Chapter 20 Physical Agent Modalities
Modalities are physical agents that are used to produce a therapeutic response in tissue. They include heat, cold, water, sound, electricity, and electromagnetic waves (including infrared, visible, or ultraviolet light; shortwaves; and microwaves) (Figure 20-1). This chapter focuses on these physical agent modalities, except for most of the therapeutic uses of electrical stimulation (which are covered in Chapter 21). These modalities are generally considered adjunctive treatments rather than primary curative interventions. This chapter reviews the physiologic effects, common uses, techniques of application, and precautions for the therapeutic use of modalities. Although there is a growing body of literature to support the use of physical agent modalities, further research is needed to more specifically define the efficacy and indications for these agents.17,159–162
Modality Prescription
The elements of a prescription for heat or cold are listed in Box 20-1. The condition for which the modality is being used should be clearly indicated. The location to be treated influences modality selection, in that large areas can preclude the use of modalities such as ultrasound or ice massage. The surface to be treated can also influence selection. If using ultrasound over an irregular surface, degassed water might be preferred over a gel coupling agent. If using superficial heat over an irregular surface, hot packs or heating pads can result in focal heating over prominences, so radiant heat might be preferred. Intensity should be indicated where appropriate (e.g., ultrasound power output, hydrotherapy, fluidotherapy, and paraffin bath temperature). Most modalities allow only qualitative dosimetry as currently used in physical medicine, and therefore rely on patient perception of thermal intensity for safety. The duration for most modalities is 20 to 30 minutes, except for ultrasound, which is typically 5 to 10 minutes per site. Frequency is based on the severity of the condition being treated and on clinical judgment. Although information about duration and intensity of treatment is noted in this chapter, it is intended only as a guideline, and should be modified by clinical experience and the clinical condition.
Modality selection is influenced by multiple factors (Box 20-2). In selecting a modality, one should recognize that there are few well-designed clinical trials demonstrating the efficacy of specific modalities in specific conditions. There are, however, numerous studies that review the physiologic effects of modalities. Having a firm understanding of the physiologic effects of a particular modality allows one to make an educated selection. One must understand the heating or cooling capabilities of the various modalities to ensure the selection of the proper modality for the target tissue. Body habitus influences modality selection in that subcutaneous adipose affects the depth of penetration of many modalities. Comorbid conditions should also be considered. For example, both cold and heat can have adverse effects in the patient with significant arterial insufficiency. Cold can have harmful effects via the production of arterial vasoconstriction, and heat can cause complications via the production of increased metabolic activity, which can exceed the potential increase in blood supply and produce ischemia. Age is also a factor in modality selection. In the pediatric population, ultrasound should generally be avoided near open epiphyses.186 In the elderly population, there can be comorbidities that affect modality choice. Gender can also play a role in modality use, because fetal malformations have been reported after ultrasound near a gravid uterus.133
Heat
Forms of heat can be broadly classified by depth of penetration and form of heat transfer (Table 20-1). Depth of penetration is arbitrarily divided into superficial and deep. Superficial heat includes hot packs, heating pads, paraffin baths, fluidotherapy, whirlpool baths, and radiant heat. Deep heating agents (or diathermies) include ultrasound, shortwave, and microwave. Mechanisms of heat transfer include conduction, convection, radiation, evaporation, and conversion (Figure 20-2). Conduction is the transfer of thermal energy between two bodies in direct contact. Convection uses movement of a medium (e.g., water, air, or blood) to transport thermal energy, although the actual transfer of thermal energy is ultimately by conduction. Radiation refers to the thermal radiation emitted from any body whose surface temperature is above absolute zero (–273.15° C or –459.67° F). Evaporation involves the transformation of a liquid into a gas, a process that requires thermal energy. Evaporation is actually a process of heat dissipation, and plays a role in cooling modalities such as vapocoolant sprays. For each gram of water that evaporates from the body surface, approximately 0.6 cal (calorie) of heat is lost.81 Conversion refers to the transformation of energy (e.g., sound or electromagnetic) to heat. Likewise, the human body converts protein, carbohydrates, and fats to thermal energy via numerous metabolic processes. The next section reviews the physiologic effects (Box 20-3) of the therapeutic use of heat, followed by discussions of the general uses of heat (Box 20-4), some general precautions (Box 20-5), and descriptions of the agents currently used in physical medicine.
