Chapter 112 Phage Therapy
Bacteriophages as Natural, Self-limiting Antibiotics
Introduction
Phage therapy involves the use of bacteriophages—viruses that only attack bacteria and are very host specific—to kill pathogenic microorganisms. The art was first developed at the Pasteur Institute in Paris early in the twentieth century, but since the advent of chemical antibiotics in the 1940s, it has been little used in the West. Today, however, the increased prevalence of bacteria that are resistant to most or all available antibiotics is precipitating a major health crisis, as was again passionately stressed by the World Health Organization in their call to action on World Health Day, 2011.1 Here, we summarize the evidence that the available data strongly support expedited evaluation of phage therapy to help address this serious menace.2–14
Extensively documented results of French and Eastern European therapeutic phage applications are encouraging, but most involved individualized applications to infections recalcitrant to all other available treatments rather than double-blind clinical trials. There have also been encouraging recent developments. In 2006, the U.S. Food and Drug Administration (FDA) and the European Union (EU) both approved phage preparations targeting Listeria monocytogenes on ready-to-eat foods. The Nestlé Corporation is currently carrying out an extensive project in Dhaka, Bangladesh, to study the safety and efficacy of phage therapy in treating enterotoxigenic Escherichia coli and enteropathogenic E. coli induced diarrhea in children.15 Either of two preparations, a novel cocktail of T4-like phages used in earlier safety trials as well as a commercially available Russian anti-E. coli phage cocktail (Microgen), or a placebo is being added to the standard oral rehydration solution currently in use in double-blind fashion. This work demonstrates all the key elements of modern clinical trials. The phages being used for the main experimental arm of the trial were isolated from the stools of pediatric diarrhea patients in Bangladesh, with the broad-spectrum ones applicable for phage therapy all turning out to be members of the highly studied T4 family (see the following).16 They have also reported the details of their very extensive genomic, mouse, and human safety studies of representative phages in their set.17
Historical Context
Discovery and Early Research
Edward Twort and Félix d’Hérelle independently reported the isolation of filterable entities capable of lysing bacterial cultures and of producing multiple small cleared areas on bacterial cultures when diluted, rather than showing a minimum inhibitory concentration, implying that discrete particles were involved. They are jointly given credit for the discovery of bacteriophages. It was d’Hérelle, a Canadian working at the Pasteur Institute in Paris, who gave the name bacteriophage to these entities he discovered in the stools of soldiers recovering from dysentery—using the suffix phage “not in its strict sense of to eat, but in that of developing at the expense of.”18 He soon also isolated phages targeting avian typhosis and tested their application during an outbreak in French chickens, showing that three quarters of the untreated chickens died, but all of the infected chickens treated with phages survived. He then accepted a request to treat several children battling dysentery at the Paris Hôpital des Enfantes Malades; the day before treating the first child, he and several assistants swallowed far more phages than they would be administering, thus carrying out the first known Phase I clinical trial. This trial was fully successful, but d’Hérelle turned to intense study of phages before publishing the results or carrying out further therapeutic applications. Thus, the first-known publication discussing successful phage therapy was published in 1921 from Belgians Bruynoghe and Maisin,19 who used phages to treat staphylococcal skin infections.
In a 300-page 1922 book, The Bacteriophage: Its Role in Immunity,18 d’Hérelle wrote the original, classic descriptions of plaque formation and composition, infective centers, the lysis process, host specificity of adsorption and multiplication, the dependence of phage production on the precise state of the host, isolation of phages from various sources, and the factors controlling stability of the free phage. He quickly became fascinated with the apparent role of phages in the natural control of microbial infections, and noted the frequent specificities of the phages isolated from recuperating patients for disease organisms infecting them and the rather rapid variations over time of the phage populations. Throughout his life, he worked to develop the therapeutic potential of properly selected phages against the most devastating health problems of the day, traveling to many parts of the world, teaching at Yale from 1928 to 1933, and establishing his own Laboratoire du Bactériophage in Paris, run by his son-in-law, Theodore Mazure, which produced the first commercial phage cocktails—Bacté-Coli-Phage, Bacté-Intesti-Phage, Bacté-Dysentérie-Phage, Bacté-Pyo-Phage, and Bacté-Rhino-Phage. Although France is clearly a western country, most of the literature reviews of phage therapy have ignored the successful continuation of phage therapy in France until the early 1990s, which largely used well-made phage preparations produced by d’Hérelle’s laboratory or by the Bacteriophage Service of the Lyon and Paris branches of the Institut Pasteur.
