1.5 Low Back Pain and the Pelvic Floor
Introduction
All of the muscles of the trunk contribute, at least to some extent, to control of the spine and pelvis. This necessarily includes the muscles of the pelvic floor, due to the contribution of these muscles to the generation and modulation of intraabdominal pressure and due to direct effects via attachments to the pelvis, sacrum, and coccyx. Consequently, dysfunction of the pelvic floor is likely to affect the efficiency of lumbopelvic control, and dysfunction of motor control of the spine and pelvis in lumbopelvic pain is likely to have consequences for the continence mechanism. The objective of this section is to consider the contribution of the pelvic floor muscles to control of the lumbar spine and pelvis, the coordination between the multiple functions of the trunk muscles, the association between pelvic floor dysfunction and lumbopelvic pain, and the mechanical consequences of dysfunction of this system.
Contribution of the Pelvic Floor Muscles to Lumbopelvic Control
Requirements for Lumbopelvic Control
Optimal control of the lumbar spine and pelvis involves maintenance of a balance between movement and stability. The lumbar spine is inherently unstable. If all of the trunk muscles were to be removed, the spine would buckle and collapse under compressive loading of as little as 90 N [Lucas and Bresler 1960]. Thus, coordinated activity of the muscle system is required to maintain stability under functional loads [Panjabi 1992a]. There are several different components to stability. In a basic sense, stability can be regarded as the requirement to prevent buckling and collapse of the spine due to compressive forces (Euler stability) and the requirement to control translations between vertebrae (shear stability) [McGill 2002] (Fig. 1.46). Notably, the mechanisms for controlling each of these elements may be independent to some extent.
An important consideration is that, although normal function of the lumbar spine and pelvis requires adequate consideration of mechanisms for maintaining stability, this must be balanced with the requirement for movement. That is, stability must be dynamic. Movement is critical to minimize forces acting on the spine (shock absorption) [Hodges 2004], reduce energy expenditure (such as movement of the spine and pelvis to minimize movement of the center of mass during gait) [Perry 1992], and is important for other functions such as balance control [van Dieen and Mok, 2004] and breathing [Hodges et al. 2002]. Notably, when the nervous system aims to control stability, these strategies often involve movement rather than simply making the spine rigid [Hodges et al. 1999]. If stability were to be optimized – for example, by strong co-contraction of large trunk muscles – at the expense of movement, this would reduce the contribution of movement to lumbopelvic control, restrict the movement of the spine required for normal respiration, and restrict the contribution of movement of the spine and pelvis to maintain the center of mass over the base of support. Optimal control relies on a precise match between the demands for movement and stability, and the demands of the task.
For control of the pelvic girdle, it is important to consider the control of the orientation of the pelvis, and stability of the sacroiliac joints and pubic symphysis. In upright postures, the mass of the upper body must be transferred across the sacroiliac joints to the lower limbs [Snijders and Vleeming 1993a, 1993b]. This is associated with high downward shear forces at the sacroiliac joints. Two mechanisms are available to control this shear force and maintain the integrity of the sacroiliac joints [Snijders and Vleeming 1995]. The first is related to the shape of the joints; the sacrum is wedged between the ilia, the joint surfaces are undulated rather than smooth, and the cartilage of the joint surface has a high friction coefficient [Snijders and Vleeming 1995]. Each of these factors resists downward shear and is called “form closure.” The other mechanism is known as “force closure” and refers the contribution of muscle forces to compression of the joints, which assist control of shear forces. The stability of the sacroiliac joints is also dependent on the position of the sacrum relative to the ilia [Snijders and Vleeming 1995]. If the sacrum is rotated forward relative to the ilia (i. e., nutation of the sacrum, Fig. 1.47), the posterior sacroiliac ligaments are tightened, which places the joint in the close-packed position and increases the stability of the joint. The opposite movement (counternutation, Fig. 1.47) reduces the stability of the joint. Compression of the pubic symphysis is required to assist the passive elements to control the stability of this joint. The muscles of the pelvic floor may contribute to force closure and motion of the sacrum relative to the ilia.
Contribution of the Pelvic Floor Muscles to Control of the Lumbar Spine
Muscular Control of the Lumbar Spine
All muscles of the trunk contribute to control of the lumbar spine. However, different muscles and control strategies are required to maintain the stability of the spine against buckling forces and shear. Control of buckling forces is dependent on muscles that act like the guy wires of the mast of a ship. Muscles that are ideal for this function include the lumbar erector spinae, obliquus externus abdominis, and quadratus lumborum [McGill 2002]. These muscles co-contract and increase the stability of the spine against compressive loads. This type of strategy is particularly important in tasks with higher demand such as lifting a mass from the floor [Cholewicki and McGill 1996] or when the loading of the trunk is unpredictable [Van der Burg et al. 2003].