Table 20-1 Classification of Various Types of Heating
Type of Heating | Depth | Main Mechanism of Energy Transfer |
---|---|---|
Hot packs or heating pads | Superficial | Conduction |
Paraffin baths | Superficial | Conduction |
Fluidotherapy | Superficial | Convection |
Whirlpool baths | Superficial | Convection |
Radiant heat | Superficial | Radiation |
Ultrasound | Deep | Conversion |
Shortwave diathermy | Deep | Conversion |
Microwave | Deep | Conversion |
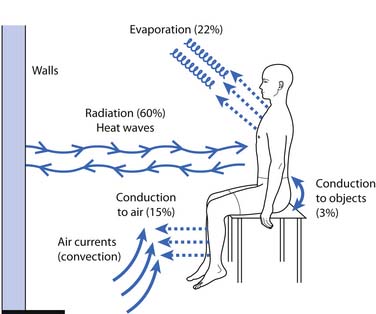
Figure 20-2 Mechanisms of heat transfer.
(Redrawn from Guyton AC: Body temperature, temperature regulation, and fever. In Guyton AC, edition: Textbook of medical physiology, 8th ed, Philadelphia, 1991, Saunders.)
Physiologic Effects of Heat
Hemodynamic
Localized heating produces a variety of hemodynamic effects. A twofold to threefold increase in forearm blood flow has been demonstrated after hydrotherapy at 44° to 45° C (111.2° to 113° F) or shortwave diathermy (SWD).1 This vasodilatation results in increased ingress of nutrients, leukocytes, and antibodies, and increased egress of metabolic byproducts and tissue debris, and can facilitate resolution of inflammatory conditions.80,173 Vasodilatation with heating can unfortunately also contribute to increased bleeding and increased edema formation, and can exacerbate acute inflammatory conditions.173 A number of experimental animal models of acute and chronic inflammation support the general clinical impression that acute inflammatory conditions tend to react unfavorably to heat, whereas chronic inflammatory conditions tend to benefit from heat.173
Neuromuscular
Animal experiments of localized heating have demonstrated increased firing rates in all group 1a fibers (muscle spindle) and many group 1b (Golgi tendon organ) fibers, and decreased firing rates in most group 2 fibers (muscle spindle).136 It should be noted that all experiments on muscle spindle and Golgi tendon organ firing rates are significantly influenced by the length and tension at which they are performed. This factor probably accounts for many of the differences among investigators.63,136 In contrast, the effects of temperature change on nerve conduction velocity are much more consistent. Heating produces modest increases in conduction velocity, whereas cooling is capable of producing dramatic decreases in conduction velocity. Abramson et al.1 noted increases in conduction velocity of up to 7.5 m/s after hydrotherapy at 44° to 45° C (111.2° to 113° F) or SWD, whereas cooling produced conduction velocity decreases of up to 35.8 m/s. The effects of temperature in clinical neurophysiology are succinctly summarized elsewhere.49
Joint and Connective Tissue
In vitro experiments demonstrate the importance of combined heating and stretching to maximize tendon extensibility. Lehmann et al.119 measured tendon extensibility under a variety of temperature and loading conditions. Tendon extensibility was greater at 45° C (113° F) than at 25° C (77° F). Furthermore, simultaneous use of heating and stretching produced significantly increased tendon extensibility when compared with the isolated use of either agent. Sustaining stretching during the cooldown period also facilitated tendon elongation. Other investigators have demonstrated metacarpophalangeal joint stiffness to decrease by as much as 20% at 45° C (113° F) compared with at 33° C (91.4° F).205 Temperature also affects enzymatic activity. In vitro experiments have shown a fourfold increase in collagenase activity with a temperature increase from 33° to 36° C (91.4° to 96.8° F).82
Miscellaneous Effects of Heat
It is generally accepted that heat produces an analgesic effect. A variety of mechanisms for the analgesic effect of heat have been postulated.65,112 These include a cutaneous counterirritant effect, vasodilatation resulting in decreased ischemic pain, vasodilatation resulting in washout of pain mediators, an endorphin-mediated response, alteration of nerve conduction, and alteration of cell membrane permeability. Elevation of the pain threshold has been demonstrated after therapeutic application of ultrasound, microwave, and infrared radiation.113 Many patients also find heat to have a general relaxation effect, although the specific mechanisms are not well defined.