Key to d’Hérelle’s many successes was that he focused intensely on understanding phage biology and on applying that knowledge in his production and application of phages, including careful ongoing quality control, close work with physicians, and development of appropriate treatment modalities. He wrote several more detailed books on phages and phage therapy, two of which were also translated into English (1926 and 1930). The depth of his insights into the practice of phage therapy became more accessible via the recent publication of a translation of the appendix of his book.18 Through much of the time, he worked closely with George Eliava, director of the Georgian Institute of Microbiology, who had seen bacteriocidal action of the water of the Koura River in Tbilisi (Tiflis) that he could not explain until he became familiar with d’Hérelle’s work while spending 1920 to 1921 at the Pasteur Institute.18 Over the years, the two developed plans to found an Institute of Bacteriophage Research in Tbilisi as a world center of phage therapy, including scientific and industrial facilities and supplied with its own experimental clinics. A large campus on the river Mtkvari was allotted for the project in 1926. D’Hérelle sent supplies, equipment, and library materials. In 1934 to 1935, he visited Tbilisi for 6 months, set up his laboratory, and wrote a book, The Bacteriophage and the Phenomenon of Recovery,20 which was translated into Russian by Eliava and had a very strong impact on Stalin, and thus on the implementation of phage therapy in the Soviet Union. D’Hérelle intended to move to Georgia; his cottage still stands on the institute’s grounds. However, in 1937, Eliava was arrested as a “people’s enemy” by Beria, then head of the Georgian NKVD and soon to direct the Soviet NKVD as Stalin’s much-feared henchman. Eliava was executed, sharing the tragic fate of many Georgian and Russian progressive intellectuals of the time, and d’Hérelle, disillusioned, never returned to Georgia. However, their Bacteriophage Institute survived under the leadership of a group of women well trained by Eliava and d’Hérelle. The Institute was put under the People’s Commissary of Health of Georgia in 1938 and was transferred to the All-Union Ministry of Health in 1951, taking on the leadership role in providing bacteriophages for therapy and bacterial typing throughout the Soviet Union. Hundreds of thousands of pathogenic bacterial samples were sent to the Institute from throughout the Soviet Union to isolate and produce more effective phage strains and to better characterize their usefulness. A new five-story building provided facilities for making two tons of phage products two or more times a week, with 80% of the product going to the Soviet military. These included tablets against dysentery, pyophage cocktails targeting wound and other purulent infections, as well as intestiphage intended for a wide range of enteric pathogens. Much of the main building held research laboratories continually isolating new phages, upgrading therapeutic cocktails, working on anaerobes and defenses against potential biowarfare, and collaborating closely with military and civilian physicians to test the phage preparations and ways of administering them. These were the golden years of the Institute, when its employees were among the best paid in Tbilisi, and there were no shortages of supplies, new research targets, or potential customers. In 1988, an official Scientific Industrial Union “Bacteriophage” was formed, centered in Tbilisi with branches in Ufa, Habarovsk, and Gorki.
Initial Attempts at Commercialization
Phage therapy has been explored extensively, with successes being reported for a variety of diseases, including dysentery, typhoid and paratyphoid fevers, pyogenic and urinary tract infections, and cholera.4 Phages have been given orally, through colon infusion, and as aerosols as well as infused directly into lesions. They have also been given as injections: intradermal, intramuscular, intraduodenal, intraperitoneal, into the pericardium and arteries leading to infected areas, as well as intravenously. The early strong interest in phage therapy is reflected in some 800 papers published on the topic between 1917 and 1956. The reported results were quite variable. Many of the physicians, entrepreneurs, and pharmaceutical firms who initially became very excited by the potential clinical implications, especially in the pre-antibiotic era, jumped into application efforts with very little understanding of phages, microbiology in general, or the basic scientific process. Thus, many of the studies were anecdotal and/or poorly controlled; many of the failures were predictable; and some of the reported successes did not make sense in light of current knowledge. Too often, uncharacterized phages at unknown concentrations were given to patients without previous specific bacteriologic diagnosis, and there is no mention of follow-up, controls, or placebos. Much of the understanding gained by d’Hérelle was ignored in this early work, and inappropriate methods of preparation, “preservatives,” and storage procedures were often used. On one occasion, d’Hérelle reported testing 20 preparations from various companies and finding that not one of them contained active phages! Not surprisingly, a check of the product showed that one phage had out-competed all the others, and this was not a polyvalent preparation. In general, there was little essential quality control except in a few research centers. Large clinical studies were rare, and the results of those few that were carried out were largely inaccessible outside of what was then the Soviet Union.
