or elevated. The basic resting position with the arm at the side is often referred to as “simple adduction.” When the humerus is elevated to 90° followed by movement of the humerus towards the opposite shoulder, this is most often referred to as “horizontal adduction” or “cross-body adduction” (Fig. 2.8). Conversely, “horizontal extension” corresponds to the opposite motion, where the humerus is extended posteriorly beyond the scapular plane.
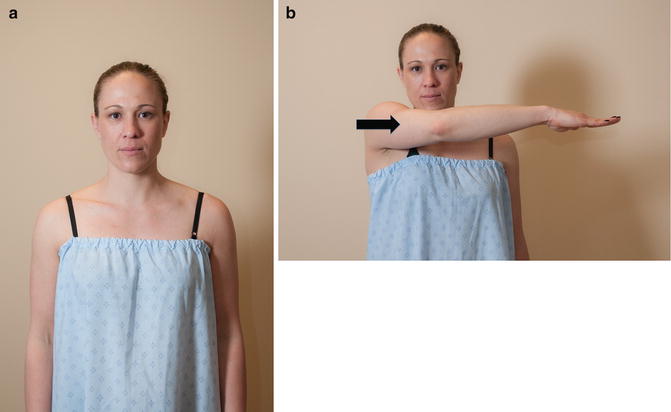
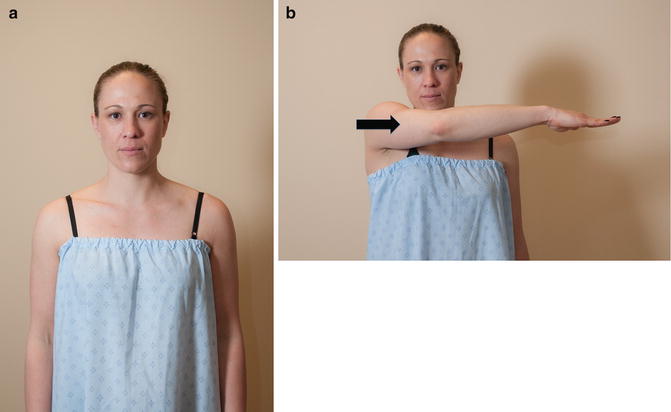
Fig. 2.8
(a) Demonstration of simple adduction with the humerus resting at the side. (b) Demonstration of horizontal adduction in which the humerus is elevated and rotated towards the contralateral shoulder (arrow).
2.2.7 Scapular Plane
The scapular plane is generally defined as a position of neutral scapulohumeral angulation which optimizes glenohumeral joint congruity and facilitates accurate and consistent evaluation of the joint. This position of neutral scapulohumeral angulation is determined by the angle between a line drawn along the center axis of the scapula and second line drawn at the same level that is perpendicular to the coronal plane (see Fig. 2.3). This position, which most often occurs between 20° and 30° of forward angulation relative to the coronal plane with the humerus in various degrees of abduction, minimizes the potential for acromiohumeral contact while also allowing for the theoretical isolation of the rotator cuff musculature during various clinical examination tests. In other words, some have theorized that abduction of the humerus within the scapular plane requires zero contribution from internal or external rotators to achieve full abduction capacity [6]. Maximum capsuloligamentous laxity also occurs within the scapular plane (at the glenohumeral resting position, discussed below) which facilitates examination of these structures (instability and laxity testing are discussed in Chap. 6).
Although the scapular plane is generally defined as 20–30° of humeral forward angulation relative to the coronal plane in normal individuals, it must be recognized that patients with scapular malposition or dyskinesis, as which occurs commonly in overhead athletes, may have a scapular plane that differs from the rest of the population. For example, a throwing athlete with scapular malposition may display increased protraction and upward rotation in the resting position (discussed below), thus altering the position of the glenoid such that the plane of the scapula occurs with greater forward angulation of the humerus. Therefore, performing physical examination tests within the “normal” scapular plane in a patient with scapular malposition may produce inaccurate results (specific examination maneuvers for evaluation of the scapulothoracic articulation are presented in Chap. 9).
2.2.8 Glenohumeral Resting Position
Also known as the “loose pack position,” the resting position of a joint is the position at which surrounding soft tissues are under the least amount of tension, the joint capsule has its greatest laxity and the bony surfaces of the joint are minimally congruent [7–9]. In other words, this position is considered to allow maximal glenohumeral mobility owing to an increase in joint laxity [8]. The glenohumeral resting position in normal shoulders is thought to be between 55° and 70° of abduction with the humerus in neutral rotation within the plane of the scapula (Fig. 2.9) [10–12]. In this position, the amount of external force required to translate the humeral head is minimal which is thought to facilitate examination accuracy. Although there is a general consensus regarding the location of the glenohumeral resting position, validation studies have seldom been conducted.