General Uses of Heat in Physical Medicine
The general uses of heat are summarized in Box 20-4. These uses are based on the physiologic effects previously described. Heat is used in a variety of musculoskeletal conditions because of its potential to produce analgesia and muscle relaxation, and to facilitate resolution of inflammation. Increased soft tissue extensibility and decreased joint stiffness make heat useful in contractures and a variety of arthritides.
General Precautions for the Use of Heat
The general precautions for the use of heat are listed in Box 20-5. As noted earlier, heat can exacerbate acute inflammation and should generally be avoided in the acute management stage.173 In the patient with impaired circulation, increased metabolic activity with heating can exceed the capacity of arterial supply, so heat should be used with caution in this patient population. Vasodilatation from heat can result in increased bleeding in persons with bleeding diatheses. Vasodilatation can also produce increased edema. Because scars can be relatively avascular and have a reduced ability to dissipate heat, they can be selectively heated. Heat should generally be avoided in areas of impaired sensation because of the obvious potential for thermal injury as a result of the lack of precise dosimetry in modality use. This same reason justifies caution in using heat in patients with impaired cognition or communication, which precludes reporting of pain. Lehmann112 cautioned against the use of heat over malignancies because of the potential for increased rate of tumor growth or hyperemia increasing hematogenous spread. However, this should be distinguished from the use of specific local hyperthermia in the adjunctive treatment of malignancies.155 It also does not preclude the use of heat for adjunctive analgesia in the terminally ill cancer patient.
Superficial Heat
Superficial heating agents achieve their maximum tissue temperatures in skin and subcutaneous fat. Deeper tissue heating is limited by vasodilatation (which dissipates heat) and the insulating properties of fat. Superficial heating agents can heat via conduction (hot packs, heating pads, and paraffin baths), radiation (heat lamps), or convection (fluidotherapy and whirlpool baths). Superficial heat is used in osteoarthritis, rheumatoid arthritis, neck pain, low back pain, muscle pain syndromes, and a variety of musculoskeletal conditions.112
Hot Packs
Commercially available hot packs, such as Hydrocollator packs, typically contain silicon dioxide encased in a canvas pack. They come in a variety of sizes and styles for use over different areas. Hot packs are immersed in tanks at 74.5° C (166° F) and applied over several layers of insulating towels.122 After several minutes the skin should be inspected briefly to ensure that the heating is not excessive. Total treatment time is usually 30 minutes. Lehmann et al.122 showed that the 30-minute application of a hydrocollator pack to the posterior thigh produced approximately 3.3° and 1.3° C tissue temperature elevations at depths of 1 and 2 cm, respectively. Other investigators observed a 1.1° C temperature rise 4 cm deep in the brachioradialis muscle after a 30-minute application of a hydrocollator pack.2 A 1.2° C increase in intraarticular temperature (knee) has also been demonstrated after hot pack application.199 General heat precautions should be observed. The patient should not lie on the pack. This can squeeze water from the pack, wetting the insulating towels, thereby raising their thermal conductivity and increasing the risk of burns. The focal pressure from lying on a hot pack can also produce increased heating over bony prominences. Lying on the hot pack also puts the pressure of the weight of the body on the skin next to the pack. This pressure exceeds capillary pressure and makes it difficult for blood to come into the area to carry away the heat. Hot packs are among the more common causes of burns in physical therapy. This is because heat has sedative effects, and the patient is typically not directly supervised when this modality is used.
