Chapter 16 Postoperative Cartilage Repair Rehabilitation
Introduction
Participation in physical activity is at the forefront of international health promotion agendas, and there is increasing encouragement for the maintenance of participation in sports and exercise activities throughout an individual’s life span.1 Moderate recreational physical exercise is associated with a decrease in the risk of knee osteoarthritis.2 However, participation in high-impact sports can increase the risk of developing articular cartilage lesions,3,4 and athletes are at a higher risk of developing knee osteoarthritis.5 There is increasing evidence that excessive stress on a joint with an articular cartilage lesion may accelerate further degenerative changes6 and that, if unaddressed, small cartilage defects progress to osteoarthritis.7 Messner and Maletius established that 75% of young athletes demonstrated radiographic joint space reduction an average of 14 years after sustaining chondral damage and returning to their preinjury sport levels.8 Consequently, it is not surprising that individuals are seeking out elective surgical procedures more frequently in order to address cartilage defects with the aim of maintaining or regaining their ability to participate in sports and exercise activities.
The ultimate goal for surgical intervention on chondral defects is the restoration of the joint surface to restore “normal” hyaline articular cartilage. Since the late 1980s, orthopaedic surgeons and tissue engineers have immersed themselves in this quest, resulting in the development of a new range of surgical procedures to restore the structure of normal articular cartilage.9 The treatment of articular cartilage defects has undergone a rapid and exciting evolution in recent years, most notably in the field of advanced cell-based orthobiological technologies. However, although some of these surgical interventions have demonstrated great promise, the new tissue in its current state does not precisely replicate natural articular cartilage in terms of form and function. Consequently, these interventions can only be considered reparative rather than the desired restorative.
To maximize the benefits of ACI surgery, it is essential for patients to be well informed and educated in order for them to comply to a specific rehabilitation program.9–11 Patient education, the management of patient expectations, and clear goal setting are indispensable within ACI rehabilitation. These values are reliant on a collaborative environment, with thorough communication between the surgeon, therapist, and patient. In addition, patient selection and the patients’ respective characteristics can affect the functional outcome in an inordinate way. deWindt et al. published a study examining the prognostic factors related to cartilage implantation and found that in lesions smaller than 3 cm2, defect location and defect age were statistically linked to better outcome scores on the knee injury and osteoarthritis outcome score (KOOS) at three years after ACI surgery.12 We cannot underestimate the notion of patient selection and the characteristics of the defect itself.
The two primary goals for an ACI rehabilitation program are as follows:
The three main components of the rehabilitation program are as follows:
Rehabilitation following an ACI procedure typically begins the day after the surgery, beginning with continuous passive motion (CPM). The frequency, duration, and ROM will depend on the location and size of the lesion. Active and passive motion is also utilized to facilitate the integration of the graft into the surrounding articular cartilage and subchondral bone. CPM is clinically recommended for 2 to 8 hours per day (depending on the site of the lesion) at one cycle per minute during the early phase of rehabilitation.
The contact area (distribution and magnitude), contact load, and contact pressure during rehabilitation should be considered to minimize the danger of damaging the graft and to support the healing process by stimulating the graft physiologically in harmless positions. The articulation and contact area at various degrees of knee flexion are of crucial importance to ACI rehabilitation in relationship to the graft location (Table 16-1).
TABLE 16-1 Summary of Patellar Articulation During Knee Flexion and Extension
Articulation | Contact Area | |
---|---|---|
Full extension | Patella sits above femoral articular surface and rests on supratrochlear fat pad. | No patellofemoral contact with femur. |
10°–20° | Initial contact occurs between inferior patella and trochlea. | Joint contact area increases steadily with flexion. Mean contact area at 10° = 126 mm2; mean contact area at 60° = 560 mm2. |
30°–60° | Middle surface of patella makes contact with middle third of trochlea. | |
60°–90° | Superior patella makes contact with trochlea. | Contact area remains constant. |
90°–135° | Superior patella contact area splits into medial and lateral contact areas that articulate with the opposing femoral condyles. | Controversial—research differs, with contact area either leveling off after 90° or continuing to increase with increasing flexion. |
135° | Odd facet of patella contacts medial femoral condyle. | |
Full flexion | Lateral femoral condyle is fully covered by patella, and medial femoral condyle is nearly completely exposed. |
The patella has a large articulating surface, presenting with the thickest layer of articular cartilage in order to optimize the distribution of forces and stresses.13–15 The patellar cartilage presents with multiple facets in a pattern that is unique to each individual, and it does not follow the contour of the underlying subchondral bone.13 The articular surface of the joint is congruent in the axial plane but not in the sagittal plane, and the material properties of the patellar cartilage differ from those in the cartilage of the articulating trochlea.5,13
The kinematics of the tibiofemoral joint is initiated, guided, and limited mainly by the cruciate ligaments, muscles, and capsular structures. Injury or loss of function to one of these structures leads to altered arthrokinematics, which may be deleterious to the menisci and cartilage.16
During normal activities, the joint contact forces (shear and compressive forces) that are produced are attenuated by several structures of the joint. Shear forces are primarily restrained by the cruciate ligaments. Compressive forces are mostly attenuated by the menisci and the articular cartilage.5,17 Excessive shear and compressive forces can be deleterious to the menisci and the cartilage. Numerous studies have measured these forces;16 the exact level of musculoskeletal loading is influenced by a number of individual factors such as weight, gender, movement coordination, and the activity being undertaken.
