2.4 Physical Therapies
Electrotherapy
Introduction
Since ancient times, it has been known that electricity can be conducted through living tissues and that it can alter physiologic and pathological states. In ad 46, Scribonius Largus used “electric” fish to alleviate gout and chronic headache. In ad 76, Dioscorides of Anazarbos expanded the treatment options to curing anal prolapse and hemorrhoids [Kelloway 1947, Eriksen 1992]. In 1791, Luigi Galvani linked muscle contractions in frog legs to electric shocks, and in the 1800s, Benjamin Franklin used electric shocks to treat pain in his neighbor’s frozen shoulder. This section includes a review of selected principles of electrophysiology, stimulation guidelines, clinical applications for pelvic floor stimulation, case reports, and a chronological summary of pelvic floor research and literature from 1847 to the present day.
Principles of Electrophysiology
The author gratefully acknowledges her indebtedness to Dr. Gad Alon for his lectures, advice, and published works, on which the information provided in this section is based [Alon 1987, 1991]. It is hoped that the information will facilitate the use of electrotherapy for pelvic floor applications and will provide sound physiological principles for clinical decision-making, comprehension of stimulation protocols, and easier navigation through the research literature.
Types of Current, Waveforms, and Terminology [Alon 1991]
Direct current (DC), also known as galvanic current. An electrical current that flows in one direction for about 1 second or longer.
Interrupted DC is used to obtain a twitch contraction from denervated muscle during electrodiagnosis or treatment. A hand switch on the applicator interrupts the DC current (after flow for about 1 s or longer). The interruption lasts about 50–60s and is then switched on again for 1s or longer.
Alternating current (AC). A current that changes its direction of flow (with reference to the zero baseline) at least once every second. Alternating current with no modulation and no intervals between pulses is known as continuous AC.
Typical AC is symmetrical or asymmetrical and can have several shapes, including sinusoidal, rectangular, trapezoidal, and triangular. With AC, there is an inverse relationship between frequency and duration. As the frequency increases, the phase and pulse duration decrease, and as the frequency decreases, the phase and pulse duration increase (Fig. 2.33). AC frequencies are described in Hertz (Hz), or cycles per second.
Pulsatile current. An electrical current that is conducted as a signal, or signals, of short duration—a few milliseconds (ms) or microseconds (ms).
This is used to treat intact peripheral nerves and is distinguished by its waveform. Most, if not all, contemporary clinical electrical stimulators are pulsatile stimulators. Pulsatile current frequencies are described as pulses per second (pps).
Waveforms See also Fig. 2.34.
Monophasic. A monophasic wave has one phase to each pulse. The pulse shape can be square, triangular, twin-peaked, or a half-rectified sine wave. A monophasic wave is unidirectional, indicating positive or negative polarity.
Biphasic. A biphasic wave or AC (alternating current) has a positive and negative phase in a single pulse. Pulse shapes are square, triangular, or sinusoidal—symmetrical or asymmetrical. A current of asymmetrical biphasic pulses is also known as Faradic current.
Polyphasic. A polyphasic pulse contains three or more phases—e.g., “Russian” and interferential current.
Pulse Characteristics
These include phase and pulse duration, phase and pulse intensity, phase and pulse charge, pulse rate (frequency), duty cycle, sweep frequency, and ramping (Table 2.3; Figs. 2.35, 2.36).
Pulse characteristics, parameters | Also known as | Measurement units |
---|---|---|
Phase duration | Pulse duration, phase duration, pulse time, pulse width | Microseconds (us) or milliseconds (ms)* |
Interpulse interval | Interpulse space | Milliseconds (ms) |
Phase or pulse Intensity | Peak pulse, peak current intensity | Milliamperes (mA) |
Duty cycle | Pulse rate, pulses per second (pps), Hertz (Hz, cycles/second) | Seconds (s) |
Ramp | Surge, rise or fall time | Seconds (s) |
Intensity | Current strength | Milliamperes (mA) |
Effects of Electrical Stimulation
Specialized terms used in this section are explained in the glossary given below. Thermal, chemical, and physical effects of electrical stimulation and the resultant physiological changes occur at four levels in the body: cellular, tissue, segmental, and systemic. These effects can be direct (D) indirect (I), or both (D and I). Thermal effects (heat production) occur due to movement, microvibration, and minute frictional forces of charged particles in the conductive medium. Physical effects occur due to the movement of ions, leading to excitation of peripheral nerves (D), which can result in contraction of smooth or skeletal muscle (D or I), activation of endogenous analgesic mechanisms, or vascular responses (both I). Chemical changes are most commonly associated with direct current (DC), due to changes in the molecular configuration of ions.
Changes at the cellular level
- (I) Excitation of peripheral nerves—e. g., the pudendal nerve
- (I or D) Changes of membrane permeability in nonexcitatory cells
- (I or D) Modification of fibroblasts and fibroclastic formation
- (I or D) Modification of osteoblasts and osteoclastic formation
- (Not known) Modification of microcirculation—arterial, venous, and lymphatic (capillary) flow
- (Not known) Alteration of protein and blood cell concentration
- (I or D) Alteration of enzymatic activity, such as that of succinate dehydrogenase (SDH) and adenosine triphosphatase (ATPase)
- (I) Alteration of protein synthesis
- (I or D) Modification of mitochondrial size and concentration
Changes at the tissue level
- (I) Skeletal muscle contraction and its effects on muscle strength, contraction speed, reaction time, and fatigability
- (I) Smooth-muscle contraction or relaxation and its effect on peripheral blood flow
- (I or D) Tissue regeneration in bone, ligaments, and connective and dermal tissues
- (I or D) Tissue remodeling—including softening, stretching, decreasing viscosity, and fluid absorption from joint cavities and interstitial spaces
- (I) Changes in tissue thermal and chemical balance
Changes at the segmental level
- (I) Muscle group contraction and its effect on joint mobility and synergistic muscle activity
- (I) Muscle pumping action; effects on lymphatic drainage and on venous and arterial blood flow in the large circulatory and lymphatic vessels (macrocirculation)
- (I) Alteration of lymphatic drainage and arterial blood flow not associated with skeletal muscle contraction
- (I) When transcutaneous electrical stimulation is applied to the lower urinary tract, a normalization of reflex activity has been reported—a segmental and systemic change [Fall and Lindstrom 1991].
Changes at the systemic level
- (I) Analgesic effects associated with endogenous peptides such as beta-endorphins, enkephalins, dopamines, and dynorphins (may have some potential for treatment of pelvic pain patients)
- (I) Analgesic effects associated with neurotransmitters such as serotonin and substance P
- (I) Circulatory effects associated with polypeptides such as vasoactive intestinal polypeptides (VIP)
- (I) Modulation of internal organ activity, such as bladder function
Modulations of currents may enhance or suppress the respective physiological activities and processes that are affected at each level. Janneck [1976] described a “new” way of treating the neurogenic bladder. Electrical stimulation studies by Godec and Cass [1978] on myelomeningocele using anal electrodes, and on patients with spinal cord injuries [Godec and Cass 1979] using anal and needle electrodes, documented increased bladder capacities in both populations. The parameters for both groups were: 1 ms (1000 ms) phase duration, continuous train of stimulation, frequency 20 Hz, and low intensity—3–8 V (10– 15 mA). Stimulation was “on” continuously at the low amplitude described, except for voiding and a pause every 2 h for passing flatus [Godec and Cass 1978]. Higher amplitudes (100–150 mA) were used in the spinal cord injury population, because of the sensory loss [Godec and Cass 1979].
Direct and Indirect Effects; Excitatory and Nonexcitatory Responses
There is always a direct effect at the cellular level under the stimulating electrode, regardless of the current. This direct effect can extend to the tissue level if DC current is used or if a monophasic waveform with a very long phase duration (10–300 ms/10,000–300,000 ms) is used, or with symmetric biphasic or polyphasic pulses if the current density is excessively high. Indirect effects are defined as those physiological reactions or responses that are triggered by the direct effects and may occur at or remote from the area where the current flows (Table 2.4).
Excitatory responses of peripheral nerves to stimulation are perceived as sensory, motor, and pain (Table 2.5). An example of a non-excitatory response to electrical stimulation is bone tissue remodeling, used to facilitate fracture healing.
Terminology of Electrical Stimulation
Transcutaneous electrical nerve stimulation (TENS). In the United States, the TENS device is associated with pain modulation. However, since the mode of operation and pulse characteristics of pulsatile stimulators that use surface electrodes to stimulate peripheral nerves involves transcutaneous electrical stimulation of nerves, all pulsatile stimulators—Russian stimulators, dedicated pelvic floor neuromuscular electrical stimulators, and interferential stimulators, including pain control stimulators—are transcutaneous electrical nerve stimulators. If one accepts this broad model, it may minimize confusion and multiple decisions about which electrical equipment to use or purchase. Physiological outcomes such as “improved circulation,” “retarding muscle atrophy,” “reeducating pelvic floor musculature,” “healing tissue,” “relieving pain,” “converting fasttwitch muscle fibers to slow-twitch muscle fibers,” etc., would not be a function of a particular brand of stimulator—e. g., TENS, interferential stimulator, or high-voltage galvanic stimulator— but rather a function of the pulses, pulse characteristics, waveforms, and parameters available on many pulsatile “transcutaneous electrical nerve stimulators” (using the broad definition) (Table 2.6). Although a pain-modulation TENS unit does not have a duty cycle as neuromuscular and other pulsatile stimulators do, it could be used in certain pelvic floor applications by manipulating the available parameters of phase duration, waveform (if available), rate, and intensity. It could be applied for low-intensity chronic stimulation (see p. 189).
Direct effect | Indirect effect | |
---|---|---|
TENS for pain control | Cellular level: Excitation of peripheral sensory nerve cells | Systemic level: Release of endogenous analgesic substances—endorphins, enkephalins, serotonin |
Neuromuscular stimulation to treat muscle atrophy | Cellular level: Excitation of motor and sensory fibers of peripheral nerves | Tissue level: Contraction of skeletal muscle fibers Segmental level: Contraction of the whole muscle or muscle group if enough motor units are activated |
TENS: Transcutaneous electrical nerve stimulation.
Electrode placement. Two leads are placed at S2– S4 on the back and two over the perineal body or perianally.
Current. Continuous or modulated, depending on the characteristics of the unit; phase duration 100–250 ms, and intensity to sensation only (see the contact information for V. Richard given at the end of the reference list below).
Electrodes
Size, shape, and placement. Both surface and internal electrodes come in a variety of sizes and shapes. Each of these factors can affect the current density [Cook 1987, Cook and Barr 1991]. Current density is the amount of current flow per unit area. It is a measure of the quantity of charged ions in milliamperes per square centimeter or per square inch. It is an important factor in determining the reaction of biological tissues to stimulation. The greater the current density, the greater the effect on the tissues. The two determinants of current density are the amount of current applied and the size of the area of application (Table 2.6).