Heating Pads
Two main types of heating pad are available: electric heating pads and circulating fluid pads. Electric heating pads usually control heat output by regulating current flow. Circulating fluid (e.g., water) heating pads usually control heat output thermostatically. Peak temperatures of nearly 52° C (125° F) were achieved with an electric heating pad set on the lowest setting.50 Periodic temperature oscillations of up to 5° C were also noted.50 General heat precautions should be observed with heating pads. There is an obvious potential for electric shock, particularly when used in conjunction with moist toweling. Many commercially available heating pads are designed to be used in conjunction with moist toweling, but caution should be taken to inspect them regularly to ensure that all insulating materials are intact. The patient should not lie on a heating pad because this can result in focal temperature increases, leading to burns. This is of particular concern in the slender or cachectic patient with minimal subcutaneous adipose over bony prominences. Figure 20-3 shows a typical heating pad burn. The patient was an elderly woman who had not received adequate instruction in home use of her modalities, and had been repeatedly lying supine on an electric heating pad. Repeated and prolonged skin exposure to heat can result in erythema ab igne, a skin condition characterized by reticular pigmentation and telangiectasia, which has been noted after the use of a variety of superficial heating modalities.52
Radiant Heat
Radiant energy, including infrared radiation, is emitted from any substance with a temperature above absolute zero. Infrared is the portion of the electromagnetic spectrum adjacent to the long-wavelength, low-frequency (red) end of the visible spectrum. Luminous infrared heat lamps emit radiation in the near-infrared spectrum (wavelength 770 to 1500 nm), and nonluminous infrared heat lamps emit radiation in the far-infrared spectrum (wavelength 1500 to 12,500 nm).112 Infrared produces heating by inducing molecular vibration.52 A 1.3° C temperature rise has been noted at a depth of 2 cm after heat lamp application.122
The main determinants of intensity of radiant heating are distance and angle of delivery. The inverse square law states that the intensity of radiation varies inversely with the square of the distance from the source (Figure 20-4). This means that doubling the distance from a heat lamp reduces the heating intensity by a factor of four. Conversely, decreasing the distance from the heat lamp by half would increase the intensity fourfold. Typical distances are 30 to 60 cm from the patient’s body, depending on heat lamp wattage.112 The angle of delivery also affects the intensity of thermal radiation. Maximal radiation is applied when the source of radiation is perpendicular to the surface. As the angle away from perpendicular increases, the intensity of radiation decreases in proportion to the cosine of the angle.
Radiant heat is often preferable in patients who cannot tolerate the weight of hot packs. Caveats for the use of radiant heat include general heat precautions, light sensitivity, skin drying, and dermal photoaging.52,112 Some laboratory data suggest a potential for infrared acting synergistically with ultraviolet radiation in cutaneous photocarcinogenesis, but the clinical significance of this, if any, remains to be determined.52
Fluidotherapy
Fluidotherapy is a superficial, dry heating modality that uses convective heating with forced hot air and a bed of finely divided solid particles.20 This solid-gas system reportedly behaves like a heterogeneous fluid of low viscosity, a phenomenon labeled fluidization.19 Reputed advantages include the massaging action of the highly turbulent solid-gas mixture and the freedom to perform range-of-motion exercises.19 Peak hand muscle and joint capsule temperatures of nearly 42° C (107.6° F), and peak foot muscle and joint capsule temperatures of approximately 39.5° C (103.1° F), have been achieved after 20 minutes of fluidotherapy at 47.8° C (118° F).20 Both the temperature and the amount of agitation can be controlled. The typical temperature range is 46.1° to 48.9° C (115° to 120° F). Decreased degrees of agitation can be used for sensitive areas. Although fluidotherapy is touted for use in a variety of conditions, further study is needed to more objectively define its role in physical medicine.89 General heat precautions should be observed, and infected wounds should probably be avoided because of the risk of cross-contamination.
Paraffin Baths
A paraffin bath is a superficial heating agent that uses conduction as the primary form of heat transfer. Paraffin wax and mineral oil are mixed in a ratio of 6:1 or 7:1.112 Treatment temperatures are 52.2° to 54.4° C (126° to 130° F). These are tolerated because of the low heat conductivity of the paraffin mixture.112 A thermometer should be used to ensure proper temperature. A thin film of unmelted paraffin on the tank walls generally indicates a safe temperature. Methods of application include dipping, immersion, and brushing. After removing all jewelry, thoroughly wash and dry the area to be treated. The dipping method involves 7 to 12 dips followed by wrapping in plastic and towels or insulated mitts to retain heat.2 The immersion method involves several dips to form a thin glove of paraffin, followed by immersion for 30 minutes.2 The brushing method involves brushing on several coats of paraffin, followed by covering with towels. The brushing method is more cumbersome, and infrequently used in the adult population. However, children often find paraffin brushing to be fun, thereby improving their treatment compliance. Paraffin brushing can also be useful for areas difficult to immerse. For home treatment, patients can use a double boiler, although commercial paraffin tanks are reasonably inexpensive, probably safer, and more convenient. The equipment safety precautions should be carefully reviewed. General heat precautions apply to paraffin use. Open wounds and infected areas should be avoided.