Control of intervertebral motion is dependent on mechanisms such as compressive forces generated by contraction of the deep back muscles, such as lumbar multifidus [Bogduk et al. 1992], tension of the thoracolumbar fascia [Tesh et al. 1987], and increased intraabdominal pressure [Hodges et al., 2005]. Intra-abdominal pressure is controlled by the muscles that surround the abdominal cavity (Fig. 1.48). This includes the abdominal muscles, the diaphragm, and the pelvic floor muscles [Hemborg et al. 1985]. Contraction of the pelvic floor muscles supports the abdominal and pelvic contents, as well as providing an additional striated muscle contribution to the maintenance of urinary and fecal continence [De-Lancey 1994]. If the other muscles of the abdominal cavity contract, but without tension in the pelvic floor muscles, there would be minimal increase in intraabdominal pressure and descent of the pelvic floor (Fig. 1.49). Adequate control of the pelvic floor muscles is therefore essential in order to modulate intraabdominal pressure. Furthermore, tension can only be generated in the fascial structures that attach to the spine and pelvis if shortening of the muscles is resisted (Fig. 1.49). If the abdominal muscles (particularly the transversus abdominis) that attach to the thoracolumbar fascia contract, they narrow the waist and displace the abdominal contents superiorly and inferiorly. If the diaphragm and pelvicfloor muscles contract at the same time as the abdominal muscles, the displacement of the abdominal contents is resisted and the tension in the muscles and fascia is increased as the muscles contract around the abdominal cavity (Fig. 1.49). Thus, pelvic floor muscle contraction is essential both to tense the thoracolumbar fascia and to increase intraabdominal pressure.
When considering the range of strategies available to control stability, it is important to recognize that intervertebral stability can be achieved if the spine is compressed by co-contraction of the larger superficial muscles, the guy wires, or by contraction of the deeper muscles of the abdominal canister. A key advantage of using the deep muscles, fascial tension, and intraabdominal pressure is that they control intervertebral motion without restricting the overall movement of the spine. That is, these muscles can control the spine in a dynamic sense by providing underlying control of intervertebral motion, while the larger, more superficial muscles can move the spine and oppose applied forces [Hodges 2004].
Intra-Abdominal Pressure and the Pelvic Floor
It has been argued for many years that intraabdominal pressure contributes to control of the spine. Originally, intraabdominal pressure was thought to act as a balloon in front of the spine that pushed down on the pelvic floor and up on the diaphragm to cause the spine to extend and thus prevent it from being flexed during lifting [Bartelink 1957, Morris and Lucas 1961]. However, this was questioned, as the pressures that would need to be generated to produce this effect are greater than those recorded during functional tasks [Davis and Troup 1964]. Recent in-vivo human experiments that involved measuring the effect of an artificial increase in intraabdominal pressure from electrical stimulation of the diaphragm indicate that the extension moment produced by intraabdominal pressure is small [Hodges et al. 2001a]. Alternatively, it has been argued that intraabdominal pressure controls shear forces. This has been confirmed in vivo. Resistance of the spine to a posteroanterior shear force is increased when intraabdominal pressure is increased by stimulation of the diaphragm in humans [Hodges and Shirley, in press] and stimulation of the diaphragm and/or transversus abdominis in pigs [Hodges et al. 2004]. Thus, via a contribution to intraabdominal pressure, the pelvic floor muscles may contribute to intervertebral control of the lumbar spine (Fig. 1.49). An important consideration is that there are many muscles that can modulate intraabdominal pressure, and the effect of the pressure on the spine may differ between strategies. For instance, if pressure is generated by bracing of the superficial oblique abdominal muscles, the flexor moment from contraction of these muscle must be matched by contraction of the extensor muscles. This strategy could make the spine rigid, which may compromise dynamic control, and although this strategy may be appropriate when high loads are imposed on the spine (e. g., during lifting), this may not be ideal for low-load functional situations. In addition, the pressure generated from this contraction may overcome that of the pelvic floor muscles and lead to descent of the pelvic floor. Alternatively, if pressure is generated by contraction of the pelvic floor muscles, transversus abdominis, and the diaphragm, these muscles do not generate torque and can control intervertebral motion without making the spine rigid.