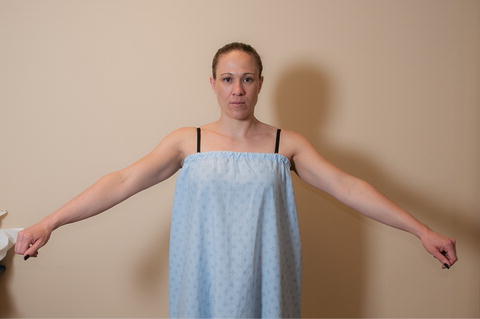
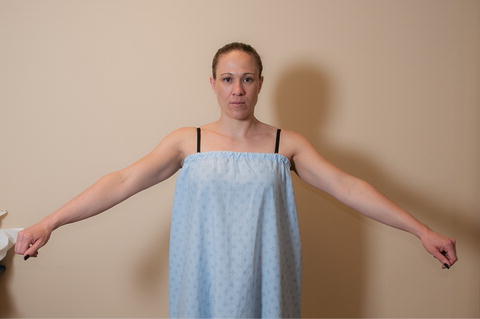
Fig. 2.9
Demonstration of the approximate glenohumeral resting position with the humerus abducted to 55–70° within scapular plane and in neutral rotation.
In a cadaveric study, An et al. [13] evaluated arm elevation in positions of either internal or external rotation. In this study, maximum elevation occurred with the arm externally rotated within the plane of the scapula. They could not achieve this maximal elevation with the arm internally rotated due to the acromiohumeral impingement that occurs in this position. In other words, there was bony contact between the acromion and the greater tuberosity, thus hindering the ability to further elevate the arm. When the humerus was placed in a position of 30° of forward angulation, there was little contribution from the internal and external rotators during humeral abduction.
In 2002, Hsu et al. [14] used seven cadaveric specimens to measure the translational and rotational range of motion at different angles of humeral abduction within the scapular plane. The glenohumeral resting position was calculated as the mid-point of the confidence intervals where maximal rotational and translational motion occurred. Maximal anteroposterior translation and maximal rotational range of motion occurred at approximately 39° of humeral abduction in the scapular plane and corresponded to approximately 45 % of the maximum available abduction range of motion. They also found that the glenohumeral resting position varied according to the maximal available range of motion, possibly suggesting that patients with joint hypermobility and hypomobility should be tested at greater and lesser degrees of humeral abduction, respectively. Since this was a cadaveric study, the effect of dynamic glenohumeral stabilization (which also contributes to the resting position) could not be evaluated.
More recently, Lin et al. [9] attempted to define the glenohumeral resting position in vivo in the dominant shoulders of 15 healthy patients. In that study, translational and rotational range of motion capacities were determined using an electromagnetic tracking device after an 80 N translational load and a 4 N-m (torque) rotational load were applied. The greatest maximal rotational range of motion occurred at approximately 49.8° of abduction in the scapular plane. However, in contrast to Hsu et al. [14], the greatest maximal anterior–posterior translation occurred at approximately 23.7° of abduction in the scapular plane. These results suggested that testing for anteroposterior joint laxity should be conducted at lower degrees of abduction than when testing for rotational joint laxity.
Considered together, these studies demonstrate the complexity and potential variability that the glenohumeral resting position can have across a population, between populations or even between individuals (dominant versus non-dominant shoulders). In general, it is important to determine the maximal range of translational and rotational range of motion for each patient. In general, the rotational resting position is thought to occur at a point near 45 % of the total abduction arc [14] where half of this abduction angle is thought to represent the translational resting position [9].
2.2.9 Codman’s Paradox
Codman’s paradox is the observation that as the arm is flexed upward in the sagittal plane and let down in the coronal plane, the humerus appears to rotate 180° as evidenced by the orientation of the palm. In other words, when beginning the motion, the palm faces posteriorly and, at the end of the motion, the palm faces anteriorly (Fig. 2.10). Alternatively, an individual can place their hand at the top of the head through either (1) forward flexion and internal rotation or (2) abduction and external rotation. This observation has traditionally been of academic interest; however, many investigators have attempted to mathematically solve the “paradox” using complex equations and algorithms [15, 16]. Although the clinical relevance of Codman’s paradox is debatable, some authors have investigated an application of Codman’s paradox during manipulation of a stiff shoulder under anesthesia [17]. In addition, the quadrant test (discussed later in this chapter) is based on Codman’s paradox and can be a useful measure of global shoulder motion [18].
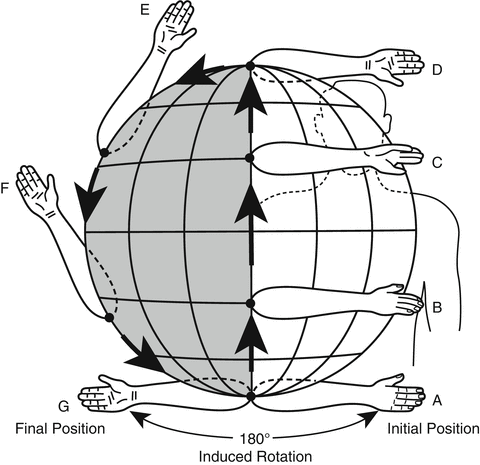
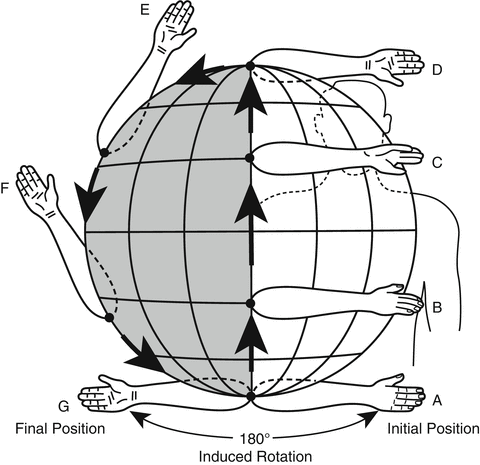
Fig. 2.10
Illustration of Codman’s paradox demonstrating that the top of the head can be reached via forward flexion and internal rotation or abduction and external rotation. (Matsen FA 3rd, Lippitt SB, Sidles JA, Harryman DT II (eds) Practical evaluation and management of the shoulder. W.B. Saunders Company, Philadelphia, 1994) Chapter 2.