The immersion method produces the greatest quantity and duration of temperature increase, with peak forearm subcutaneous tissue temperatures of 5.5° C over baseline, and brachioradialis temperatures of 2.4° C over baseline.2 The dip method produces a 4.4° C peak forearm subcutaneous tissue temperature rise and a 1.0° C brachioradialis temperature rise, but these temperature rises decrease significantly by 15 to 20 minutes postdipping.2 In a study of patients with scleroderma, paraffin baths in conjunction with friction massage and active range-of-motion exercise resulted in statistically significant improvement in skin compliance and overall hand function.6 In a study of patients with rheumatoid arthritis, statistically significant improvements in range of motion and grip function were noted after paraffin treatment in conjunction with active range-of-motion exercises, whereas paraffin baths alone had no statistically significant effect.47 This again emphasizes the importance of using exercise in combination with the modality.200,201
Deep Heat
Diathermy is derived from dia (“through”) and therme (“heat”), and refers to several forms of deep heating, namely shortwave, microwave, and ultrasound. Because the target tissue in physical medicine is generally muscle, tendon, ligament, or bone (rather than skin or subcutaneous fat), the goal of early investigators was to discover a mode of heating that minimized skin and subcutaneous tissue heating but maximized heating of deeper tissues.80 The challenge of diathermy developers was to discover a modality that could penetrate the skin and subcutaneous fat to produce a maximum temperature rise in underlying soft tissues. Conversely, the modality should not produce excessive temperatures in more superficial tissues (subcutaneous fat, being relatively avascular compared with muscle, is unable to adequately dissipate heat via vasodilatation). The therapeutic target temperature is generally considered to be 40° to 45° C (104° to 113° F).80 Lower temperatures might not produce adequate vasodilatation and increased metabolism, whereas higher temperatures can result in tissue damage. Because the thermal pain threshold is approximately 45° C (113° F), pain perception can be used to monitor intensity of heating in the neurologically intact and alert person.80 One gradually increases modality intensity to the earliest pain perception, then slightly decreases intensity. There is, however, only a fine line between the therapeutic temperature range and potential thermal injury.
Ultrasound
Ultrasound is defined as acoustic vibration with frequencies above the audible range (i.e., >20,000 Hz). Medical uses of ultrasound can be diagnostic or therapeutic. Diagnostic ultrasound is used for a variety of obstetric, urologic, cardiovascular, and other imaging studies, and is outside the scope of this chapter. Therapeutic ultrasound involves the use of high-frequency acoustic energy to produce thermal and nonthermal effects in tissue. Ultrasonic signals are typically generated using the reverse piezoelectric effect. Certain quartz crystals and synthetic ceramics have piezoelectric characteristics, such that when they vibrate they produce an electric current.188 The reverse happens when an electric current is passed across such crystals, causing vibration at a specific frequency.
As ultrasonic waves travel through tissue, they lose a proportion of their energy, a process called attenuation.208 Attenuation in tissue is produced by several mechanisms: absorption, beam divergence, and deflection.208 Absorption is the major cause of ultrasound attenuation.208 Ultrasonic energy is absorbed by the tissue and is ultimately converted into heat.208 For most tissues, attenuation increases as frequency increases, so a 1.0-MHz signal would penetrate deeper than a 3.0-MHz signal because of its lower attenuation by the tissue.208 Beam divergence is the amount that the beam spreads out from the transducer. Beam divergence decreases as frequency increases, so a higher frequency signal has a more focused beam.
Deflection includes the processes of reflection, refraction, and scattering.208 The angle of the reflected wave is equal to the angle of the incident wave (Figure 20-5). The magnitude of the reflected wave depends on the difference in acoustic impedance between the tissues on each side of the reflecting surface.208 Acoustic impedance is a measure of the resistance to the transmission of a sound wave, and is the product of the velocity of sound and the density of the medium.188,208 The magnitude of the reflected wave is proportional to the following formula208:
where A2 is the acoustic impedance of tissue 2 and A1 is the acoustic impedance of tissue 1. The greater the mismatch of acoustic impedance of the two tissues, the greater the magnitude of the reflected wave. Because the impedance mismatch between skin and air is extremely high, essentially all the acoustic signal is reflected if a coupling agent is not used (see below).208 Refraction is a deviation of beam direction as it is transmitted between two media (see Figure 20-5). The angle of the transmitted (refracted) wave is determined by the velocity of sound in the two media, and is given by Snell’s law208:
where sine 1 is the sine of the incident wave, sine 2 is the sine of the transmitted wave, velocity 1 is the velocity of sound in the first tissue, and velocity 2 is the velocity of sound in the second tissue. Scattering is the last mechanism of beam deflection. It occurs when surface irregularities scatter the signal. Scattering is minimized when surface irregularities are small with respect to the wavelength.