Thoracolumbar Fasical Tension and the Pelvic Floor
It has been also argued that tension of the thoracolumbar fascia produces an extension moment and controls intervertebral shear force [Farfan 1975, Tesh et al. 1987]. Although the contribution to extension has been shown to be small [Bogduk and Macintosh 1984, McGill and Norman 1988], recent porcine studies provide evidence that fascial tension contributes to the control of intervertebral motion [Hodges et al. 2003a], and in-vitro human cadaver studies indicate that tension of the fascia controls intervertebral translation/shear [Barker and Guggenheimer 2004]. Thus, via an indirect contribution to fascial tensioning by resisting displacement of the abdominal contents (Fig. 1.49), the pelvic floor muscles provide an additional effect on the control of intervertebral motion.
Motor Control of the Pelvic Floor Muscles for Control of the Lumbar Spine
Coordinated activity of the muscles that surround the abdominal cavity (sometimes described as an “abdominal canister,” Fig. 1.48) is required to meet the demands of control of the lumbar spine. Coordinated activity of the pelvic floor muscles, diaphragm, and transversus abdominis has been investigated in a number of studies, and a variety of control strategies have been identified. When the spine is challenged in a predictable manner, such as when a limb is moved rapidly, the pelvic floor muscles [Hodges and Sapsford 2002] (Fig. 1.50), transversus abdominis [Hodges and Richardson 1997], and diaphragm [Hodges et al. 1997] contract in advance of the initiation of the movement. Notably, this response occurs irrespective of the direction of force at the spine, and is accompanied by contraction of the deep fibers of the lumbar multifidus [Moseley et al. 2002]. Another example of a predictable challenge to spinal control is lifting a mass from the floor. Pelvic floor muscle activity has been recorded during this task [Hemborg et al. 1985]. It is argued that the activity of these muscles is responsible for the control of intervertebral motion (Fig. 1.46). Contraction of the more superficial muscle also occurs in this situation. However, the activity of these muscles is responsible for controlling the orientation of the spine and control of buckling forces (Fig. 1.46) and is dependent on the direction of forces acting on the spine [Aruin and Latash 1995, Hodges and Richardson 1997]. As expected, in conjunction with the activity of the muscles of the abdominal canister, intraabdominal pressure is increased in advance of predictable challenges to the spine [Hodges et al. 1997]. Thus, the response of the pelvic floor muscles in this context is similar to the activity of the pelvic floor muscles in advance of other tasks that involve an increase in intraabdominal pressure, such as a cough or sneeze [Thind et al. 1990]. An important issue to consider is that the majority of these studies have investigated the general activity of the pelvic floor muscles. Further studies are required to determine whether specific muscles in the pelvic floor are differentially active in these tasks.
When the spine is challenged unpredictably, activity of the trunk muscles is initiated with short latency after the disturbance, in response to afferent (sensory) input from the perturbation, such as muscle stretch or joint movement. Recent studies with recordings of pelvic floor muscle activity have confirmed the contribution of these muscles to this task [Sommerville and Hodges, unpublished data]. When stability of the spine is challenged in an ongoing manner, such as during repetitive arm movements or walking, activity of the diaphragm [Hodges and Gandevia 2000a] and transversus abdominis [Hodges and Gandevia 2000b] occurs tonically. Studies of repetitive arm movement confirm that activity of the pelvic floor muscles occurs tonically, with increased activity with each arm movement [Hodges and Sapsford 2002].
Coordination of the Muscles of the Abdominal Canister
Studies of the postural function of the pelvic floor muscles suggest a coordinated strategy in which all of the muscles of the abdominal canister are co-activated in order to control the intraabdominal pressure and fascia tension. Ideally, this would be simplified by neural strategies that coordinate their activity. Early animal studies provide support for this hypothesis. Stimulation of the pelvic afferents was associated with reflex co-activation of the diaphragm, pelvic floor and abdominal muscles [Yamamoto et al. 1961]. This provides an initial indication that coordinative strategies do exist, even at the reflex level. More recent studies have investigated similar phenomena in human subjects. These studies have evaluated whether co-activation of the transversus abdominis, diaphragm, and pelvic floor muscles is initiated by contraction of one muscle of the group. Initial studies showed that although activity of all of the abdominal muscles was initiated by maximal contraction of the pelvic floor muscles, the activity was more selective to the transversus abdominis when the pelvic floor muscle contraction was performed in a slow, controlled manner [Sapsford et al. 2001] (Fig. 1.51). The converse was also tested, with the subjects performing a transversus abdominis contraction, and pelvic floor muscle activity was initiated [Sapsford and Hodges 2001].