2.3 Scapulothoracic Motion
The role of the scapula in the development and progression of various pathologies has been studied extensively over the most recent decade. Some authors have suggested that scapular malposition may be involved with both external and internal impingement mechanisms, especially in overhead athletes [19–24]. The scapula has four basic functions with regard to shoulder motion. The first function is to dynamically position the glenoid in space to facilitate the generation of a large arc of glenohumeral motion. Second, the scapula provides a stable fulcrum upon which glenohumeral motion can arise. Third, dynamic scapular positioning allows the rotator cuff tendons to glide smoothly beneath the acromion with humeral elevation. Finally, the scapula functions to transfer potential energy through the kinetic chain, into the shoulder and, finally, to the hand thus allowing for functional overhead motion. Changes in scapular positioning as a result of alterations in the dynamic periscapular muscle force couples leads to scapular dyskinesis.
Evaluation of the scapular range of motion is one of the most difficult aspects of the shoulder examination for several reasons. One reason is that scapular motion is very complex and requires the examiner to visualize motion in three dimensions. Another reason is that the relative contributions of glenohumeral and scapulothoracic motions are difficult to distinguish, especially when abnormal motions are the result of muscle compensation for some other shoulder condition outside of the scapulothoracic articulation. The scapula is also covered with large, thick muscles making it difficult to visualize or palpate the various scapular motions. In addition, there exists a change in nomenclature when referring to scapular motion (discussed below). Specific examination maneuvers used to examine the scapulothoracic articulation are presented in Chap. 9.
To help the reader thoroughly understand scapulothoracic motion, we have organized the remainder of this section according to increasing complexity, beginning with the scapular resting position and two-dimensional motion planes followed by the interpretation of three-dimensional motion.
2.3.1 Scapular Resting Position
With the arm at rest, the scapula is predictably positioned in a specific orientation that can be used to detect scapular malposition before any motion measurements or evaluations are undertaken. There have only been a few studies that quantified the precise location of the scapula on the posterior thorax. Sobush et al. [25] quantified the normal scapular resting position in cadavers using the “Lennie test,” or a series of measurements taken from the superomedial and inferomedial angles of the scapula. The distance from the superomedial angle to the midline, the distance from the inferomedial angle to the midline and also the angle of scapular inclination were determined (i.e., the angle formed between a line connecting the spinous processes and a line drawn along the margin of the medial scapular border). This test was found to have high inter-rater reliability and accuracy when compared to post-measurement radiographs. Using similar measurements, a cadaveric study by Fung et al. [26] found that the resting position of the scapula was at approximately 3° of external rotation, 40° of internal rotation, and 2° of posterior tilt. Of note, this nomenclature does not reflect the position of the humerus. Rather, it represents the position of the scapular body relative to the coronal plane (discussed below).
2.3.2 Two-Dimensional Scapular Motion
In order to evaluate three-dimensional scapulothoracic motion, it is perhaps most advantageous to begin with an understanding of the basic two-dimensional scapular motions. In total, there are three rotational movements and two translational movements (Fig. 2.11). Although it is not possible to isolate these movements, they represent the basic components that comprise three-dimensional scapular motion.
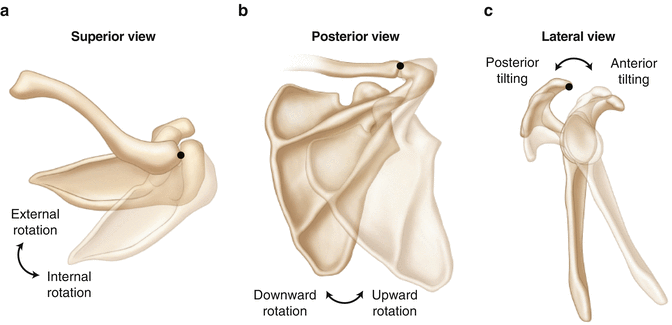
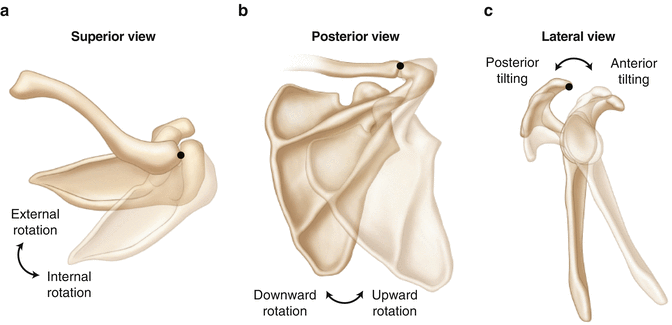
Fig. 2.11
Illustration depicting (a) scapular internal and external rotation, (b) scapular upward and downward rotation, and (c) scapular anterior and posterior tilting.