Parameters for therapeutic ultrasound are noted in Table 20-2. Frequency is generally in the range of millions of cycles per second (or megahertz). The most commonly used frequencies in the United States are in the range of 0.8 to 1.1 MHz, although frequencies around 3.0 MHz are also fairly common. Power is total energy per unit time, whereas intensity is power per unit area. Intensity can be expressed in terms of peak or average intensity, and spatial or temporal intensity, and is indicated in units of watts per square centimeter. These different measures of intensity are defined in Table 20-2. The World Health Organization and the International Electrical Commission both recommend limiting spatial average intensity to 3 W/cm2.88 Most clinically used intensities of therapeutic ultrasound are in the range of 0.5 to 2.0 W/cm2. Temperatures of up to 46° C (114.8° F) in deep tissues (e.g., bone-muscle interface) are easily achieved with ultrasound.115–117 If very deep heating (e.g., of the hip joint) is the goal, ultrasound appears to be superior to microwave or SWD.121
Table 20-2 Ultrasound Parameters
Parameter | Details |
---|---|
Frequency | Millions of cycles per second (MHz) |
Power | Total energy per unit time (W) |
Effective radiating area | The area of the transducer that actually radiates ultrasonic waves (cm2) |
Intensity Spatial average intensity Spatial peak intensity Temporal average intensity Temporal peak intensity | May be expressed in terms of peak or average and spatial or temporal (W/cm2) Total power output divided by effective radiating area Maximal intensity anywhere within the beam Average intensity of “on” and “off” periods of a pulsed signal Maximal intensity of “on” period of a pulsed signal |
Duration | Generally 5-10 minutes per site |
Additional parameters for pulsed ultrasound Pulse duration Pulse repetition period Pulse repetition frequency Duty factor | Time of actual ultrasound pulse (“time on”) Time interval from one pulse to the next (“time on” and “time off”) Number of pulses per second Fraction of total time during which ultrasound is emitted (calculated by dividing pulse duration by pulse repetition period) |
Ultrasound delivery can be continuous or pulsed. Pulsed delivery involves the emission of brief bursts or pulses of ultrasound, interspersed with periods of silence (Figure 20-6). For pulsed ultrasound delivery, additional parameters should be indicated.188 These include pulse duration, pulse repetition period, pulse repetition frequency, and duty factor, and are defined in Table 20-2. In most machines available for use in the United States, selecting a duty factor automatically determines the other parameters. Duty factor commonly ranges from 10% to 50%. For example, a 2-ms pulse duration might be interspersed with 8 ms of silence. The pulse repetition period would be 10 ms, the pulse repetition frequency would be 100 Hz, and the duty factor would be 20%. Pulsed delivery, especially at low duty factors, results in less heating than continuous wave ultrasound, and thereby presumably emphasizes nonthermal effects.
The physiologic effects of ultrasound can be divided into thermal and nonthermal effects. Thermal effects are produced when acoustic energy is absorbed, producing molecular vibration, which results in heat production.36 Nonthermal effects include cavitation, media motion (acoustic streaming, microstreaming), and standing waves. Cavitation is the production of gas bubbles in a sound field.36 These bubbles can expand and contract with alternating compressions and rarefactions of a sound wave.36 Stable cavitation refers to bubbles that oscillate in size within the sound field.57 Unstable cavitation refers to bubbles that continue to grow in size and then collapse. The high temperatures and pressures generated by this can produce platelet aggregation, localized tissue damage, and cell death.57 Both forms of cavitation are capable of mechanical distortion, movement of material, and alteration of cellular function, but their clinical significance is not yet clearly defined.36,138
Media motion includes acoustic streaming and microstreaming. Acoustic streaming is defined as unidirectional movement in an ultrasonic pressure field, and results from an ultrasonic wave traveling through a compressible medium.57 Acoustic microstreaming is produced by stable cavitation. As the stable cavitation bubbles oscillate in size, the surrounding fluid is set in motion, with nearby particles being attracted to the oscillating bubble.57 In addition to movement of material, acoustic streaming and microstreaming can result in cell membrane damage and accelerate metabolic processes.57
Standing waves are produced by the superimposition of incident and reflected sound waves, and can result in focal heating at tissue interfaces of different densities.57 Stasis of red blood cells at one-half wavelength intervals in a sound field has also been demonstrated in the laboratory.58 Although numerous subcellular, cellular, and tissue nonthermal ultrasound effects have been reported, their clinical significance remains to be elucidated.36,43,57,58,138 Certain measures can minimize the nonthermal effects of ultrasound. Higher frequency, lower intensity, and pulsed delivery mode minimizes acoustic cavitation.43 Stroking technique of application minimizes standing wave formation.57