More recently, authors have argued that in some individuals, contraction of the transversus abdominis may lead to descent of the pelvic floor muscles [B⊘ et al. 2003]. However, it is not possible to infer activity of pelvic floor muscles from this measure. In addition, it is difficult to interpret these data as the strategy used by the subjects, as no attempt was made to confirm that the contraction of transversus abdominis was isolated from a bracing maneuver of the oblique abdominal muscles, pelvic floor motion was measured via a transabdominal technique (bracing of the abdominal wall would have displaced the ultrasound transducer, giving the appearance of pelvic floor descent), and women with mild incontinence were included in the study. In a final study, activity of the diaphragm was identified in conjunction with voluntary contraction of the transversus abdominis [Allison et al. 1998]. Although this could not be convincingly confirmed due to the use of surface electrodes to record from the diaphragm, the findings were confirmed in a more recent study using intramuscular electrodes to record the activity of the diaphragm [Hodges and Gandevia 1998].
In summary, the muscles of the pelvic floor provide a critical contribution to the control of the spine, particularly for control of intervertebral motion via intraabdominal pressure increases and tension of the thoracolumbar fascia. Notably, the activity of these muscles is coordinated as a component of the neural strategy to meet the challenges to control of the spine.
Contribution of the Pelvic Floor Muscles to Control of the Pelvis
Muscular Control of the Pelvic Girdle
Control of the joints of the pelvic girdle (sacroiliac joints and pubic symphysis) is dependent on muscle activity. Contraction of the transversus abdominis, which attaches to the iliac crests, generates a compressive force at the sacroiliac joint and reduces joint laxity [Snijders and Vleeming 1995] (Fig. 1.52). Due to the lever created by the ilia, the force of contraction of this muscle is amplified, creating an efficient mechanism for control [Snijders and Vleeming 1995]. The effect of this contraction on the sacroiliac joints has been confirmed in vivo in humans with measurement of sacroiliac joint laxity with ultrasound imaging [Richardson et al. 2002]. This mechanism can also be assisted by contraction of the obliquus internus abdominis due to the horizontal orientation of the lower fibers of this muscle. Furthermore, posterior muscles such as the gluteal muscles and latissimus dorsi (together called the posterior sling, Fig. 1.52) and some fibers of the lumbar multifidus, which also cross the joints, may assist the force closure mechanism [Snijders and Vleeming 1995] (Fig. 1.52).
Control of the pubic symphysis has been less well investigated. Contraction of the transversely oriented abdominal muscles, with attachments to the iliac crests and inguinal ligaments, will compress the joint, at least superiorly [Cowan and Schache, in press]. It has also been argued that some fibers of the abdominal muscles may cross the joint, leading to direct compression (Fig. 1.52). Whether the pelvic floor muscles contribute to the control of the pubic symphysis is unclear.
Finally, in addition to the control of the joints of the pelvis it is also necessary to control the orientation of the pelvis in space (Fig. 1.46). Orientation of the pelvis may be controlled by co-contraction of the larger superficial abdominal muscles or by carefully sequenced activity of these muscles [Saunders et al. 2004]. As for the lumbar spine, the balance between movement and stability must be matched to the demands of each task.
Pelvic Floor Muscles and Pelvic Girdle Control
The effect of pelvic floor muscles on the joints of the pelvis is complex. The iliococcygeus and ischiococcygeus muscles cross the sacroiliac joints and may generate a compressive force [Pool-Goudzwaard et al. 2004] (Fig. 1.52). Data from an in-vitro model suggest that simulated contraction of these muscles may increase sacroiliac stability in women, but not men [Pool-Goudzwaard et al. 2004]. However, contraction of the pelvic floor muscles – particularly the pubococcygeus and puborectalis – would also have the effect of counternutation of the sacrum (i. e., rotation of the sacrum backward relative to the ilia) (Fig. 1.52). This would place the sacroiliac joints in an open packed position, thus reducing the inherent stability of the joint. This motion may be counteracted by co-contraction with the lumbar multifidus, which acts to nutate the sacrum [Snijders and Vleeming 1993a] (Fig. 1.52). This requirement for coordinated activity of multiple muscles to ensure control highlights the importance of the integrated function of the muscles of the abdominal canister. For instance, pelvic floor muscle activity is required when intraabdominal pressure is increased, but as this is accompanied by counternutation of the sacrum, activity of the transversus abdominis is required to increase force closure of the sacroiliac joint, and activity of the lumbar multifidus is required to nutate the sacrum and assist force closure. Any factor that compromises the function of any component of the system (for example, altered function of the pelvic floor muscles with incontinence, altered function of multifidus with low back pain, or altered function of the transversus abdominis with respiratory disease or low back pain) is likely to lead to compromised control of lumbopelvic stability.