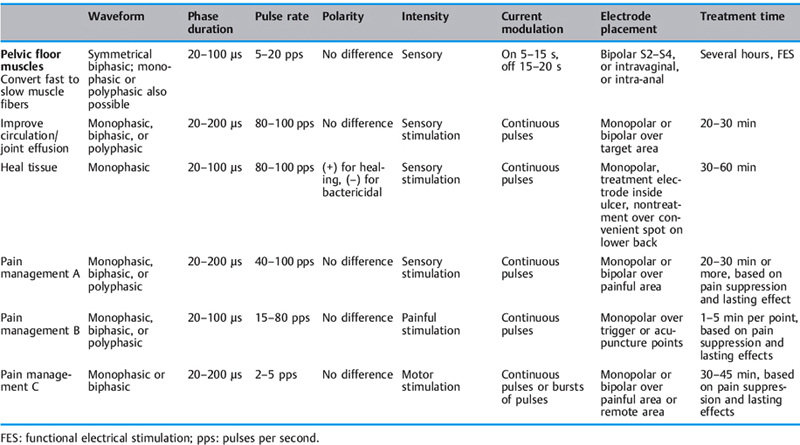
Size |
|
Placement |
|
Tissue impedance |
|
Electrode coupling |
|
Vaginal application. Illustrations of internal electrodes of varying sizes and shapes to accommodate different dimensions of the vaginal and/or anal canal can be found in the literature [Sotiropoulos 1975, Sotiropoulos et al. 1976, Suhel 1976, Fall et al. 1986, Ohlsson and Erlandson 1988, Eriksen et al. 1989]. In a 1984 study, Magnus Fall used an inflatable vaginal electrode “so that the carrier can be secured in the proper position within the vagina to retain the electrodes in individual optimal positions. The inflatable device also has the great advantage of minimizing mechanical discomfort.” Well-designed electrodes that deliver a current that is tolerable to patients are essential. Two important principles of electrodes and electrode placement are size and contact. Comfortable or uncomfortable perception of current is a direct result of size and contact. These considerations are especially important for applications in the rectum or vagina. Intimate contact within the rectum or vagina, at the appropriate level (of the pelvic floor musculature) [Kegel 1948, 1952, Benzl 1992, Laycock 1994] is essential for the patient to tolerate the sensations produced by the electrical current. Ideally, one envisions an electrode that would be adjustable to accommodate the most narrow or capacious canal. In the absence of the ideal device, the author recommends a handheld electrode that can be manually positioned on each vaginal wall during the course of the stimulation treatment. The popular tampon-style electrode is convenient, but should only be used by patients who have enough tone in the vagina to retain it in place. It has been noted in the author’s clinical practice that greater current intensity is required to achieve a pelvic floor muscle contraction using a tampon-style electrode. When a hand-held electrode is positioned against the vaginal wall, the contraction is achieved with less current intensity. Patients who have used both styles have stated that the hand-held electrode is more comfortable. While a teaching video was being made in 2001, the model remarked that the current sensation with the extended-handle electrode (held directly against the respective vaginal wall) was more tolerable than the tampon electrode she had previously used.
Rectal application. Since a rectal electrode will not be hand-held, its surface area and shape are equally important for maintaining intimate contact with the rectal walls. Using narrow electrode bands (Table 2.7) on a small diameter or thin electrode is a formula for discomfort for male patients or females who require intra-anal placement. An example of a well-fitting electrode with a unique size and shape is the Anuform (Fig. 2.37). In summary, the ideal electrode would accommodate the vagina or rectum completely.
Pelvic floor muscle response to electrical stimulation. Evidence of pelvic floor muscle contraction is described as elevation of the perineum or anus, anal wink, perineal shrug, or vulval movement [Riddle et al. 1969]. When a hand-held electrode is used, there is excellent feedback to the patient and/or therapist, in that the electrode moves in reaction to the contraction of the pelvic floor muscle.
Clinical Stimulation Units/ Pulsatile Current Units
Neuromuscular electrical stimulators. These are TENS used for neuromuscular reeducation and other clinical applications. Clinical models are usually programmable and have multiple parameter settings. Dedicated units have fixed parameters, and programming is limited. Dedicated pulsatile stimulation units can be used in the clinic and at home. Their typical waveform is symmetrical biphasic, with fixed parameters of 200–300 ms phase duration and a duty cycle of 5 s on and 10 s off. There is usually a choice of two frequencies—“urge” and “stress”—with the former being set at 10–12.5 pps for reflex inhibition of the detrusor or urge incontinence [Godec et al. 1975, 1976, Erlandson et al. 1977a, Lindstrom et al. 1983, Vodusek et al. 1988] and the latter at 40–50 pps for muscle strengthening or stress incontinence [Moore and Schofield 1967, Teague and Merrill 1977, Fall et al. 1978, Plevnik et al. 1986]. All of the dedicated units used in the United States are manufactured with these specifications, since the Food and Drug Administration (FDA) approval of these stimulators was based on these parameters. To manufacture and sell a product for pelvic floor stimulation with different parameters, a manufacturer would have to undertake the lengthy and arduous process of seeking FDA approval.
Interferential stimulator. This is a type of TENS that was devised in order to reduce the discomfort perceived by patients due to the intensity of the low-frequency current required to activate deep seated muscles. It can be described using as a type of amplitude-modified AC. Two medium-frequency currents (e. g., 2000–5000 Hz) are used in such a way that they flow in a crossed fashion. At the point of intersection, the total current applied to the patient is the difference between the two currents; 35 Hz is produced by the interaction of 4000 and 4035 Hz. It is a continuous current with a multiphasic waveform. The phase duration is 125 ms, the pulse rate is 1–200 pps, and the total current is 80–90 mA. The electrode configuration can involve four in a criss-crossed pattern or may be bipolar.
Russian stimulator. This is a TENS in which a continuous sine wave of about 2500 Hz is modulated to yield 50 bursts per second. Each burst is a polyphasic pulse waveform. The current mode is interrupted. The phase duration is 200 ms, adjustable, the pulse rate is 1–100 pps, and the total current is 100 mA.
High-volt pulsed galvanic (HVPG) devices. This is a TENS with a pulsatile waveform that is monophasic and twin-peaked. The current intensity is high, at 2000–2500 mA, with very short phase duration and a very high peak. The current mode is continuous/interrupted. The phase duration is 1–20 ms, the pulse rate is low at 25 pps, and total current is very low, reaching a maximum of 1.2–1.5 mA. This TENS generally does not have a duty cycle. The current sensation is relatively comfortable and can be used for sensory, motor, and pain-conducting nerve fibers.
Pain-relief TENS. This is a pulsatile current stimulator that does not have a duty cycle. The pulsatile current is modulated by frequency, width, and amplitude, etc., so as to provide the “best” stimulation of peripheral nerves with the least accommodation of the patient [Barr 1991].
Ultrasonography. Although ultrasound devices are not pulsatile stimulators, they are electrical devices that are present in almost all physical therapy clinics. They can be used for perineal application—for example, prior to using soft-tissue techniques on painful episiotomy scars. Since sound waves can be transmitted through a latex condom, a condom may be used over the transducer to protect the patient and the equipment. (Before treatment, the therapist has to determine whether the patient is allergic to latex.) Ultrasound gel is applied to the transducer before putting the condom over it, and more gel is then applied to the condom-covered transducer. The treatment proceeds as usual. The therapist needs to ensure that there is good coupling, so additional conductive gel should be handy. The pulsed mode is usually recommended in order to minimize heat build-up, because of the small total area of the perineum. After treatment, the transducer should be cleaned with an appropriate disinfectant.
Other Electrical Stimulation Systems
Magnetic stimulation is one of the more recent technological innovations. Extracorporeal magnetic innervation (ExMI, Neotonus, Inc., Marietta, Georgia, USA; www.neotonus.com) uses technology based on Faraday’s discovery in the mid-1800s that muscular contractions could be induced by the application of a time variable magnetic field. ExMI technology has created a delivery system for a focused magnetic field that penetrates into the perineal musculature. The therapy “head” is set into the base of a comfortable easy chair. This therapy head generates a magnetic field that creates an electrical potential, causing an ion flow in the tissues. The ion flow results in depolarization of resting motor neuron membrane potentials. Action potentials are initiated and propagate along the axion via the Na+ and K+ ion flows. The musculature of the pelvic floor responds with a contraction equal to the output pulse rate of the magnetic field source. The magnetic field induces nerve impulses, which cause muscle contractions and increase circulation. The noninvasive nature of this therapy is attractive, as the patient can sit fully clothed on the treatment chair over the magnetic field generator to receive treatment. Sand et al. [1995] concluded that 6 weeks of bi-weekly, 20-min ExMI treatments provided effective therapy, as assessed by the patients’ voiding diaries at 2 weeks and 18 weeks after therapy. They noted that this “long-term” response is not seen after physical therapy or pelvic floor stimulation. Patients without risk factors respond best, with cure rates of 44% and 43% at 8 and 24 weeks, respectively. In a 2000 update, Galloway et al. concluded that ExMI was equally effective for stress and urge incontinence, but that the best results were achieved in patients who use no more than three pads per day and have had no previous incontinence surgery [Galloway et al. 2000]. In 2000, Bavendam studied the impact of ExMI on the incontinence quality of life (IQOL) in the treatment of stress urinary incontinence and found a high correlation between increased IQOL scores and positive changes in the number of leaks, pad changes, and urinary frequency [Bavendam 2000]. Yamanishi et al. [2000] compared magnetic with electrical stimulation in the inhibition of detrusor overactivity. The authors concluded that both treatments were effective, but that the inhibition of detrusor overactivity appeared to be greater in the magnetic stimulation group. Unsal et al. studied extracorporeal magnetic stimulation in a group of 35 patients with stress incontinence and 17 patients with urge incontinence. The treatments lasted 20 min (10 min at 5 Hz and 10 min at 50 Hz) twice a week for 8 weeks. After 1 year of follow-up, 11 patients with stress incontinence and six with urge incontinence were cured.
Sacral neurostimulation. Mild electrical impulses are delivered through electrodes that are surgically implanted into the sacral nerve roots. The result is modulation of nerve responses to restore more normal reflex control of bladder function. The indications for this form of treatment are urinary urge incontinence, nonobstructive urinary retention, and symptoms of urgency–frequency. A test stimulation is first done with percutaneous insertion of needle electrodes near the appropriate sacral nerve root. The external lead is connected to an external test stimulator. If the response is appropriate, then the permanent stimulator and leads can be implanted [Schmidt et al. 1990, Schmidt 1992, Schmidt and Doggweiler 1998, Tanagho 1998] (further information is available at www.medtronic.com.
Intermittent percutaneous tibial nerve stimulation (PTNS) was also studied in a 12-week prospective multicenter study of 33 patients with chronic pelvic pain. Modest success rates were achieved using a visual analogue scale (VAS) for pain and the McGill pain questionnaire. In seven patients (21%), the mean VAS decreased by more than 50%; in six patients (18%), the decrease was more than 25 %. After 12 weeks of treatment, seven patients (21%) had a VAS of less than 3. PTNS may have a place in the treatment of patients with chronic pelvic pain who have tried other therapies and have few treatment options left [Van Balken et al. 2003].