Internal and external rotation occurs around the vertical axis of the scapula—that is, internal rotation elevates the medial scapular border away from the posterior thorax (i.e., the glenoid faces more anteriorly) whereas external rotation refers to the exact opposite motion (i.e., the glenoid faces less anteriorly).
Upward and downward rotation occurs along the plane of the scapula. In other words, upward rotation occurs when the inferior angle of the scapula moves laterally and the glenoid faces more superiorly. Conversely, downward rotation refers to the opposite motion in which the inferior scapular angle moves medially towards the midline and the glenoid faces more inferiorly.
Anterior and posterior rotation (i.e., tilting) occurs around the horizontal axis of the scapula. Anterior tilting of the scapula occurs when the inferior angle moves away from the thorax (and the superior border moves towards the thorax) whereas posterior tilting refers to the exact opposite motion in which the inferior angle moves towards the thorax (and the superior border moves away from the thorax).
The scapula can also translate in the medial–lateral direction (as in protraction and retraction, described below) and the superior–inferior direction (as in shrugging the shoulders). It is important to recognize that these translational motions also require intact AC and SC joints—upward and downward translation of the scapula requires upward and downward angulation of the clavicle via the SC joint whereas medial–lateral translation requires anterior–posterior motion of the clavicle through the SC joint as the scapula moves around the thorax.
2.3.3 Three-Dimensional Scapular Motion
Three-dimensional scapular motion, which is achieved by combining any of the above-mentioned two-dimensional movements, is necessary to optimize glenohumeral contact and stability throughout the entire range of shoulder motion. However, during the evaluation of an actual patient, it is most useful to consider the observed three-dimensional scapular motion as a summation of the individual rotational moments mentioned above.
The terms “protraction” and “retraction” are most often used to describe scapular movement in three-dimensional space. To understand these terms, it is perhaps easiest to first recognize that scapular motion occurs along a rounded surface (i.e., the convexity of the posterior thorax). Using this approach, one could imagine that any lateral translation of the scapular body would also require scapular internal rotation. This movement also requires some anterior tilt and downward rotation. This combination of movements is generally referred to as scapular “protraction” and can be closely simulated by having the patient thrust their shoulders anteriorly (similar to a hunchback position). Conversely, any medial translation of the scapular body would also require scapular external rotation. This movement also requires some posterior tilt and upward rotation. This combination of movements is typically referred to as scapular “retraction” which can be demonstrated by having the patient thrust their shoulders posteriorly (as in “squeezing” the scapulae together by extending the humerus posteriorly below 90° of elevation) (Fig. 2.12). Of course, neither protraction nor retraction could be achieved without some amount of upward and downward rotation along with anterior and posterior tilt; however, the purpose of the above example is to illustrate the fundamental concept of scapular translation around the posterior chest wall in three dimensions.
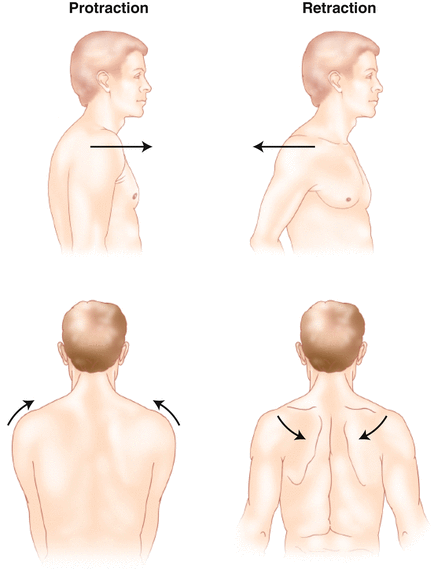
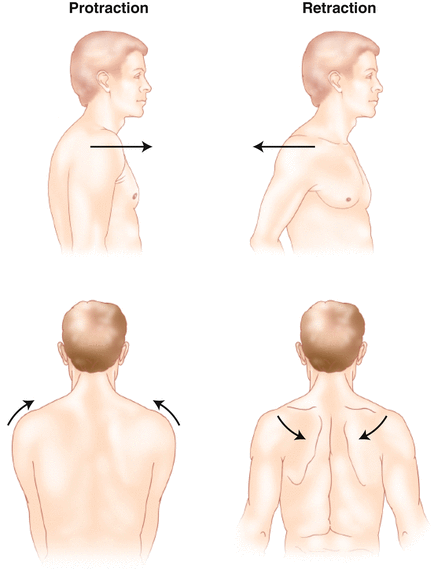
Fig. 2.12
Illustration demonstrating scapular protraction and retraction. Sagittal and posterior views are shown.
This same concept also applies when the scapula translates superiorly or inferiorly along the convex surface of the posterior thorax. In other words, inferior translation of the scapula would theoretically produce an increased posterior tilt whereas superior translation of the scapula would produce an increased anterior tilt (as in shrugging the shoulders). In reality, the shoulder shrug requires a combination of superior translation, anterior tilt, and internal rotation. Increased anterior or posterior scapular tilt is very subtle, is difficult to recognize by direct visual or tactile examination in the office setting, and generally cannot be isolated by any specific voluntary movement. Biomechanical studies suggest that scapular tilting mostly occurs during extension-type maneuvers with the arm either overhead or at the side.