Specific Problems of Early Phage Therapy Work
• Paucity of understanding of the heterogeneity and ecology of the phages and the bacteria involved
• Lack of availability or reliability of bacterial laboratories for carefully identifying the pathogens involved (important considering the relative specificity of phage therapy)
• Use of too few phages in infections that involved mixtures of different bacterial species and strains
• Failure to select obligatorily lytic phages against the target bacteria before using them in patients
• Emergence of phage-resistant bacterial strains, through selection of resistant mutants (especially if only one phage strain was used against a particular bacterium) or through lysogenization (if temperate phages were used, as discussed in “Properties of Bacteriophages”)
• Failure to appropriately characterize or titer phage preparations, many of which, even from major companies, were shown to be totally inactive21,21a
• Failure to neutralize gastric pH before oral phage administration
• Inactivation of phages by both specific and nonspecific factors in bodily fluids
All of these factors need to be taken into consideration as we now work to formally document phage efficacy and integrate phages into medical practice worldwide.
Properties of Bacteriophages
Viral particles are like spaceships that are able to transfer their genomes between susceptible cells where they can reproduce. In the case of bacteriophages, the targets are specific kinds of bacterial cells, specific to varying degrees for each phage; they cannot infect the cells of more complex organisms. Each virus consists of a long nucleic acid polymer (DNA or RNA) containing the genetic information that determines all of the properties of the virus, which is carried around packaged in a protein coat (Figure 112-1). Unlike eukaryotic viruses, most phages have tails, the tips of which have the ability to bind to specific molecules on the surfaces of their target bacteria (Figure 112-2). The viral DNA is then ejected through the tail into the host cell, where it directs the production of progeny phages; often more than 100 are produced in just half an hour from each bacterial cell.
Each strain of bacteria has characteristic protein, carbohydrate, and lipopolysaccharide molecules present in very large quantities on its surface. These molecules are involved in forming pores, motility, and binding of the bacteria to particular surfaces. Each such molecule can act as a receptor for particular phages. Development of resistance to a particular phage generally reflects mutational alteration or loss of its specific receptor; this loss frequently has negative effects on the bacterium, often making such mutants less virulent, and does not confer protection against the many other phages that use different receptors. Each kind of bacterium has its own phages, which can generally be isolated wherever that bacterium grows: from sewage, feces, soil, even ocean depths and hot springs, but finding phages suitable for therapeutic applications is much easier for some species than for others; this relates significantly to the ability to grow the host in the laboratory. The process of isolation is straightforward for phages targeting many of our best-studied pathogens. An environmental sample is combined with an appropriate nutrient-fortified solution and several targeted bacteria, incubated over night, and 10 µL each of a series of ten-fold dilutions spotted on a nutrient-agar plate prepared with a single strain of target bacteria. The next day, a dense bacterial lawn is seen, hopefully with cleared spots and, at some dilution, dotted with small, cleared plaques. Each plaque contains about a billion phages, all of them progeny of a single initial phage. Individual plaques are plucked and cultured to give a homogeneous stock of that particular phage, whose host ranges and other properties can then be studied.
One major source of confusion in the early phage work was that new phages were often isolated by each laboratory, so there was little continuity or basis for comparison. Key technical developments in the 1930s to 1940s that helped clarify the general nature and properties of bacteriophages were (1) the concentration and purification of some large phages by means of very high-speed centrifugation and the demonstration that they contained equal amounts of DNA and protein; and (2) visualization of phages by the electron microscope (EM).22,23 Phages specific for virtually every well-studied bacterial species have now been isolated, and increasingly, many are becoming well classified.
Using the EM, each phage family was found to have its own specific shape and size, from “lunar lander”–style complexity with a contractile tail and long tail fibers attached to a baseplate, to globular heads with long or short tails, to the small filamentous phages that looked much like bacterial pili (Figure 112-3). With recent advances in DNA sequencing techniques, our understanding of various phages is exploding in powerful and important ways; the genomes of several hundred phages, infecting a variety of organisms, have now been sequenced, revealing a remarkable variety of types and properties that can be very important when considering potential therapeutic applications. In general appearance, 95% of the studied phages belong to one of the three general tailed morphotypes: the short-tailed podoviridae, the siphoviridae with long, often flexible tails; and the myoviridae, with tails composed of an inner tube and an outer contractile sheath attached to a complex baseplate. There are both obligatorily lytic and temperate phage families with each of these three general morphotypes, so other kinds of data are also needed to tell whether a newly isolated phage is temperate or lytic.