Sympathetic therapy system (STS). The technology uses electrical stimulation to treat the sympathetic nervous system. This is achieved by providing systemic rather than local treatment. To gain access to the autonomic nervous system through the peripheral nerves, electrodes are attached bilaterally to the upper and lower extremities or the lumbar or cervical spine. Eight paired channels are used. STS is being marketed as a treatment for intractable pain, for diagnoses such as reflex sympathetic dystrophy [Kleinkort 2001, Guido 2002]. There might be an application for this form of treatment in patients with chronic vulvar pain (further information is available at www.ChronicPainRx.com.
Microcurrent. Microcurrent is a current of less than 1 mA. Any electrical device that produces less than 1 mA of current is a microcurrent stimulator. Proponents of microcurrent therapy believe that it helps stimulate an electric field at the cellular level to enhance circulation. It has largely been used in wound healing [Wolcott et al. 1969, Rowley et al. 1974, Gault and Gatens 1976, Carley and Wainapel 1985]. Other reported clinical uses include relief of pain in sports injuries. The mode of action could be described as occurring at the cellular level.
Pelvic Floor Stimulation and Clinical Applications
Neuromuscular Electrical Stimulation for Incontinence and Pelvic Floor Applications
Electrical stimulation provides an effective therapy adjunct for stress urinary incontinence due to sphincter weakness, and for urge incontinence (overactive bladder) due to uninhibited bladder contractions. Indications for the use of electrical stimulation for the pelvic floor are shown in Table 2.6 and Fig. 2.38. The principle of electrical stimulation is based on the restoration of normal physiological reflex mechanisms in abnormal nerves and muscles. The main functions of the lower urinary tract are storage and emptying of urine. Pelvic floor, bladder and periurethral muscles control these functions and are coordinated by balanced nervous system reflexes and voluntary control. The application of external electrical impulses to the neural regulatory system improves this control and is achieved through stimulation of the pudendal nerve [Bergman 1985, Eriksen 1992].
Electrical stimulation for incontinence. Many recommendations for stimulation parameters are found in the literature. They include a wide range of intensities, phase durations, and frequencies. Generally, the lower frequencies of 5–20 pps are recommended for urge incontinence, with 40–60 pps recommended for muscle strengthening [Glen 1971a, Plevnik and Janez 1979, Fall 1984, Laycock et al. 1994] (Table 2.8). Two methods of electrical stimulation are described in the literature: acute maximum/ high-intensity stimulation and chronic/low-intensity stimulation.
Acute maximal stimulation. Applied with a pulsatile stimulator, up to 120 mA, as tolerated, for 10–30 min, one to three times per week for several weeks. Eriksen’s acute maximal electrical stimulation study [Eriksen et al. 1989] used a pulsatile stimulator with the following parameters: monophasic square pulse, frequency 5–10 Hz, cyclic sweep 5–10 Hz or 25–50 Hz, 1 ms (1000 ms) phase duration and duty cycle of 1.5s on and 3s off. Eriksen recommended amplitudes of 65–120 mA. The patients in the study tolerated an average current intensity of 86 mA. Long phase durations cause discomfort to patients. In earlier studies with maximal stimulation, patients were anesthetized. Moore and Schofield [1967] used faradic surges in patients under anesthesia. A faradic surge consisted of pulses of 1ms (1000 ms), with the whole surge lasting 2–3s. After pause of 2–3s, the surge was repeated. The intensity of the stimulation was increased until there was a maximal contraction of all the voluntary musculature. From four to six maximal contractions were produced in each patient. According to Plevnik et al. [1986], symmetrical biphasic waveforms with a phase duration of 100–300 ms are suitable for pelvic floor electrical stimulation, as they are best tolerated, and these are used today.
Stress urinary incontinence | Urge incontinence | |
---|---|---|
Parameters |
|
|
Results |
|
|
Chronic low-intensity stimulation. Intensity is to sensory perception only, at 10–20 pps. This is usually used in the home setting, up to several hours daily or overnight, for several months. It is referred to as “trophic” stimulation in the United Kingdom and as “functional electrical stimulation” (FES) in the USA [Plevnik et al. 1986]. Buller et al. [1960] found that reinnervation of a slow-twitch or fasttwitch muscle with a nerve normally supplying a slow-twitch or a fasttwitch muscle, respectively, leads to a transformation of that muscle’s phenotype. Salmons and Vrbova [1969] demonstrated that transformation of a fasttwitch into a slow-twitch muscle can be brought about without altering innervation by transmitting to the nerve of the fasttwitch muscle, through implanted electrodes, a mean frequency pattern that is normally delivered by action potentials to a slow-twitch muscle. These physiological phenomena were first described in the animal model and then were developed for human electrotherapy [Kidd and Oldham 1988a, 1988b]. This property of skeletal muscle is adaptive plasticity, or the ability to change in molecular form to match a desired motor function [Salmons and Vrbova 1969, Brown et al. 1976, Scott et al. 1985, Kidd and Oldham 1988b, Jeyaseelan and Oldham 2000, Jeyaseelan et al. 2000]. Studies on the electrical stimulation of gracilis muscle perineoplasties for anal sphincter incompetence use the principle of conversion of fatiguable muscle to fatigue-resistant muscle [Bouamrirene et al. 2000, Zonnevijlle et al. 2000, Baeten et al. 2001].
Pelvic floor strengthening. Laycock [1994] describes three theories of muscle strengthening using pelvic floor electrical stimulation.
- Theory 1. Employs maximum stimulation and proposes that muscle strength is increased by repeatedly “overloading” the pelvic floor muscle, with repeated maximum contractions in a manner similar to voluntary exercise.
- Theory 2. Employs maximal stimulation and proposes that with electrically induced contractions, type 2 (fasttwitch) muscle fibers are more effectively targeted and trained than with active exercise only. It suggests that improved reflex (fast) responses would be a result of maximum neuromuscular electrostimulation treatment.
- Theory 3. Employs chronic low-intensity stimulation. These parameters support the concept that FES may modify the physiological and metabolic characteristics of normal muscle and muscle atrophied by disuse. The chronically stimulated muscle “switches” to slow twitch, increasing the endurance.
Clinical Application/Techniques of Pelvic Floor Electrical Stimulation
Indications. The clinical decision to incorporate electrical stimulation into a therapy program should be dependent on the state of the pelvic floor as determined in evaluation, plus diagnosis and symptoms. Many practitioners experienced in pelvic floor rehabilitation agree that electrical stimulation should not be used just because it is available. They consider that educating patients is the first approach, so that they can learn and understand the correct pelvic floor muscle contractions. On the basis of the strength and control of the pelvic floor muscles, a functional pelvic floor exercise program should also be initiated. There are, however, certain indications for using electrical stimulation as a first-line intervention. Three significant indicators are an inability to elicit a contraction of the pelvic floor muscles, weak muscles trace to 2 over 5 strength (2/5), and poor or absent proprioception of pelvic floor muscle activity. Some patients may also benefit from stimulation to augment voluntary contractions. During stimulation, it is important to know whether contraction of the pelvic floor musculature is occurring. This can be easily observed and felt if the therapist is holding a long-handled electrode. If a tampon-style electrode is being used, pelvic floor muscle contraction will cause movement of the wire as the perineum elevates. Sometimes no elevation is noted with this electrode. Muscle activity can then be assessed with a gloved finger placed on the electrode within the vagina. If electrical stimulation is used as a treatment, its period of use may be temporary or cyclical, as needed. The duration of favorable muscle or bladder changes secondary to electrical stimulation varies. Many studies recommend or note that repeat treatment periods may be necessary to maintain continence after it has been restored [Eriksen and Eik-Nes 1989, Jonasson et al. 1990, Fall and Lindstrom 1991]. This view is corroborated in the author’s clinical observation of certain cases, with patients reporting they were still using their home stimulators as needed (after the recommended initial home treatment period was over).
Equipment. The physical therapist will probably use dedicated hand-held units or clinical units. As mentioned earlier, the clinical unit could be any type of TENS, including high-volt galvanic stimulation, interferential stimulation, neuromuscular electrical stimulation, or pain-relieving TENS. An excellent randomized controlled study on the effect of behavioral training with or without pelvic floor electrical stimulation on stress incontinence in women was conducted between October 1995 and May 2001 on a voluntary sample of 200 non-demented community-dwelling women aged 40–78 with stress or mixed incontinence (stress being the predominant pattern) [Goode et al. 2003]. The patients were randomly assigned to 8 weeks (four visits) of behavioral training, 8 weeks of behavioral training plus home pelvic floor electrical stimulation, or 8 weeks of self-administered behavioral treatment using a self-help booklet. The overall results showed that incontinence was reduced by a mean of 68.6% with behavioral training, 71.9% with behavioral training plus electrical stimulation, and 52.5% with the self-help booklet. Overall, treatment with pelvic floor electrical stimulation did not increase the effectiveness of a comprehensive behavioral program for women with stress incontinence. A self-help booklet reduced incontinence and improved the quality of life, but not as much as the clinic-based programs. In their comments on the study, the authors state “current evidence suggests that the two technologies (pelvic floor electrical stimulation and biofeedback) may overlap in function. Either may improve the results of pelvic floor muscle exercise training, but both technologies are apparently not needed in the same protocol for most women with stress incontinence. It is possible that neither technology is needed as a part of the initial therapy for urinary incontinence, but should be reserved for patients who do not improve with a less invasive behavioral program” [Goode et al. 2003]. This opinion would be consistent with the philosophy of experienced practitioners regarding the arbitrary use of a modality just because it is available.
Procedure for intravaginal or intra-anal electrical stimulation. The following (adapted from Hakeem [1997, 2002]) is a guide from a hospital outpatient clinic setting on the use of pelvic floor electrical stimulation. Before the treatment is started, a thorough pelvic floor evaluation must previously determine whether electrical stimulation is appropriate for the patient.
(Note “*”: If a “declicated” device is used, the available frequencies are 12.5–20 pps and 40– 60 pps. Clinical units, will allow more variability of parameters.)
Treatment objectives.
- To facilitate contraction of the levator ani and pelvic floor muscles (pubococcygeus, puborectalis). Set the frequency to 50 pps.*
- To enhance the contractions of a weak muscle (strength of 0–2+/5Y on a scale of 0–5) so that the client can perceive a contraction. (This is neuromuscular reeducation to increase pro-prioception.) Set the frequency to 50 pps and gradually increase the amplitude until a contraction is achieved with a current that is tolerable to the patient.
- To decrease urgency in patients with urge incontinence or overactive bladder. Set frequency low, 5–20 pps.*
- To “fatigue” a muscle spasm. Set the frequency in the range of 50–100 pps.*
- To decrease intravaginal or intra-anal pain by creating a “TENS”-like effect. Set the frequency in the range of 80–100 pps.*
- Intravaginal, intra-anal electrode, or surface electrodes.