Most clinicians agree that isolated glenohumeral motion occurs below approximately 90° of elevation whereas combined glenohumeral and scapulothoracic motion occurs above this level (discussed below). In order to maximize glenohumeral contact and stability during this combined motion, the scapular stabilizers must not only contract in synchrony with each other, but also with each of the muscles that cross the glenohumeral joint along with the proprioceptive feedback obtained from surrounding soft-tissue structures (such as the glenohumeral joint capsule [6]). Although a thorough discussion of each possible scapular movement is beyond the scope of this book, we aim to emphasize the extreme importance of understanding the fundamental concepts related to three-dimensional scapular motion. It is with this foundational knowledge that one can begin to understand the complex disease processes related to the shoulder.
2.3.4 Roles of the AC and SC Joints in Scapular Motion
The clavicle acts as a strut which allows for the strategic positioning of the shoulder girdle along the side of the thorax. In order to maximize shoulder range of motion, the clavicle must be dynamically positioned according to scapular motion via the AC and SC joints. Therefore, the health of the clavicle and the AC and SC joints is extremely important to achieve normal scapular motion in three-dimensional space [26–28]. For example, arm elevation requires the clavicle to retract, elevate, translate, and rotate posteriorly along its long axis (i.e., the so-called “screw axis”) where each of these movements is dependent on the function of intact, painless AC and SC joints [29, 30].
2.4 Differentiating Between Glenohumeral and Scapulothoracic Motion
The ability to elevate the arm overhead through any plane relies on dynamic scapular positioning which essentially places the glenoid in a position of maximum contact with the humeral head. Due to the three-dimensional complexity of scapular motion, it may be difficult for an inexperienced examiner to differentiate between the glenohumeral and scapulothoracic components of shoulder elevation. Many investigators have proposed methods of isolating each movement, thus allowing clinicians to more easily diagnose common shoulder problems. For example, in order to achieve normal cross-body adduction, the scapula must protract to maintain adequate glenohumeral contact and stability (i.e., the scapula must translate laterally and internally rotate to conform with the convexity of the posterior chest wall). While measuring posterior capsular tightness using a cross-body adduction technique (described below), the clinician must first stabilize the scapula to minimize protraction. Otherwise, the measured amount of total combined adduction capacity will almost always be significantly greater than the true isolated glenohumeral adduction capacity as a result of the additive effect of scapular protraction.
Although complete isolation is probably not feasible in the clinical setting, clinicians can usually estimate the amount of isolated glenohumeral motion by detecting (or, in some cases, stabilizing) scapular motion. In the case of humeral elevation, the examiner can place one hand over the scapula (with the thumb over the scapular spine and the fingers wrapped anteriorly over the top of the shoulder) and ask the patient to slowly flex or abduct the humerus. During this movement, the examiner uses their hand to determine the point at which the scapula begins to translate or rotate. It is then assumed that any degree of elevation below this level would be composed of primarily glenohumeral motion whereas any motion above this level would involve a combination of glenohumeral and scapulothoracic motion (Fig. 2.13). The same concept can theoretically be applied to a variety of other testing procedures where isolation of glenohumeral motion is desired. In contrast to the method of detecting scapular motion, the examiner can also stabilize the scapula by applying a downward force to the top of the shoulder during shoulder elevation. In many cases, the same effect can be achieved by performing certain examination maneuvers with the patient placed supine on the examination table (i.e., laying on a flat surface is thought to limit scapular motion during testing).
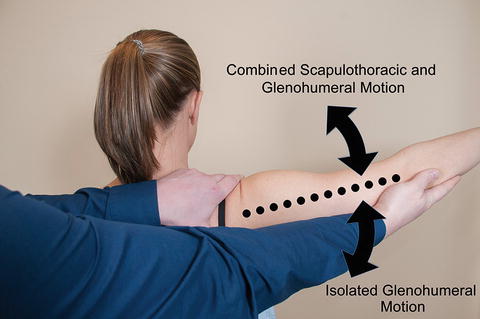
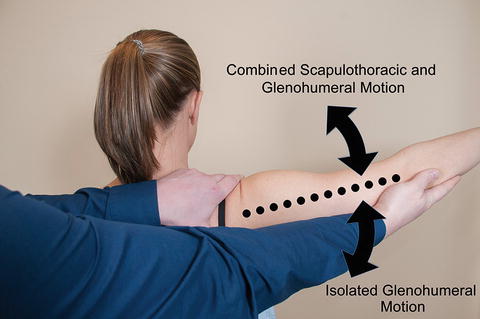
Fig. 2.13
Isolated glenohumeral motion versus combined glenohumeral and scapulothoracic motion. In this subject, the scapula began to rotate at approximately 100° of humeral abduction. Therefore, motion above this point is considered combined glenohumeral and scapulothoracic motion whereas motion below this point is considered isolated glenohumeral motion.