Obligatorily Lytic Phages and the Development of Molecular Biology
A much better understanding of the interactions between the lytic lifecycle of phages and bacteria began with one-step growth curve experiments. These demonstrated an eclipse period, during which the DNA began replicating and there were no free phages in the cell; a latent period of accumulation of intracellular phages; and a precisely timed lysis process that released the phage to go in search of new hosts. This phage infection cycle is illustrated in Figure 112-4 for coliphage T4, which does a particularly effective job of shutting off all host functions and whose family is very widespread in nature and in therapeutic phage preparations for enteric bacteria.
The temperate phages generally encode repressors to turn off most of their own genes when they are in the temperate pro-phage state, integrases to let them insert themselves into the DNA of their hosts, and excision enzymes to cut their DNA back out to enter a lytic cycle. Obligately lytic phages generally have more extensive mechanisms for shutting off the host, often involving nucleases and transcription factors, as well as a variety of small proteins made under the control of strong promoters very early in infection to re-direct cellular metabolism; T4, for example, encodes at least 50 such proteins.
Phage Interactions in the Body
A number of early experiments involving phage injection into animals led to widespread belief that phage therapy could not succeed because the phages were too rapidly cleared by the immune system. Early experiments in rabbits, rats, and mice showed rapid disappearance of phage from the blood and organs, but long-term survival in the spleen.24,25 However, these experiments were done in the absence of host bacteria in which the phage could multiply and were carried out by the unnatural mode of intravenous injection, exposing the phage almost immediately to the reticuloendothelial system. Many later studies made it clear that phages are seen in the mammalian circulatory system for prolonged periods when they are entering it from some sort of reservoir in other tissues, and the host is dealing with infection by a bacterium in which they can replicate —precisely the sort of situation seen in phage therapy as currently practiced.
This pattern is particularly clear in research published in 1943 by noted Harvard bacteriologist René Dubos26 (Figure 112-5). Dubos et al26 injected mice intracerebrally with a dose of a smooth Shigella dysenteriae strain that was sufficient to kill more than 95% of the mice in 2 to 5 days and treated them with intraperitoneal injection of a mixture of phages that had been isolated from New York City sewage, grown in the same bacteria, and purified only by sterile filtration. With no treatment or when treated with filtrates of bacterial cultures or with heat-killed phage, only 3 of 84 mice (3.6%) survived; in contrast, 46 of 64 (72%) of the mice given 107 to 109 phages survived. Pharmacokinetic studies on the mice showed that when phages were given to uninfected mice, they appeared in the bloodstream almost immediately, but the levels started to drop within hours and very few were seen in the brain, as shown in Figure 112-5. However, in infected animals, brain levels of viable phage appropriate to those bacteria soon far exceeded blood levels; around 107 to 109 phages/g were often seen between 8 and 114 hours after administration, with the level dropping between 75 and 138 hours after phage addition. After the first 18 hours, blood levels were far lower than brain levels, but phages were still present in blood at 104 to 105 phages/mL in those animals in which the brain levels were still high.
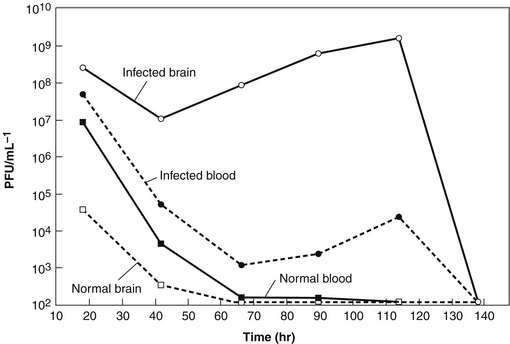
FIGURE 112-5 This figure, based on the data in the 1943 mouse studies of Dubos et al,26 provides significant insight into why phage therapy works well even in treating infections that antibiotics cannot treat. When the mice were injected intraperitoneally with 109 phage, they quickly appeared in the bloodstream and some even crossed the blood−brain barrier, but they were rapidly cleared. However, when the mice were also injected intracerebrally with Shigella dysenteriae, the host for these phages, 46 of 64 of the mice survived (compared with 3 of 84 in the absence of appropriate viable phage), and the brain level of phage climbed to over 109/g. Once the bacteria cleared, the level of phage dropped below detection limits.