- Appropriate electrical stimulator: clinical model, hand-held portable, or dedicated unit.
- Conductive water soluble lubricant: e. g., KY jelly, Surgilube.
- Gloves for the therapist.
- Disposable pad (blue pad) under the patient’s buttocks.
- Mirror available for perineal observation.
Procedure
- Explain the rationale for the treatment to the patient.
- Explain the range of sensations created by the current.
- Set up the equipment to be used.
- Place the patient in propped supine position, with pillows supporting the legs. The patient may have to reposition slightly to render the perineum visible for evaluation and observation.
- Note the appearance of the perineum and check for sensation.
- Apply gel to the rectal or vaginal electrode.
- Insert vaginally or rectally to the appropriate depth.
- To establish the appropriate current amplitude for treatment, turn the unit on and slowly advance the amplitude until the patient perceives the current sensation. Continue slowly advancing the amplitude to the patient’s comfort or until a contraction of the levator ani (pelvic floor) is elicited. A contraction will be observed as an elevation of the perineum. Constantly ask the patient if it is all right to turn up the current. A general rule: attempt to achieve the best muscle contraction at a current that is completely tolerable to the patient.
- – Note: If a contraction is not readily apparent, it is appropriate to continue the stimulation at sensory level until the patient is comfortable with increasing the amplitude.
- – If an extended handle vaginal electrode is being used, moving the electrode slightly a few millimeters in an anterior, posterior, superior, or inferior direction will result in better contact with the vaginal wall nerve endings, and a better muscle contraction will result.
- – The use of an extended-handle vaginal electrode allows excellent electrode contact with the area (see electrode sizes and contact, pp. 182, 184).
- – Note: If a contraction is not readily apparent, it is appropriate to continue the stimulation at sensory level until the patient is comfortable with increasing the amplitude.
- Set the recommended duty cycle and begin the treatment.
- During the stimulation treatment, you may ask the patient to actively co-contract the pelvic floor muscles with the stimulation.
- It is important not to fatigue the muscles. Unfortunately, one often does not know that the muscle is becoming fatigued until symptoms of increased leakage and less control of leakage appear.
Points of emphasis
- Equipment dispensed to patients in the United States must have received FDA approval.
- Electrical stimulation must be carefully managed.
- – With electrical stimulation, motor units fire synchronously and the muscle contracts maximally. Muscle fatigue is a distinct possibility and should be avoided when prescribing and using electrical stimulation.
- – The patient must be cognitively aware and able to manipulate the electrodes and controls.
- – The patient must be able to follow specific written or illustrated instructions provided by therapist.
- – With electrical stimulation, motor units fire synchronously and the muscle contracts maximally. Muscle fatigue is a distinct possibility and should be avoided when prescribing and using electrical stimulation.
- The electrode is either hand-held (extended handle), or (if insertable) should be securely placed and not at risk of slipping out.
- Electrical stimulation for home use should be under the supervision of a physical therapist and easy communication with the physical therapist should be available.
Contraindications
Do not use electrical stimulation in the following circumstances:
- If the patient is or may be pregnant.
- If the patient is menstruating.
- If the patient has an active vaginal or bladder infection.
- If the patient has a pacemaker.
- Over metal implants.
- In the presence of a known malignancy.
Case Reports
Case report 1
A 79-year-old man who had undergone a radical prostatectomy was experiencing continuous leakage, with no control over urination and no improvement in the symptoms over time. After an extensive literature review of current and past studies on electrical stimulation for the treatment of urinary incontinence, the patient, a bioelectrical engineer, designed his own stimulator. The parameters he used were pulse frequencies of 10 and 50 Hz, a phase duration of 300 ms, and a duty cycle of 5 s on and 5 s off. Adhesive TENS-type electrodes were used on the back at S3 and at the base of the scrotum on the perineal body. Intensity was advanced to just threshold sensation levels for 10 min at each frequency. The treatment frequency was every other night until he began to achieve control (about 3 weeks). He now uses electrical stimulation as needed—for him, once a week. Before the stimulation treatments, he used multiple pads each day and now only needs to use one. From his experience, he feels that continence can be maintained using a standard (pain) TENS. Parameters: 50 and 10 pps, 300 m s (or maximum) phase duration, and current mode “M” for modulated. For some therapists and patients, a “dedicated” unit may be more effective as a first-line stimulation experience. From his own experience, he is adamant about not fatiguing the pelvic floor muscles. He feels that electrical stimulation often fails because intensities are too strong or the duration of the stimulation per treatment is too long (see the contact information for V. Richard given at the end of the reference list below).
Case report 2
A 72-year-old man who had been a licensed engineer by profession underwent a radical prostatectomy in 1992. His incontinence symptoms were steady dripping all day, from the time the postsurgical catheter was removed. There was no active control over the steady daytime dripping, but he was usually dry at night. He managed to contain the dripping with a combination of protective pads and a penile clamp. He questioned his surgeon regarding electrical stimulation of the urinary sphincter and was told that this was not possible and that he would “get better.” Still seeking answers and solutions for his leakage problem, he had diagnostic urodynamic testing in 1993. Although he was relieved that his lower urinary tract was normal, he was dismayed to be told there was no therapy for incontinence except the artificial urethral sphincter. He found a nurse-practitioner in early 1994 who provided conservative management of incontinence and on March 29, 1994 he started electrical stimulation using an anal electrode.
Table 2.9 summarizes the course and results of his electrical stimulation treatment. Conclusions to be drawn from these data:
- Pavlovian conditioning worked in this patient’s case.
- Randomized delivery of pulsed current appears to be superior to repetitive timed cycles.
- The establishment of an optimal stimulation dose is vital. Low amplitudes appear to be successful.
- An argument is clearly made for not fatiguing the pelvic floor muscles.
Case report 3
A 54-year-old woman was diagnosed with stress urinary incontinence and weak pelvic floor muscles. A check-up after 10 years revealed that the patient was still using electrical stimulation as needed. She reported that she judged by her leakage pattern when she could stop daily treatments and when she needed to resume.
Parameters: symmetrical biphasic waveform, phase duration 250 m s, 40–60 pps, duty cycle 5 on, 10 off. The intensity used was only sufficient to produce a tolerable contraction of pelvic floor muscles. The patient used a hand-held electrode and did 10 contractions on each vaginal wall, right, left, and posterior. Treatment time: 10–15 min every other day.
Conclusions
It is theorized that in the male patients, tonic musculature was being enhanced or fasttwitch muscle was being converted to slow-twitch muscle, thus facilitating the internal sphincter. The female patient experienced benefit from the direct effects of stronger pelvic floor contractions and a more efficient urethral closure mechanism.
Historical Citations and Current Research
Early History of Pelvic Floor Electrical Stimulation
See also Table 2.10 and the contact information for V. Richard given at the end of the reference list below.
Articles on pelvic floor electrical stimulation appeared in the literature from the late 1800s through the 1950s. Between 1863 and 1880, six studies on pudendal nerve stimulation were pub lished. The authors were physicians from the United Kingdom, Scandinavia, and the United States. In earlier reports (1878–1924), electrodes were placed in the bladder or urethra [Saxtorph 1878, Somerville 1923]. In a paper delivered to the Obstetric Section of the New York Academy of Medicine, Furniss stated that “galvanism or faradism of the urethra with one electrode in the urethra and a large indifferent one over the sacrum is at times beneficial.” Bathurst [1929] observed that the status of atrophied sphincter musculature is greatly improved by the application of electrical stimulation. Clayton, consulting physician to the physiotherapy department at Kings’ College Hospital, London, recommended strengthening abdominal and pelvic floor muscles with surged faradic current “to cause contractions of the levator ani and urinary sphincter” [Clayton 1949]. On the basis of a “theory that individuals unable to control their sphincter muscles might be able to develop sphincter muscle strength and initiate volitional contraction by means of electrical stimulation,” Huffman et al. [1952] studied 17 patients with stress incontinence in 1952. In 1955, Hudgins successfully used this protocol in a stress-incontinent patient in whom two operations and Kegel exercises had failed. He also used electrical stimulation on patients with stress incontinence, prolapse, pelvic pain/localized vaginal tenderness, genital relaxation, coccydynia, and dyspareunia [Hudgins 1955]. The same principles of neuromuscular reeducation that are followed today were already evident in the parameters and study designs used by Huffman et al., Clayton, and Hudgins.
Later Twentieth Century Research to Present Day
Descriptive trials during the 1960s and 1970s attempted to determine the effect of stimulation on different types of incontinence. Investigators began to explore electrical stimulation techniques and means of delivery for the treatment of voiding disorders such as stress urinary incontinence, urge incontinence, urinary retention, post-prostatectomy incontinence, and neurogenic bladder. Caldwell [1963, 1968] and Alexander and Rowan [1968] used electrodes that were implanted via a retropubic approach directly into the pelvic floor in women with fecal or urinary incontinence. The current delivered caused tetanic contractions and resulted in a high failure rate [Caldwell 1963, Moore and Schofield 1967, Caldwell 1968, Caldwell et al. 1968, Alexander et al. 1970, Harrison and Paterson 1970, Bergman 1985]. Because of migration problems with the implantables, inconsistent results, and lack of success, alternative delivery systems were later tested. In the 1960s and into the 1970s, non-implantable electrodes, electronic pessaries [Alexander et al. 1970, Harrison and Paterson 1970] and anal plug electrodes [Glen 1971b] appeared. These electrodes delivered continuous current, which caused muscle fatigue and resulted in less than optimal clinical results [Alexander and Rowan 1968, De Soldenhoff and McDonnell 1969, Hopkinson and Lightwood 1969, Bergman 1985]. Although studies with implantable electrodes were still being published in the 1970s, nonimplantable anal and vaginal electrodes began to take precedence [Doyle et al. 1974, Sotiropoulos 1975, Sotiropoulos et al. 1976].
Studies published in the 1970s by researchers such as Godec, Cass, and Fall were creative in the ways in which they used stimulation. For bladder inhibition, two methods were used: high inten sities (maximal stimulation) with the patient anesthetized, and long-term chronic stimulation for up to 12 h a day [Fall et al. 1977]. Fall’s studies in the late 1970s focused on the effectiveness of stimulation for bladder inhibition and recommended frequencies for inhibition and strengthening [Fall et al. 1977, 1978, Erlandson et al. 1977b, Plevnik and Janez 1979].
Trials during the 1980s were concerned with optimal parameters for electrical stimulation [Godec et al. 1981], explaining the neurophysiological basis for bladder inhibition [Lindstrom et al. 1983], and linking electrostimulation with control of stress and/or urge incontinence [Nakamura et al. 1983]. Papers were also published that examined conduction velocity values in patients with idiopathic fecal incontinence and in patients with damage to the periurethral sphincter musculature [Kiff and Swash 1984, Snooks et al. 1984]. Different pulse parameters were being studied [Ohlsson and Erlandson 1988]. Early articles that eventually culminated in the development of sacral neurostimulation through implanted electrodes were written by Tanagho [1988] and Vodusek et al. [1988]. In 1989, Griffith described the muscles and nerve supply of the pelvic floor and the effects of electrical stimulation on the nature, magnitude, and sequence of contractions [Griffith 1989]. Eriksen et al. [1989] published studies on maximal stimulation. The Eriksen study was used as evidence to support reimbursement for electrical stimulation for incontinence in a landmark Health Care Financing Administration (HCFA) hearing in the United States in 2000.