The exact transition point between isolated and combined motion has been debated. Clarke et al. [31] found that passive isolated glenohumeral abduction in a series of young, healthy patients occurred below 85.6° in females and below 77.4° in males. Gagey and Gagey [32] found a similar result in which 95 % of their subjects with normal shoulders transitioned to combined scapulothoracic motion between 85° and 90° of glenohumeral elevation. In contrast, Sauers et al. [33] found that the transition occurred at approximately 112° of glenohumeral elevation and Lintner et al. [34] found that the transition occurred at approximately 109° of glenohumeral elevation.
Due to these conflicting results, the reliability of this method in the measurement of isolated glenohumeral motion came into question. A study by Hoving et al. [35] determined that the intra-rater reliability for isolated glenohumeral motion was only 0.35; however, the study involved a series of patients with varying degrees of shoulder pain which may have confounded their results. In addition, the clinicians were somewhat unfamiliar with the digital inclinometers that were used in the study, potentially blurring the interpretability of their results.
Several biomechanical studies have suggested that although the majority of scapular motion occurs above 90° of glenohumeral elevation, there does exist some scapular motion below this level. This fact calls into question the ability of an examiner to completely isolate glenohumeral elevation. Currently, it is thought that the scapula moves throughout the total arc of shoulder elevation and that complete isolation of glenohumeral motion is probably not realistic. However, when the angle of glenohumeral elevation is less than 90°, the degree and quality of glenohumeral motion can be reliably estimated.
The above discussion only considers the ability of an examiner to isolate glenohumeral motion during arm elevation. No published studies have examined the ability of an examiner to isolate glenohumeral rotation. However, the results of an unpublished cadaveric study by McFarland et al. [36] and Yap et al. [37] that were presented at the 1998 annual meeting of the Orthopedic Research Society in New Orleans, LA and the annual meeting of the American College of Sports Medicine in Orlando, FL in the same year suggested that glenohumeral rotation may be isolated and, potentially, accurately measured to within 2° prior to initiation of scapulothoracic motion. Their methods have not been validated in the literature to date.
2.5 End Feel Classification
Accurate range of motion testing requires that the examiner utilizes both visual and tactile clues that ultimately aid in the entire physical examination process. While the visual clues are obvious in many cases, tactile sensations that are transmitted to the examiner’s hands or fingers as they manipulate the upper extremity are equally important in directing future examination maneuvers and diagnostic studies. With range of motion testing, the concept of end feel is extremely important on several levels. As the glenohumeral joint nears its maximal range of motion, the quality of the end feel can give the clinician an idea of what is happening anatomically.
In 1947, Cyriax and Cyriax [38] described a basic classification system in which normal end feel was characterized as bony, capsular or soft-tissue approximation and abnormal end feel was characterized as spasm, springy block, and empty (Table 2.1). These sensations occurred near the extremes of shoulder motion as a result of bony architecture, muscle contraction, and/or soft-tissue stretching.
Table 2.1
Cyriax and Cyriax end feel classification
End-feel | Description |
---|---|
Capsular | Motion ends gradually, as if a leather band were being stretched. |
Tissue approximation | Motion ends in a manner suggesting that motion would continue if not prevented by another structure. |
Springy block | Motion ends with a noticeable rebound sensation. |
Bony | Motion ends immediately when two hard surfaces come into contact. |
Spasm | Motion ends in a “vibrant twang,” or when motion is counteracted by muscle contraction. |
Empty | Motion does not end, but patient asks examiner to stop maneuver as a result of pain. |
A bony end feel occurs when an abrupt end point is reached as two hard surfaces come into contact (e.g., terminal extension of the elbow). Capsular end feel occurs as the joint approaches an extreme motion plane—further motion becomes increasingly difficult to obtain as the capsule stretches. Cyriax and Cyriax [38] suggested that capsular feel was analogous to a thick leather band being stretched. Soft-tissue approximation occurs when soft tissues prevent further motion, such as in the instance of cross-body adduction or extreme elbow flexion. Muscle spasm can often have a hard end feel and was characterized as a “vibrant twang” towards the extremes of motion. This can especially occur in the evaluation of a patient with instability who demonstrates a positive apprehension sign (the apprehension sign is discussed in Chap. 6). A springy block is felt when an intra-articular block prevents motion, followed by an episode of rebound. An empty end feel occurs when the examiner cannot discern a palpable end point; however, significant pain often prevents further motion.
In 2001, Hayes and Petersen [39] examined the inter- and intra-rater reliability of end feel in patients with painful shoulders and knees. Two physical therapists evaluated each patient twice, measuring two knee motions and five shoulder motions. The examiners noted the character and quality of the end feel at the extremes of range of motion while patients vocalized the exact moment of pain reproduction. The inter-rater κ coefficients for end feel ranged from 0.65–1.00 to 0.59–0.87 for the pain/resistance sequence. However, their study also demonstrated large variations in end feel when the shoulder was abducted. The authors suggested that this discrepancy was related to the fact that the scapulae of the subjects were variably stabilized which may have produced differences in end feel in these patients. In addition to this variation, it is thought that the presence of pain may also have a significant effect on different end feel characteristics [40].