To develop a safe and effective ACI rehabilitation program, shear forces have to be minimized, and the size and location of the defect have to be known because during several activities only parts of the femur/tibia are articulating.5,18–21 For example, the posterior aspect of the medial femur condyle contacts the tibia between 90° and 120°; therefore, loading in positions between 0° and 80° of knee flexion are unlikely to be injurious to an ACI in this area.
Neuromuscular reeducation is a critical component in the restoration of functional joint stability. Neuromuscular function broadly involves the detection of afferent input via mechanoreceptors locally in the joint, the processing of a motor response to the stimulus in the central nervous system, and the initiation of an efferent reaction to maintain balance, stability, and mobility.22 Rehabilitation can assist in the restoration of proprioception, but high-level studies are scarce.23–25
The exercises should be performed throughout the full available ROM and should be performed on both the involved and the uninvolved limbs because of the likelihood that proprioception deficits are also present in the contralateral limb.13,26–29 Specific exercises for neuromuscular rehabilitation after ACI should be addressed on an individual basis in line with any weight bearing or ROM restrictions that may be in place.
Hydrotherapy
Exercises in water allow early active mobilization and early loading and improve neuromuscular performance, especially during the initial phase of a rehabilitation program.15,25,30,31
The reduction in gravity under water decreases the deleterious effects of weight bearing and dissipates the impact forces on joint structures during movement,13,32 enabling ROM exercises to be performed in a functional position with a reduced risk of high shear forces under compression. Factors such as water depth and flow will also influence the loading demands on the knee joint, so it is important to base the rehabilitation program on the general principles of hydrotherapy.
Exercises under water produce lower electromyography (EMG) activity during isometric and dynamic conditions when compared to similar exercises on dry land,33 thereby leading to lower joint forces. Research has shown that an early and intensive application of hydrotherapy for improving coordination and strength during rehabilitation is advisable.34 In addition, moving in water endows patients with a “feeling of freedom,” as they can walk without assistive devices and move around without restriction. This is an important psychological advantage.