Many studies were published in the 1990s as electrical stimulation became known and used throughout the world. Berghmans et al. [1998] published a systematic review of 24 randomized clinical trials to assess the efficacy of physical therapies for first-line use in the treatment and prevention of stress urinary incontinence (SUI). The conclusions reached were that there is strong evidence to suggest that pelvic floor muscle exercises are effective in reducing the symptoms of SUI; that there is limited evidence for the efficacy of high-intensity exercise in comparison with low-intensity exercise; and that there is no evidence that pelvic floor muscle exercises with biofeedback are more effective that pelvic floor muscle exercises alone. With regard to electrical stimulation studies, the authors found little consistency among the stimulation types and parameters used, but when the results were combined there was strong evidence to suggest that electrical stimulation is superior to sham stimulation.
In 2000, electrical stimulation and biofeedback were approved as interventions in the Medicare population by a Medicare Coverage Advisory Committee of the Health Care Financing Administration. Hearings took place in Baltimore in April 2000. A variety of practitioners, including the American physical therapists Cynthia Feldt and Dr. Julie Pauls, presented arguments in favor of reimbursement for electrical stimulation and bio-feedback treatments. Many trials conducted in the late 1980s and 1990s were used in the body of supportive evidence for electrical stimulation that was presented [Bergmann and Eriksen 1986, Eriksen and Mjolnerod 1987, Eriksen 1990, Blowman et al. 1991, Esa et al. 1991, Meyer et al. 1992, Zollner-Nielsen and Samuelsson 1992, Bent et al. 1993, Caputo et al. 1993, Laycock and Jerwood 1993, Sand et al. 1995, B⊘ and Maanum 1996, Richardson et al. 1996]. In 2000, in a systematic review of the incontinence literature, Berghmans et al. examined studies of conservative treatment of urge urinary incontinence in women, published between 1980 and 1999 [Berghmans et al. 2000]. Fifteen randomly controlled trials were identified. Based on the levels of evidence, the stimulation types and parameters used were heterogeneous. The authors concluded that there is insufficient evidence that electrical stimulation is more effective than sham stimulation. In a 2001 Cochrane review of pelvic floor muscle training for urinary incontinence in women, Hay-Smith et al. reviewed 43 trials [Hay-Smith et al. 2001]. The pelvic floor muscle training programs and comparison interventions varied markedly. Outcome measures differed between trials and the methods of data reporting varied, making the data difficult to combine. In the authors’ conclusions, pelvic floor muscle training appeared to be an effective treatment for adult women with stress or urge incontinence—better than no treatment or placebo treatments. Pelvic floor muscle training may be more effective than some types of electrical stimulation [Hay-Smith et al. 2001]. In a systematic review of electrical stimulation for fecal incontinence, Hosker et al. [2000] concluded that there is a suggestion that electrical stimulation may have a therapeutic effect, but stated that better-designed, generalizable trials are needed. All of the systematic reviews have concluded that further high-quality trials are necessary. The present author and other clinicians have noted that the lack of consistency in termi nology, definitions, and stimulation parameters in many studies hampers understanding of the procedures and makes the studies hard to compare. For clinicians to understand and compare parameters for known equipment, stimulation studies need to include: the name and type of the unit used, the waveform, frequencies, phase duration, intensity, duty cycle, and voltage, as well as patients’ response to the treatment. Amaro et al. [2003] studied 30 stress-incontinent women with a mean age of 54 years who were treated with intravaginal electrical stimulation and pelvic floor physiotherapy. The protocol consisted of three sessions of stimulation per week for 14 weeks, and physiotherapy (pelvic floor strengthening exercises) was initiated in the fifth week. The results reported included a significant decrease in voids and urgency, a reduction in urinary leakage using the pad test, and objective improvement in pelvic floor muscle strength. Arruda et al. [2003] studied 29 women with detrusor instability clinically and urodynamically before and after 3 months of functional pelvic floor stimulation. Twenty-two patients considered that they were cured or had symptomatic improvement, and seven patients had no change in symptoms after therapy. The authors considered that electrical stimulation resulted in a gradual decrease in the number of urinary leakage episodes and in an increase in maximum cystometric capacity in the first desire to void and in urinary volume. Indrekvam and Hunskaar [2003] investigated the extent to which physicians requested home electrical stimulation and found that gynecologists prescribed electrical stimulation more often and earlier than general practitioners. Prescribers of electrical stimulation were more proactive in treating urinary incontinence than those who were not prescribers. Spruijt et al. [2003] studied vaginal electrical stimulation of the pelvic floor in a randomized feasibility study in urinary-incontinent postmenopausal women aged 65 or older. The patients underwent electrical stimulation every other day or a daily Kegel exercise program for 8 weeks. The outcome variables were urinary leakage during a standardized pad test, pelvic muscle strength (measured by perineometer), and detrusor instability measured by urodynamics. There was no significant improvement in these variables in the population treated with electrical stimulation in comparison with the population treated with Kegel exercises. The authors concluded that treating elderly women with electrical stimulation has a high physical and emotional cost, that the effectiveness of electrical stimulation in the studied group was low, that there was no great discrepancy between the Pad test and subjective amelioration, and that the effectiveness of the treatment did not compensate for the long treatment protocol.
Conclusions
The principles of electrophysiology were presented here initially to facilitate an understanding of the effects of stimulation. This was followed by a discussion of pelvic floor stimulators, the clinical application of stimulation, and broad descriptions of more recent electrical technologies. Three case reports were presented. Highlights of research from 1895 to 2001 were discussed, with the parameters used being summarized in a chronological table.
The field of electrical stimulation therapy is vast, with multiple applications and as yet untapped possibilities not only for treatment of the pelvic floor but also of other systems in the body. The clinical aspects of electrical stimulation most familiar to the author in clinical practice only represent a tiny portion of the possible treatments. One begins to gain knowledge about electrical stimulation with general exposure to the method. One continues to learn and contribute to the body of knowledge through clinical experience and by comparing outcomes. Known outcomes such as conversion of fasttwitch to slow-twitch muscle fiber, indirect effects of endogenous analgesics as with TENS for pain, and neuromuscular reeducation show the potential of this treatment method. There is ample opportunity for continued focused research on the use of electrical stimulation for pelvic floor applications to improve outcomes in patients with pelvic floor dysfunction.
Glossary
Adenosine triphosphatase (ATPase). An enzyme that catalyzes the release of the terminal phosphate group of adenosine 5′ -phosphate.
Beta-endorphins. See endorphins, below.
Dopamine. A neurotransmitter in the brain. It is a catecholamine (a class of molecules that serve as neurotransmitters and hormones). It is a precursor of epinephrine and norepinephrine. It is used in the treatment of Parkinson’s disease.
Dynorphin. An opiate-like chemical found in the brain that blocks transmission of pain signals along nerve fibers.
Endogenous. Originating or produced in the organism or one of its parts.
Endorphins. One of three major groups of endogenous opioid peptides (painkillers) derived from the pro-opiomelanocortin precursor. The known members of this group are alpha, beta, and gamma endorphin. The term “endorphin” is sometimes used to refer to all opioid peptides.
Enkephalins. Natural opiate pentapeptides isolated originally from pig brain.
Fibroblast. A spindle-shaped cell with cytoplasmic processes present in connective tissue, capable of forming collagen fibers.
Fibroclast. A cell in connective tissue that is responsible for resorption of collagen fibers.
Mitochondrion. A small intracellular organelle that is responsible for energy production and cellular respiration.
Neurotransmitter. A chemical released from a nerve cell that transmits an impulse from one nerve to another nerve, muscle, organ or other tissue. A messenger of neurologic information from one cell to another.
Osteoblast. A mesodermal cell that gives rise to bone.
Osteoclast. A macrophage that is responsible for the breakdown or resorption of bone.
Peptides. A class of compounds of low molecular weight that yield two or more amino acids on hydrolysis. Formed by loss of water from the NH2 and COOH groups of adjacent amino acids. They are known as di-, tri-, tetra- poly-, etc., peptides, depending on the number of amino acids in the molecule. Peptides form the constituent parts of proteins.
Polypeptide. A compound consisting of 12 or more amino acids. Amino acids make up polypeptides, which in turn make up proteins.
Serotonin. A hormone, also known as 5-hydroxytryptamine, found in the pineal gland, blood platelets, digestive tract, and brain. It acts as a chemical messenger that transmits nerve signals between nerve cells and that causes constriction of blood vessels. Changes in serotonin levels can alter mood. Antidepressants affect the action of serotonin.
Substance P. A vasoactive intestinal peptide found in the brain, spinal ganglia, and intestine of vertebrates. Induces vasodilation and salivation, and increases capillary permeability.
Succinate dehydrogenase (SDH). Succinate is a salt of succinic acid, and SDH s a flavoenzyme that catalyzes the removal of hydrogen from succinic acid and converts it into fumaric acid.
Vasoactive intestinal polypeptide (VIP). A peptide of 28 amino acids originally isolated from porcine intestine, but later found in the central nervous system, where it acts as a neuropeptide and is released by specific interneurons. It may affect the behavior of the cells of the immune system.
References
Alexander S, Rowan D. An electric pessary for stress incontinence. Lancet 1968; 1:728.
Alexander S, Rowan D, Millar W, Scott R. Treatment of urinary incontinence by electric pessary: a report of 18 patients. Br J Urol 1970; 42:184–90.
Alon G. Principles of electrical stimulation. In: Nelson RM, Currier DP Clinical electrotherapy. Norwalk, CT: Appleton and Lange, 1987: 30–83.
Alon G. Clinical electrotherapy. In: Nelson RM, Currier DP Clinical electrotherapy, 2nd ed. Norwalk, CT: Appleton and Lange, 1991: 35–103.
Alon G, De Domenico G. High voltage stimulation. Hixson, TN: Chattanooga Corporation, 1987.
Amaro JL, Oliveira Gameiro MO, Padovani CR. Treatment of urinary stress incontinence by intravaginal electrical stimulation and pelvic floor physiotherapy. Int Urogynecol J Pelvic Floor Dysfunct 2003; 14:204–8.
Arruda RM, Castro RA, Sartori MG, Takano CC, Bara-cat EC, Rodrigues de Lima G, et al. Clinical and urodynamic evaluation of women with detrusor instability before and after functional pelvic floor electrostimulation. Clin Exp Obstet Gynecol 2003; 30:220–2.
Baeten CG, Uludag OO, Rongen MJ. Dynamic graciloplasty for fecal incontinence. Microsurgery 2001; 21:230–4.