The clinical applicability or validity of the various end feel characteristics has not been evaluated in the literature. The difficulty is that different end feel characteristics probably represent combinations of anatomic variables and pathologic lesions that likely cannot be differentiated by tactile sensation alone. Therefore, despite its widespread application in clinical practice, further study is needed to validate this method of examination before it can be formally advocated.
2.6 Methods of Measurement
Range of motion is defined as the magnitude of motion capacity that exists across a joint. Because most major joints in the body achieve angular (or rotational) movements, range of motion is typically measured in degrees relative to some normative plane. Range of motion measurement is a particularly important aspect of the physical examination that is often overlooked in clinical practice. These measurements can have significant implications regarding treatment approaches and outcomes and should not be omitted when evaluating a new patient with a shoulder complaint. Shoulder range of motion is typically quantified using one of four basic techniques; these include estimation via visual inspection, the use of an inclinometer, the use of a goniometer, the use of a gyroscope or, more recently, digital photography using a high resolution camera or smart phone.
2.6.1 Visual Inspection
Unfortunately, visual estimation is the most commonly used method for the measurement of shoulder range of motion. Although several studies have found that experienced practitioners can estimate range of motion with a similar accuracy to standardized measurement devices [41, 42], the lack of teaching regarding the fundamentals of range of motion testing is disappointing. This results in inaccurate measurement estimations by the novice examiner who was never properly taught to use goniometers or inclinometers. Although formal measurement requires more time to complete, it is suggested that inexperienced examiners use standard measurement devices to aid in accurate patient assessment until they become more knowledgeable and experienced with the examination process. In a busy clinical practice, however, the experienced clinician can usually make rapid range of motion estimations without sacrificing accuracy or precision. Visual inspection and estimation of range of motion is therefore a standard of practice in most cases, but formal measurements are required when a study involving range of motion data is being conducted.
2.6.2 Inclinometers
An inclinometer is essentially a leveling device, similar to that which is used by a carpenter to measure the degree of inclination of a surface relative to the horizontal plane, that is occasionally used in clinical practice to quantify shoulder range of motion or, in some cases, the degree of spinal deformity such as kyphosis or scoliosis [43–45]. Both mechanical and digital inclinometers have been described as reliable and valid tools for the measurement of shoulder range of motion.
Mechanical inclinometers, or hygrometers, use gravity and a fluid-level indicator to measure the inclination of the humerus relative to the horizontal plane in degrees (Fig. 2.14). The first reported use of a mechanical inclinometer to measure range of motion was in 1975 by Clarke et al. [31]. In their study, the inter-observer reliability was approximately 0.93. Similarly, Dover et al. [46] calculated inter- and intra-rater reliability values of approximately 0.99 in the measurement of shoulder range of motion using a mechanical inclinometer. In an adjunct study [47], the same group found that the ability of the inclinometer to detect changes in joint proprioception was also pronounced. In that study, they calculated inter- and intra-observer reliabilities ranging from 0.978 to 0.984.
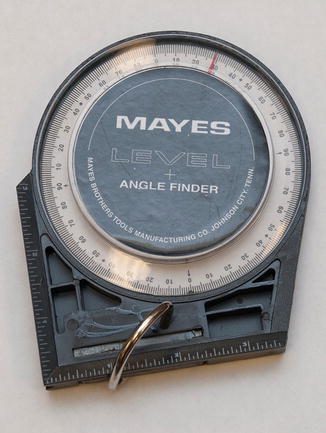
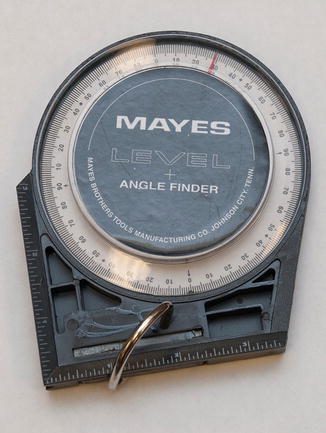
Fig. 2.14
Photograph of a mechanical inclinometer.
Currently, digital inclinometers are more commonly used to assess shoulder range of motion [48–57] and function by calculating the angle of inclination relative to the horizontal plane using an implanted gravity sensor. Kolber et al. [51] determined that the inter- and intra-observer reliabilities of digital inclinometry was greater than 0.95 and found that this method was interchangeable with goniometry (intra-class correlation [ICC] coefficients for goniometry were >0.94) [51]. Scibek and Carcia [54] studied 13 healthy collegiate subjects in an attempt to quantify scapulohumeral rhythm using a digital inclinometer. In that study, the investigators found that the scapula contributed to 2.5 % of shoulder motion in the first 30° of shoulder abduction. This proportion dramatically increased to between 20.9 and 37.5 % when measured between 30° and 90° of humeral abduction, respectively. Johnson et al. [58] calculated the reliability and validity of a digital inclinometer to measure scapular upward rotation during humeral abduction in the scapular plane. They found that the digital inclinometer had excellent reliability and validity in the assessment of scapular motion with inter- and intra-observer ICCs ranging from 0.89 to 0.96. A similar study by Tucker and Ingram [56] calculated ICCs of >0.89 after using a digital inclinometer to quantify scapular upward rotation with static humeral elevation.