Manual Therapy After ACI
The ability to define passive movement disorders in a joint, the localization of swelling, the involvement of anatomical structures, and temperature, are necessary for good clinical practice and for a comprehensive tailoring of the rehabilitation.5,35,36
Manual therapy as an independent application of manual techniques for general knee disorders is questionable. However, the combination of manual therapy with exercises and specific manual techniques for the enhancement of ROM prove to be more effective than exercises alone.2,37,38
Therapeutic Ultrasound and Laser
Low-intensity pulsed ultrasound (LIPUS)28,34,39 and low-level laser therapy17,25,40,41 have been proposed as providing appropriate stimuli for the acceleration of chondrogenesis. Naito et al. studied the effect of LIPUS on cartilage in a rat osteoarthritis (OA) model using serum biomarkers such as CTX-II (type II collagen degradation) and CPII (type II collagen synthesis). CPII was significantly increased in +LIPUS group compared to −LIPUS and the sham control group. In addition, the histological damage on the cartilage (Mankin score) was ameliorated by LIPUS, and type II collagen was immunohistochemically increased by LIPUS in the cartilage of an OA model. Of interest, mRNA expression of type II collagen was enhanced by LIPUS in chondrocytes. Low-intensity pulsed ultrasound affects human articular chondrocytes in vitro.32
Chondrocytes were exposed to LIPUS (30 mW/cm2; 20 min/day, 6 days). Stimulation of [35S]-sulphate incorporation into proteoglycans by LIPUS was 1.3-fold higher in degenerative than in collateral monolayers and 1.9-fold higher in explants. LIPUS increased the number of nests containing four to six chondrocytes by 4.8-fold in collateral and by 3.9-fold in degenerative explants. This suggests that LIPUS stimulates chondrocyte proliferation and matrix production in chondrocytes of human articular cartilage in vitro.42
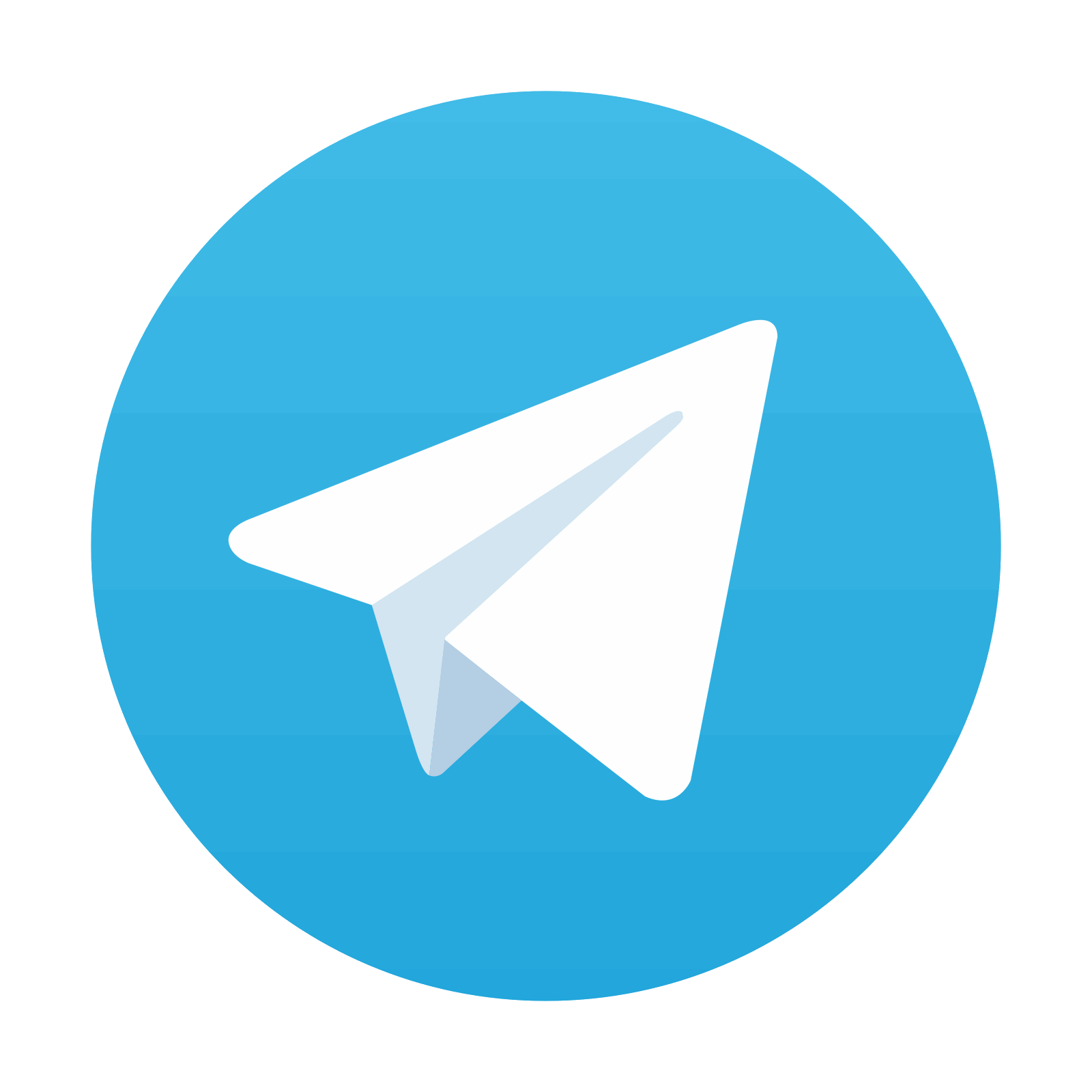