Barr J. TENS. In: Nelson RM, Currier DP Clinical electrotherapy, 2nd ed. Norwalk, CT: Appleton and Lange, 1991: 11–33.
Bathurst LW. Incontinence of urine in women: treating by electrotherapy. Med Stand 1929; 15:17–19.
Bavendam TG. Impact of extracorporeal magnetic innervation (ExMI) on quality of life in the treatment of stress urinary incontinence. [Abstract presented at the American Urogynecological Association meeting, Atlanta, GA, 4–7 May 2000.]
Bent AE, Sand PK, Ostergard DR, Brubaker LT. Transvaginal electrical stimulation in the treatment of genuine stress incontinence and detrusor instability. Int Urogynecol J 1993; 4:9–13.
Benzl J. Anatomy and physiology. In: Benson JT Female pelvic floor disorders: investigation andmanagement. New York: Norton, 1992:219–31.
Berghmans LC, Hendriks HJ, B⊘ K, Hay-Smith EJ, de Bie RA, van Waalwijk van Doorn ES. Conservative treatment of stress urinary incontinence in women: a systematic review of randomized clinical trials. Br J Urol 1998; 82:181–91.
Berghmans LC, Hendriks HJ, De Bie RA, van Waalwijk van Doorn ES, B⊘ K, van Kerrebroeck PE. Conservative treatment of urge urinary incontinence in women: a systematic review of randomized clinical trials. BJU Int 2000; 85:254–63.
Bergman A. Nonsurgical treatment of stress urinary incontinence. In: Ostergard D Gynecologic urology and urodynamics: theory and practice. Baltimore: Williams & Wilkins, 1985: 424–6.
Bergmann S, Eriksen BC. Anal electrostimulation in urinary incontinence: technical description of a new device. Urol Int 1986; 41:411–7.
Blowman C, Pickles C, Emery S, Creates V, Towell L, Blackburn N, et al. Prospective double blind controlled trial of intensive physiotherapy with and without stimulation of the pelvic floor in treatment of genuine stress incontinence. Physiotherapy 1991; 77:661–4.
B⊘ K, Maanum M. Does vaginal electrical stimulation cause pelvic floor muscle contraction? A pilot study. Scand J Urol Nephrol Suppl 1996; 179:39–45.
Bouamrirene D, Micallef JP, Rouanet P, Bacou F. Electrical stimulation-induced changes in double-wrapped muscles for dynamic graciloplasty. Arch Surg 2000; 135:1161–7.
Brown MD, Cotter MA, Hudlicka O, Vrbova G. The effects of different patterns of muscle activity on capillary density, mechanical properties and structure of slow and fast rabbit muscles. Pflugers Arch 1976; 361:241–50.
Buller AJ, Eccles JC, Eccles RM. Differentiation of fast and slow muscles in the cat hind limb. J Physiol 1960; 150:399–416.
Caldwell KP. The electrical control of sphincter incompetence. Lancet 1963; 2:174–5.
Caldwell KP. The use of electrical stimulation in urinary retention and incontinence. Proc R Soc Med 1968; 61:703–7.
Caldwell KP, Cook PJ, Flack FC, James ED. Stress incontinence in females: report on 31 cases treated by electrical implant. J Obstet Gynaecol Br Commonw 1968; 75:777–80.
Carley PJ, Wainapel SF. Electrotherapy for acceleration of wound healing: low intensity direct current. Arch Phys Med Rehabil 1985; 66:443–6.
Clayton EB. Electrotherapy and actinotherapy. Baltimore: Williams & Wilkins, 1949: 443–6.
Cook T. Instrumentation. In: Nelson RM, Currier DP Clinical electrotherapy. Norwalk, CT: Appleton and Lange, 1987: 11–27.
Cook T, Barr J. Instrumentation. In: Nelson RM, Currier DP Clinical electrotherapy, 2nd ed. Norwalk, CT: Appleton and Lange, 1991: 11–27.
De Domenico G. Modern electrotherapeutics: interferential therapy—theory and application. Sydney: Theramed, 1984.
De Soldenhoff R, McDonnell H. New device for control of female urinary incontinence. Br Med J 1969; 4:230.
Doyle PT, Edwards LE, Harrison NW, Malvern J, Stanton SL. Treatment of urinary incontinence by external stimulating devices. Urol Int 1974; 29:450–7.
Eriksen BC. Electrostimulation of the pelvic floor in female urinary incontinence. Acta Obstet Gynecol Scand 1990; 69:359–60.
Eriksen BC. Electrical stimulation. In: Benson JT Female pelvic floor disorders: investigation and management. New York: Norton, 1992:219–31.
Eriksen BC, Eik-Nes SH. Long-term electrostimulation of the pelvic floor: primary therapy in female stress incontinence? Urol Int 1989; 44:90–5.
Eriksen BC, Mjolnerod OK. Changes in urodynamic measurements after successful anal electrostimulation in female urinary incontinence. Br J Urol 1987; 59:45–9.
Eriksen BC, Bergmann S, Eik-Nes SH. Maximal electrostimulation of the pelvic floor in female idiopathic detrusor instability and urge incontinence. Neurourol Urodyn 1989; 8:219–30
Erlandson BE, Fall M, Sundin T. Intravaginal electrical stimulation: clinical experiments of urethral closure. Scand J Urol Nephrol Suppl 1977a; 44: 31–9.
Erlandson BE, Fall M, Carlsson CA, Linder LE. Mechanisms for closure of the human urethra during intravaginal electrical stimulation. Scand J Urol Nephrol Suppl 1977b; 44:49–54.
Esa A, Kiwamoto H, Sugiyama T, Park YC, Kaneko S, Kurita T. Functional electrical stimulation in the management of incontinence: studies of urodynamics. Int Urol Nephrol 1991; 23:135–41.
Fall M. Does electrostimulation cure urinary incontinence? J Urol 1984; 131:664–7.
Fall M, Lindstrom S. Electrical stimulation: a physiologic approach to the treatment of urinary incontinence. Urol Clin North Am 1991; 18: 393–407.
Fall M, Erlandson BE, Nilson AE, Sundin T. Long-term intravaginal electrical stimulation in urge and stress incontinence. Scand J Urol Nephrol Suppl 1977; 44:55–63.
Fall M, Erlandson BE, Carlsson CA, Sundin T. Effects of electrical intravaginal stimulation on bladder volume: an experimental and clinical study. Urol Int 1978; 33:440–2.
Fall M, Ahlstrom K, Carlsson CA, Ek A, Erlandson BE, Frankenberg S, et al. Contelle: pelvic floor stimulator for female stress-urge incontinence. A multicenter study. Urology 1986; 27:282–7.
Furniss HD. Incontinence of urine due to relaxed vesicle sphincter. 1924; Obstetrics and Gynecology Vol. 8: 195–7.
Galloway NT, El-Galley RE, Sand PK, Appell RA, Russell HW, Carlin SJ. Update on extracorporeal magnetic innervation (EXMI) therapy for stress urinary incontinence. Urology 2000; 56(6 Suppl 1): 82–6.
Gault WR, Gatens PF Jr. Use of low intensity direct current in management of ischemic skin ulcers. Phys Ther 1976; 56:265–9.
Glen ES. Effective and safe control of incontinence by the intra-anal plug electrode. Br J Surg 1971a; 58: 249–52.
Glen ES. Intra-anal electrode: a stimulus to bowel and bladder control. J Pediatr Surg 1971b; 6: 138–42.
Godec CJ. Clinical application of Pavlov conditioning reflexes in treatment of urinary incontinence. Urology 1983; 22:397–400.
Godec CJ, Cass AS. Electrical stimulation for incontinence in myelomeningocele. J Urol 1978; 120: 729–31.
Godec C, Cass AS. Electrical stimulation for voiding dysfunction after spinal cord injury. J Urol 1979; 121:73–5.
Godec C, Cass AS, Ayala GF. Bladder inhibition with functional electrical stimulation. Urology 1975; 6:663–6.
Godec C, Cass AS, Ayala GF. Electrical stimulation for incontinence: technique, selection, and results. Urology 1976; 7:388–97.
Godec CJ, Fravel R, Cass AS. Optimal parameters of electrical stimulation in the treatment of urinary incontinence. Invest Urol 1981; 18:239–41.
Govier FE, Litwiller S, Nitti V, Kreder KJ Jr, Rosenblatt P. Percutaneous afferent neuromodulation for the refractory overactive bladder: results of a multicenter study. J Urol 2001; 165:1193–8.
Griffith J. Observations on the urinary bladder and urethra. J Anat Physiol 1989; 29:61–83.
Guido E. Effect of sympathetic therapy on chronic pain in peripheral neuropathy subjects [abstract]. Am J Pain Manage 2002; 12: 31.
Hakeem F. In: Wilder E The gynecological manual. St. Louis, MO: American Physical Therapy Association, Section on Women’s Health, 1997: 223–264.
Hakeem F. In: Wilder E Gynecological manual, 2nd ed. Alexandria, VA: American Physical Therapy Association Section on Women’s Health, 2002: 165–200.
Harrison NW, Paterson PJ. Urinary incontinence in women treated by an electronic pessary. Br J Urol 1970; 42:481–5.
Hay-Smith EJ, B⊘ K, Berghmans LC, Hendriks HJ, de Bie RA, van Waalwijk van Doorn ES. Pelvic floor muscle training for urinary incontinence in women. Cochrane Database Syst Rev 2001; 1:CD001407.
Hopkinson BR, Lightwood R. Electrical treatment of urinary incontinence using non-implanted apparatus. Br J Surg 1969; 56:631–2.
Hosker G, Norton C, Brazzelli M. Electrical stimulation for faecal incontinence in adults. Cochrane Database Syst Rev 2000;(2):CD001310.
Hudgins AM. Electrical muscle stimulation in gynecology. Pract Dig Treat 1955; 11:695–7.
Huffman JW, Osborne SL, Sokol JK. Electrical stimulation in the treatment of intractable stress incontinence. Arch Phys Med 1952; 33:674–6.
Indrekvam S, Hunskaar S. Home electrical stimulation for urinary incontinence: a study of the diffusion of a new technology. Urology 2003; 62(4 Suppl 1):24–30.
Janneck C. Electric stimulation of the bladder and the anal sphincter: a new way to treat the neurogenic bladder. Prog Pediatr Surg 1976; 9:119–39.
Jeyaseelan SM, Oldham JA. Electrical stimulation as a treatment for stress incontinence. Br J Nurs 2000; 9:1001–7.
Jeyaseelan SM, Haslam EJ, Winstanley J, Roe BH, Oldham JA. An evaluation of a new pattern of electrical stimulation as a treatment for urinary stress incontinence: a randomized, double-blind, controlled trial. Clin Rehabil 2000; 14:631–40.
Jonasson A, Larsson B, Pschera H, Nylund L. Short-term maximal electrical stimulation: a conservative treatment of urinary incontinence. Gynecol Obstet Invest 1990; 30:120–3.