As noted previously, the amount of ultrasound reflected at an interface between two media depends on the difference in acoustic impedance, so the ideal coupling medium is one with acoustic impedance similar to that of tissue. Three factors have an impact on the effectiveness of a coupling medium:
Degassed water is a commonly used coupling medium and is used to prevent bubble formation on the skin surface. Allowing tap water to stand overnight typically allows for adequate gas evaporation. In tests of acoustic transmissivity, mineral oil and several commercially available coupling gels had similar transmissivities to the reference standard of distilled degassed water.195 However, the hydrocortisone phonophoresis coupling agents tested had a significantly lower transmissivity, presumably related to microscopic air bubbles introduced into the media. Variation in transducer pressure produced dramatic differences in transmissivity, which outweighed the differences between the various coupling media. The investigators concluded that coupling media can be chosen primarily on the basis of cost and convenience, without compromising function.195 Encased silicon gel shows promise for use as a coupling agent over irregular body surfaces, sensitive skin areas, and open wounds, if the issue of impedance mismatch with the sound head can be rectified.8 In addition to accommodating for impedance mismatches, coupling media also lubricate to permit smooth movement of the transducer over the skin.
The most common technique of ultrasound application is the stroking technique. It allows a more even energy distribution over the site being treated. The applicator is moved slowly over an area of approximately 25 cm2 (4 square inches) in a circular or longitudinal manner.112 The applicator size (usually 5 to 10 cm2) limits the size of the area that can be treated, so multiple fields of treatment might be needed for larger areas (i.e., shoulder and hip may require anterior, lateral, and posterior ports). The stationary technique generally should be avoided because of the potential for standing waves and the production of hot spots.148 Intensity is indicated by specifying the particular watts per square centimeter or by titrating to just below pain threshold. In surveys of performance of ultrasonic therapy equipment, variations in frequency and power output were common.88,182 Although the frequency of commercially available ultrasound applicators was typically within 5% of manufacturer’s specifications, the overwhelming majority (85%) of applicators tested had power output variations of over 20%.182 Consequently it is important to have ultrasound applicators recalibrated regularly.
More than 35 clinical uses of ultrasound have been described, but many of these are not well supported by experimental evidence.186 More recent systematic reviews have not conclusively shown the benefits of ultrasound in the care of a number of musculoskeletal and related conditions, for similar lack of evidential support.∗ The purported clinical uses of ultrasound continue to expand, however, as witnessed by the current clinical interest in fracture healing, especially of bony nonunions,63,64,102, 104 and in chronic wound healing.†
Ultrasound precautions are summarized in Box 20-6.133,148,186 Concern about the use of heat near malignancies was discussed previously. Deep heating over an open epiphysis could result in either increased growth (from hyperemia) or decreased growth (from thermal injury). Avoiding ultrasound near pacemakers is reasonable because of potential thermal or mechanical injury to the pacemaker. Ultrasound over laminectomy sites could theoretically result in spinal cord heating. Ultrasound at therapeutic dosage over the peroneal nerve has been shown to produce a reversible conduction block in some patients with polyneuropathy.94 There are also case reports of increased radicular pain with ultrasound.72 There are even case reports of patient abuse of ultrasound.125 The concern with ultrasound use over arthroplasties and other metallic implants is the potential for focal heating. Gersten71 reported that temperature rises near metal were actually lower than temperature rises near bone, so metal per se should not be a contraindication to ultrasound. Lehmann112 cautioned against the use of ultrasound near methyl methacrylate or high-density polyethylene because of their high coefficient of absorption. The effect of ultrasound on bony ingrowth arthroplasties is not yet well defined. Because the effects of ultrasound on arthroplasties are not yet completely delineated, the most prudent course would be to avoid ultrasound over these areas whenever possible.