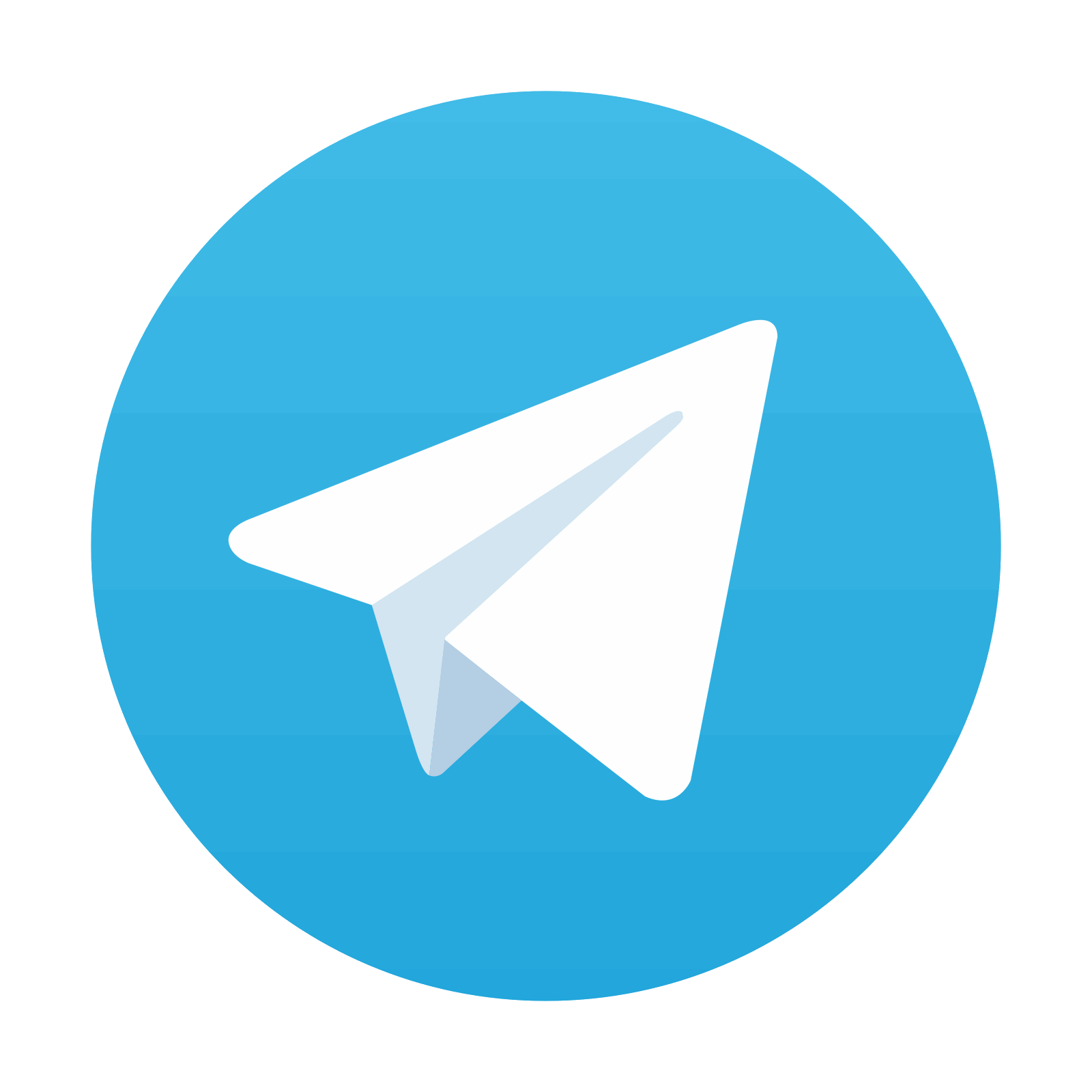
Stay updated, free articles. Join our Telegram channel

Full access? Get Clinical Tree
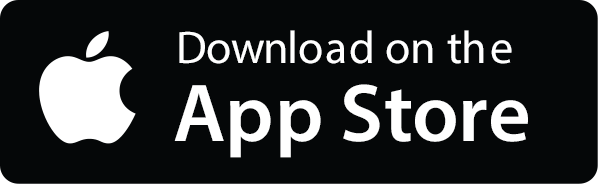
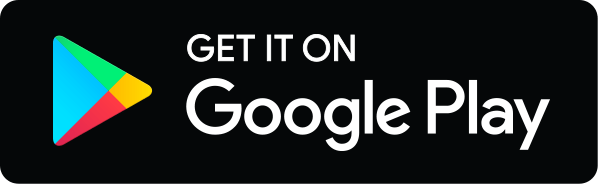