Kegel A. Progressive resistance exercise in the functional restoration of the perineal muscles. Am J Obstet Gynecol 1948; 8:238–48.
Kegel A. Active exercise of the pubococcygeus muscle. Prog in Gynecol. 1950: In: Meigs JV, Sturgis SH Vol II. New York: Grune and Stratton, 1950:778–92.
Kelloway P. The part played by the electric fish in the early history of bioelectricity. Bull Hist Med 1947; 20:112–37.
Kidd GL, Oldham JA. Motor unit action potential (MUAP) sequence and electrotherapy. Clin Rehab 1988a; 2:23–33.
Kidd GL, Oldham JA. An electrotherapy based on the natural sequence of motor unit action potentials: a laboratory trial. Clin Rehab 1988b; 2:125–38.
Kiff ES, Swash M. Normal proximal and delayed distal conduction in the pudendal nerves of patients with idiopathic (neurogenic) faecal incontinence. J Neurol Neurosurg Psychiatry 1984; 47:820–3.
Kleinkort J. Too good to be true: autonomic modulation—fact or fiction? Orthop Phys Ther Pract 2001; 13:1–2.
Klingler HC, Pycha A, Schmidbauer J, Marberger M. Use of peripheral neuromodulation of the S3 region for treatment of detrusor overactivity: a urodynamic-based study. Urology 2000; 56:766–71.
Laycock J. Clinical evaluation of the pelvic floor. In: Schüssler B Pelvic floor re-education: principles and practice. New York: Springer, 1994:42–8.
Laycock J, Jerwood D. Does pre-modulated interferential therapy cure genuine stress incontinence? Physiotherapy 1993; 79:553–60.
Laycock J, Plevnik S, Senn E. Electrical stimulation. In: Schüssler B Pelvic floor re-education: principles and practice. New York: Springer, 1994:143–53.
Lindstrom S, Fall M, Carlsson CA, Erlandson BE. The neurophysiological basis of bladder inhibition in response to intravaginal electrical stimulation. J Urol 1983; 129:405–10.
Meyer S, Dhenin T, Schmidt N, De Grandi P. Subjective and objective effects of intravaginal electrical myostimulation and biofeedback in patients with genuine stress urinary incontinence. Br J Urol 1992; 69:584–8.
Moore T, Schofield PF. Treatment of stress incontinence by maximum perineal electrical stimulation. Br Med J 1967; 3:150–1.
Ohlsson BL, Erlandson BE. Miniaturised device for long-term intravaginal electrical stimulation for the treatment of urinary incontinence. Med Biol Eng Comput 1988; 26:509–15.
Plevnik S, Janez J. Maximal electrical stimulation for urinary incontinence: report of 98 cases. Urology 1979; 14:638–45.
Plevnik S, Janez J, Vrtacnik B, Trsinar B, Vodusek DB. Short-term electrical stimulation: home treatment for urinary incontinence. World J Urol 1986; 4:24–6.
Richardson DA, Miller KL, Siegel SW, Karram MM, Blackwood NB, Staskin DR. Pelvic floor electrical stimulation: a comparison of daily and every-other-day therapy for genuine stress incontinence. Urology 1996; 48:110–8.
Riddle PR, Hill DW, Wallace DM. Electronic techniques for the control of adult urinary incontinence. Br J Urol 1969; 41:205–10.
Rowley BA, McKenna JM, Wolcott LE. The use of low level electrical current for enhancement of tissue healing. Biomed Sci Instrum 1974; 10:111–4.
Salmons S, Vrbova G. The influence of activity on some contractile characteristics of mammalian fast and slow muscles. J Physiol 1969; 201: 535–49.
Sand P. Factors influencing success with extracorporeal magnetic innervation (ExMI) treatment of mixed urinary incontinence. [Abstract presented at the International Bladder Symposium, Washington, DC, 4–7 November 1999.]
Sand PK, Richardson DA, Staskin DR, Swift SE, Appell RA, Whitmore KE, et al. Pelvic floor electrical stimulation in the treatment of genuine stress incontinence: a multicenter, placebo-controlled trial. Am J Obstet Gynecol 1995; 173:72–9.
Saxtorph MH. Strictura urethrae, fistula perinaei, retentio urinae [1878]. In: Chirurgiske Forelæsninger: Supplement til “Clinisk Chirurgi,” 1878–79, 1882–83, 1884–85 Copenhagen: Gyldenalske Boghandels Forlag, 1879–86.
Schmidt RA. Treatment of unstable bladder. Urology 1991; 376:28–32.
Schmidt RA, Doggweiler R. Neurostimulation and neuromodulation: a guide to selecting the right urologic patient. Eur Urol 1998; 34(Suppl 1):23–6.
Schmidt RA, Senn E, Tanagho EA. Functional evaluation of sacral nerve root integrity: report of a technique. Urology 1990; 35:388–92.
Scott OM, Vrbova G, Hyde SA, Dubowitz V. Effects of chronic low frequency electrical stimulation on normal human tibialis anterior muscle. J Neurol Neurosurg Psychiatry 1985; 48:774–81.
Snooks SJ, Barnes PR, Swash M. Damage to the innervation of the voluntary anal and periurethral sphincter musculature in incontinence: an electrophysiological study. J Neurol Neurosurg Psychiatry 1984; 47:1269–73.
Somerville WE. Incontinence of urine after childbirth. Br Med J 1923; 8:327.
Sotiropoulos A. Urinary incontinence: management with electronic stimulation of muscles of pelvic floor. Urology 1975; 6:312–8.
Sotiropoulos A, Yeaw S, Lattimer JK. Management of urinary incontinence with electronic stimulation: observations and results. J Urol 1976; 116: 747–50.
Spruijt J, Vierhout M, Verstraeten R, Janssens J, Burger C. Vaginal electrical stimulation of the pelvic floor: a randomized feasibility study in urinary incontinent elderly women. Acta Obstet Gynecol Scand 2003; 82:1043–8.
Suhel P. Adjustable nonimplantable electrical stimulators for correction of urinary incontinence. Urol Int 1976; 31:115–23.
Tanagho EA. Neural stimulation for bladder control. Semin Neurol 1988; 8:170–3.
Teague CT, Merrill DC. Electric pelvic floor stimulation: mechanism of action. Invest Urol 1977; 15:65–9.
Unsal A, Saglam R, Cimentepe E. Extracorporeal magnetic stimulation for the treatment of stress and urge incontinence in women: results of 1-year follow-up. Scand J Urol Nephrol 2003; 37:424–8.
Van Balken MR, Vandoninck V, Messelink BJ, Vergunst H, Heesakkers JP, Debruyne FM, et al. Percutaneous tibial nerve stimulation as neuromodulative treatment of chronic pelvic pain. Eur Urol 2003; 43:158–63.
Vodusek DB, Plevnik S, Vrtacnik P, Janez J. Detrusor inhibition on selective pudendal nerve stimulation in the perineum. Neurourol Urodyn 1988; 6:389–93.
Wolcott LE, Wheeler PC, Hardwicke HM, Rowley BA. Accelerated healing of skin ulcer by electrotherapy: preliminary clinical results. South Med J 1969; 62:795–801.
Yamanishi T, Sakakibara R, Uchiyama T, Suda S, Hattori T, Ito H, et al. Comparative study of the effects of magnetic versus electrical stimulation on inhibition of detrusor overactivity. Urology 2000; 56:777–81.
Zollner-Nielsen M, Samuelsson SM. Maximal electrical stimulation of patients with frequency, urgency and urge incontinence: report of 38 cases. Acta Obstet Gynecol Scand 1992; 71:629–31.
Resources
Richard V. Exhaustive electrical stimulation bibliography. Consultation on electrical stimulation for postprostatectomy incontinence. Victor Richard Bioelectronics, 2314 Sherwood Lane, Havre de Grace, MD 21078–2018, USA. E-mail: bioelect @juno.com.
Biofeedback and Electromyography
Jane D. Frahm
Background
“Biofeedback” and “surface electromyography” (sEMG) are terms often used interchangeably. Their use complements the evaluation and treatment of the pelvic floor as part of a conservative management program. sEMG is a valuable form of biofeedback that physical therapists and other health-care practitioners use in the treatment of muscular dysfunction. Most areas of the body, including the pelvic floor, are accessible to convenient placement of surface electrodes. “Biofeedback” is the descriptor used predominantly in much of the published literature. In April 2000, a Medicare Coverage Advisory Committee (MCAC) was convened in Baltimore to examine biofeedback treatment of non-neurogenic incontinence in adults. During this process, a multidisciplinary group of health-care providers, including physicians, physical therapists, nurses, and psychologists were invited to present scientific evidence in support of why this treatment should be reimbursed by Medicare. On October 6, 2000, despite indications that the Centers for Medicare and Medicaid Services (CMS, formerly the Health Care Financing Administration, HCFA) had not been favorably disposed toward the use of these modalities, the organization issued a national coverage decision in favor of the use of biofeedback and pelvic floor electrical stimulation in the treatment of urinary incontinence. This was the first major health-care coverage decision made using the new CMS process. To the credit of the diverse health-care community, their organized and cohesive responses influenced CMS to make a positive coverage decision [Thompson 2002]. When billing for sEMG/biofeedback using common procedural terminology (CPT) codes, practitioners who are not physical therapists generally use the biofeedback code 90902. Most physical therapists regard sEMG/ biofeedback as “another tool in the treatment toolbox” and bill it as “neuromuscular reeducation,” using the code 97112 [Cram and Kasman 1998]. The way in which it is billed depends on current reimbursement practices, which vary and change frequently from state to state in the USA.
Definitions
Biofeedback
Biofeedback is the use of instrumentation to mirror psychophysiological processes of which the individual is not normally aware and which may be brought under voluntary control. Stated with respect to pelvic floor applications, “Biofeedback … is a therapy that uses an electronic or mechanical device to relay visual and/or auditory evidence of pelvic floor muscle tone, in order to improve awareness of pelvic floor musculature and to assist patients in the performance of pelvic floor muscle exercises” [Lefevre 2000]. This involves giving a person immediate information about his or her own biological conditions such as muscle tension, skin surface temperature, brain wave activity (with electroencephalography [EEG]), galvanic skin response (GSR), blood pressure (BP), and heart rate (with electrocardiography [EKG]). The feedback enables one to become an active participant in the process of health maintenance [Fuller 1977]. Examples are given in Table 2.11.
Electromyography
Electromyography is defined as the process of recording and displaying graphic tracings of the voltage levels in muscle tissue, used in the diagnosis (and treatment) of muscle and nerve disorders. Tracings can be made audible by connecting the voltage to a speaker.