Box 20-6 Ultrasound Precautions
< div class='tao-gold-member'>
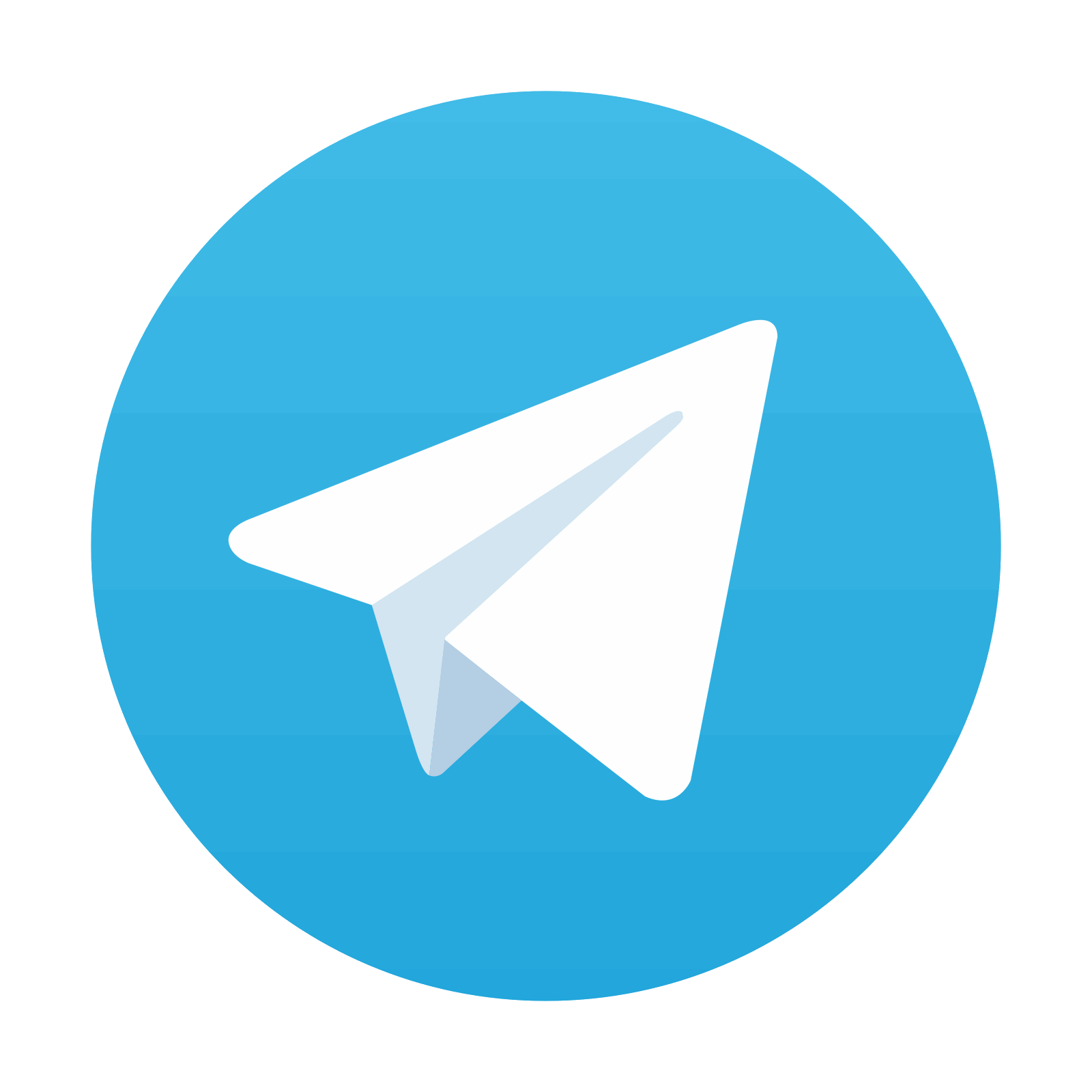
Stay updated, free articles. Join our Telegram channel

Full access? Get Clinical Tree
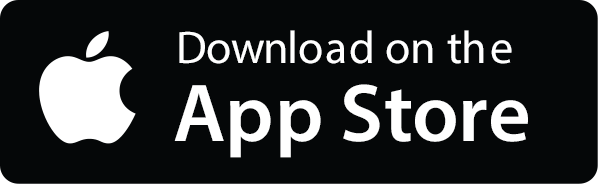
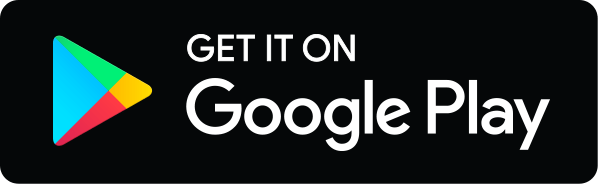