More recently, studies by Shin et al. [59] and Mitchell et al. [60] demonstrated the ability of smart phone inclinometers (and goniometers) to accurately measure range of motion with excellent inter- and intra-observer reliability (ICC >0.9). One other study [61] demonstrated the capability of smart phones to measure cervical range of motion. This method of measurement eliminates the cost of standard digital inclinometers, a factor that has limited their widespread use. Nevertheless, these studies demonstrate the utility and practicality of digital inclinometers in the accurate measurement of scapulohumeral rhythm in addition to glenohumeral range of motion capacity.
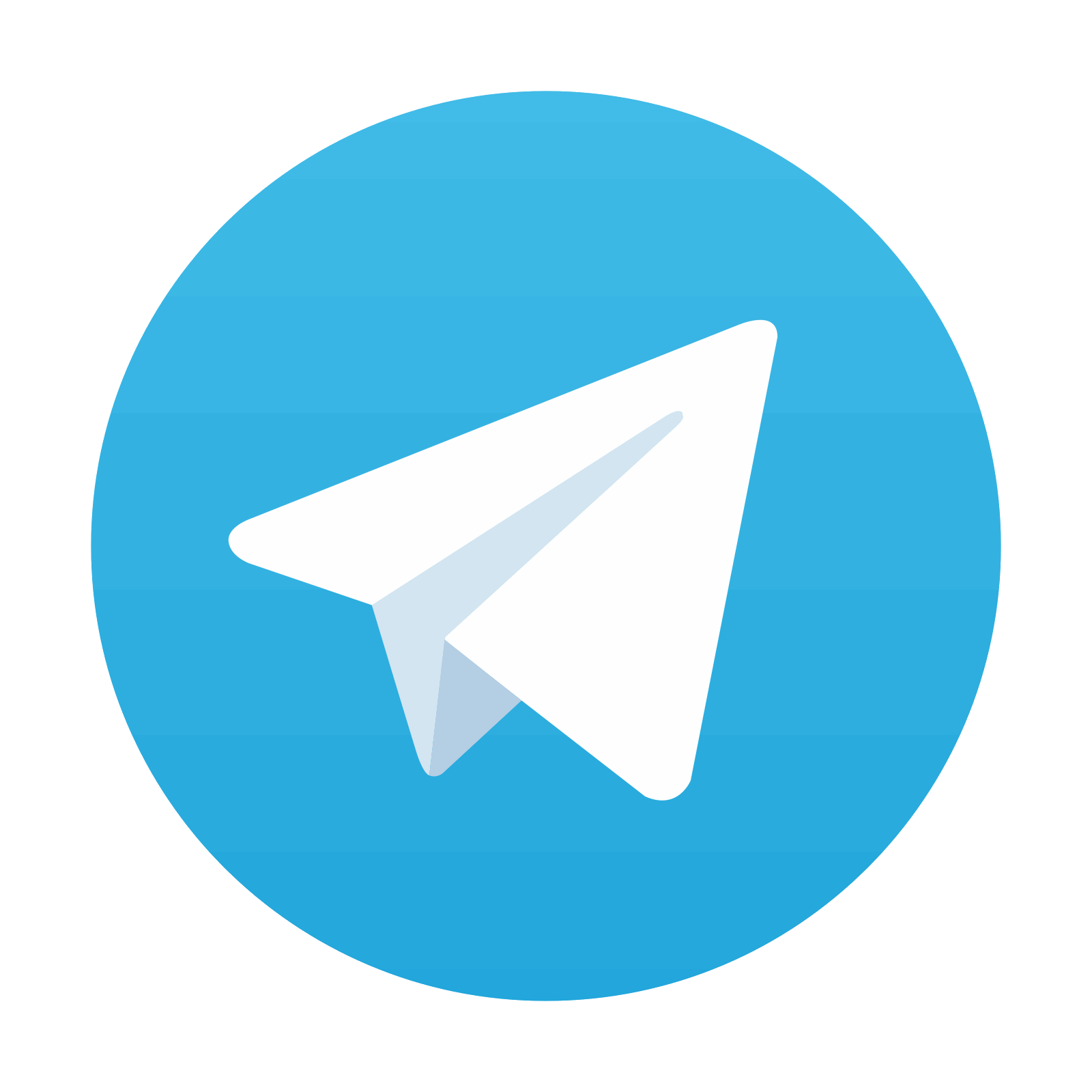
Stay updated, free articles. Join our Telegram channel

Full access? Get Clinical Tree
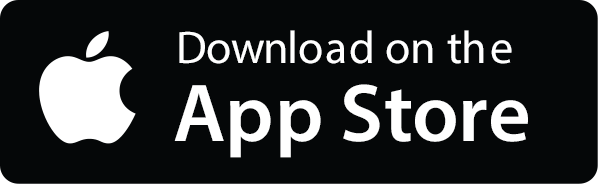
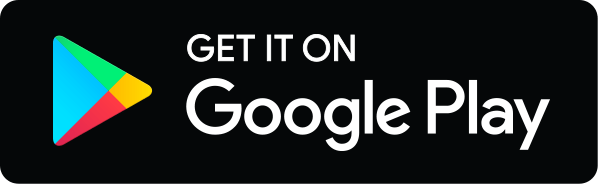