Physiological function | Type of biofeedback and/or equipment |
---|---|
Heart rate |
|
Blood pressure |
|
Perspiration, skin temperature |
|
Brain waves | – Electroencephalography (EEG) |
Muscle activity | – Strength/force transducers, including manometric devices – sEMG with external or internal electrodes—clinical units or “home” trainers – Mirrors – External or internal muscle palpation (on trunk, extremities, within vaginal or anal canal – Vaginal weights—cone sets or single weights – Vaginal exercise devices with indicators to document the desired activity: PFX Peritron, Pelvic Floor Educator, etc. [Frahm 2004] |
Needle Electromyography
Needle electromyography (EMG) is a diagnostic procedure which introduces fine wire or needle electrodes into a single muscle unit to determine the degree of innervation, denervation, and function at that level. The magnitude of energy recorded is in thousandths of volts or millivolts (mV) [Cram and Kasman 1998].
Surface Electromyography
Surface electromyography (sEMG) is the recording of the algebraic sum of muscle action potentials from the skin surface [Basmajian and De Luca 1985, Cram and Kasman 1998]. It is used to monitor skeletal muscle tension—“a direct representation of the outflow of motor neurons in the spinal cord to the muscle as a result of voluntary or reflex action” [Turker 1993]—or “to assess the magnitude and timing of overall muscle contractions” [Wallace and Kasman 2002]. The sources of the sEMG signal come from populations of motor units, which produce motor unit action potentials (MUAPs) during any given contraction. It is important to know that in any motor recruitment pattern, motor units are activated in an asynchronous pattern, which facilitates the smoothness of the movement. The magnitude of energy recorded with sEMG is in millionths of volts, or microvolts (mV). This reduced amplitude with sEMG (compared to needle EMG, Table 2.12) is due to the impedance of the body tissue, which absorbs some of the electrical potential from the muscle as it makes its way to the skin’s surface.
Needle electromyography | sEMG |
---|---|
Invasive, can be painful | Noninvasive, painless |
Information from single motor unit | Information from multiple motor units |
Examines nerve conduction velocity | Examines functional contraction |
Used mainly for diagnosis | Used mainly for evaluation and treatment |
Advantages of sEMG [Cram and Kasman 1998].
- Surface recordings are noninvasive and provide a safe and easy method that allows objective quantification of the energy in a muscle. It is not necessary to penetrate the skin to record from single motor units in order to obtain meaningful and useful information about muscles.
- Synergies in energy and movement patterns that one cannot see with the naked eye are visible on the screen—for example, the synergy between strong pelvic floor contractions and abdominal muscle engagement (see Fig. 2.47). A study by Mallett and Frahm [1994] attempted to identify the presence of an abdominal/pelvic floor muscle co-contraction or synergy. They found that in the patients studied, abdominal muscle co-contractions were present during strong pelvic floor muscle contractions and that these contractions were not detrimental to the quality of the pelvic floor muscle contraction or to bladder function. The abdominal contractions observed were compressive, abdominal engagement of the oblique and transversus muscles and not passive bulging of the abdominal wall, as is noted with Valsalva maneuvers. The study has been presented orally at three conferences, but has not yet been published.
- The therapist can observe the muscle at rest and as it continuously changes during the course of a static or dynamic movement.
- By adding sEMG data to the manual examination, the therapist can validate his or her findings during the manual examination if the sEMG data are congruent with the palpation. The information obtained by sEMG can be “fed back” to the patient, providing a basis for neuromuscular reeducation and self-regulation. Examples of the types of motor activity that can be instantaneously displayed and viewed by both therapist and patient are shown in Table 2.13.
Limitations of sEMG [Cram and Kasman 1998].
- Only a few muscle sites can be monitored. Cram and Kasman [1998] consider that using one or two channels is limiting, and they recommend at least four channels for optimal monitoring.
- Isolation of muscle activity. Since one cannot truly isolate the activity of a specific muscle to the exclusion of others with sEMG, muscle activity has to be “labeled” according to the corresponding surface anatomy—e. g., perineal muscle activity as opposed to pubococcygeus muscle per se.
- sEMG is not a measurement of muscle strength, force, pain, tightness, length, the amount of effort being exerted, or spasm. sEMG monitors the events that lead to the generation of muscle tension—a measure of electrical activity given off by the muscle as it contracts and does work and a direct representation of the outflow of motor neurons in the spinal cord to the muscle as a result of voluntary or reflex action [Turker 1993].
- Microvolt signal comparisons from person to person (inter-session consistency) and across sites (microvolt quantification standards) within the same individual are potentially unreliable and not practical unless the data are normalized.
- Although a linear or near-linear relationship between sEMG activity and force can be expected for muscles of a relatively uniform fiber type or composition under isometric, nonfatiguing conditions, complex effects on the EMG–force relationship may occur due to situations in the clinic—such as gender differences and body fat percentages.
- Because of these inherent limitations of sEMG, it is important to reproduce the same conditions with positioning and electrode placements as much as possible within the same patient during progressive treatments, whether the patient is sitting, lying, or standing.
Motor recruitment | MDo muscles fire early or late in the motor recruitment pattern? |
Motor decruitment | Does the muscle “turn off” after the given movement, or is there irritability of the resting baseline following movement? |
Motor specificity | Does the particular movement activate the muscle groups intended, or is there substitution? |
Endurance | Is the patient able to demonstrate consistent holding of contractions (endurance), or does the contraction peak and then drop precipitously? |
Significant Components and Essential Features of sEMG Instrumentation
A general description of the components of sEMG instrumentation, although brief, may help therapists appreciate the technology and help them become knowledgeable users and confident and accomplished practitioners (Figs. 2.39–2.41).
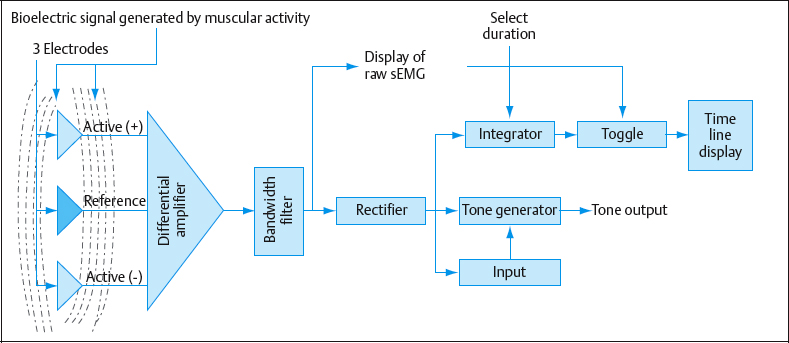
Fig. 2.39 Diagram of the surface electromyography (sEMG) instrument with several options. (Adapted from Cram and Kasman [1998].)
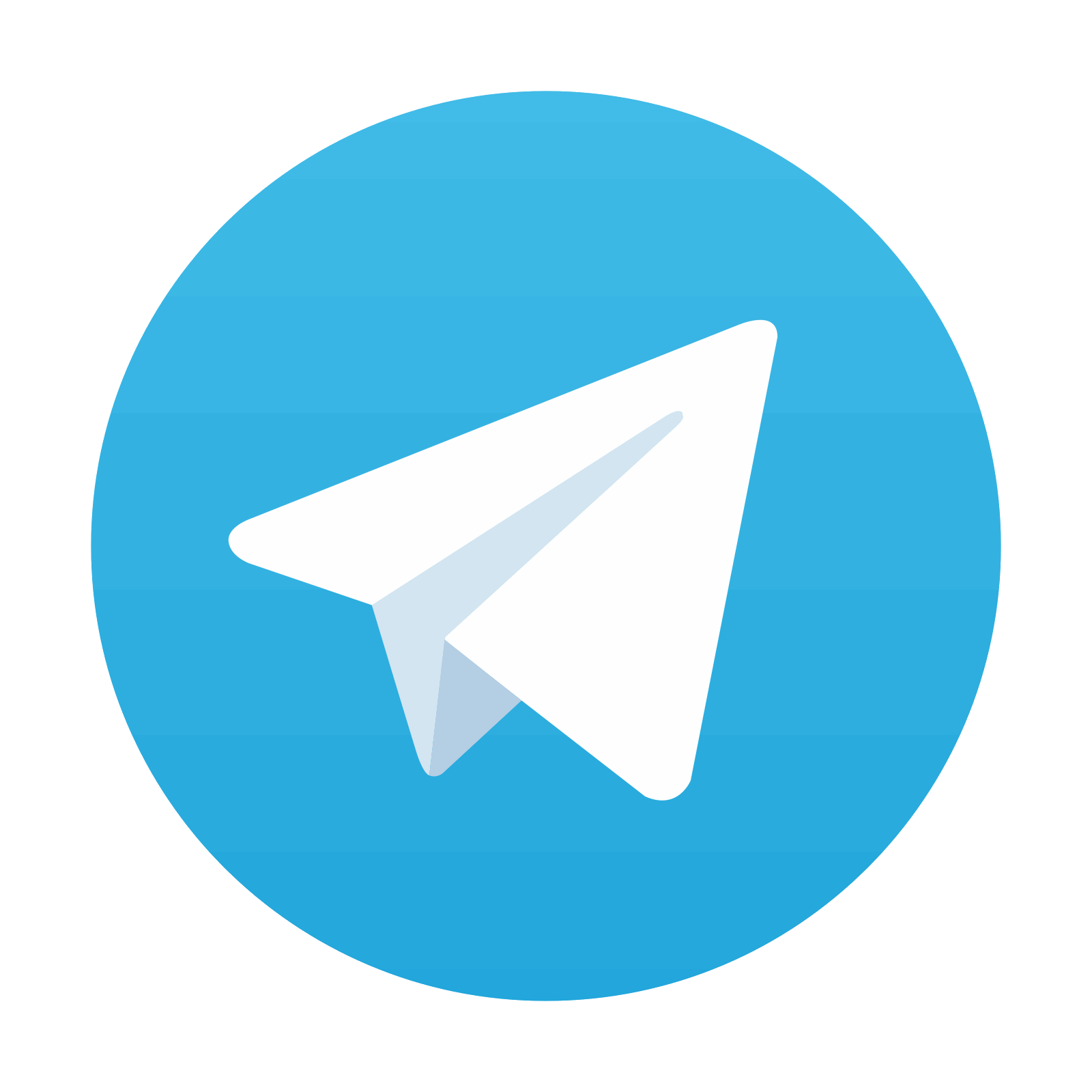
Stay updated, free articles. Join our Telegram channel

Full access? Get Clinical Tree
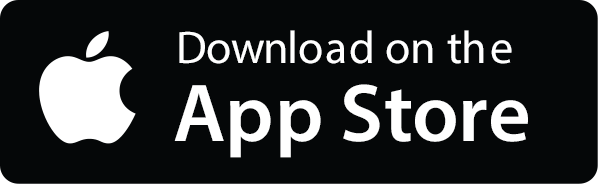
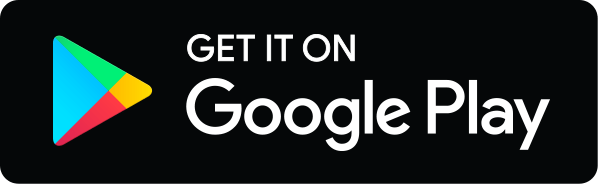