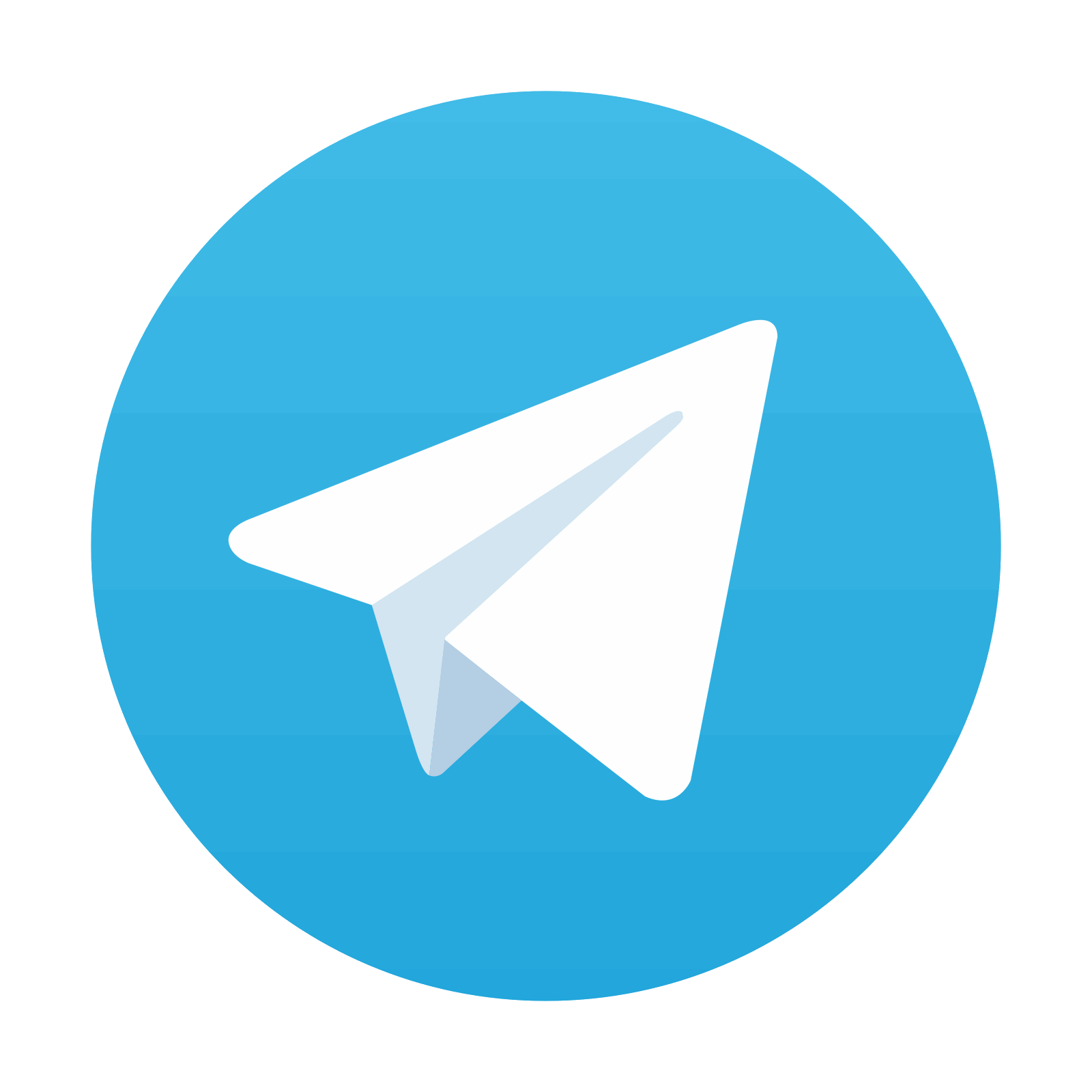
Stay updated, free articles. Join our Telegram channel

Full access? Get Clinical Tree
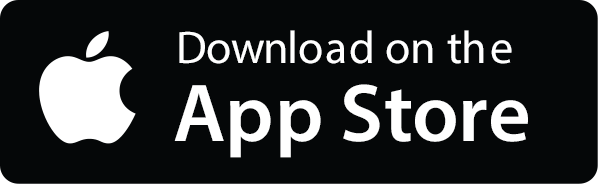
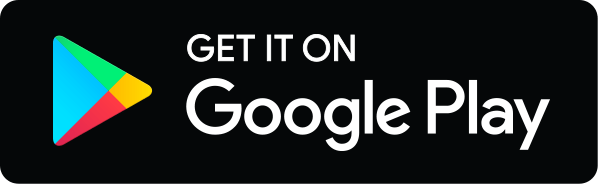