Periarticular Ligamentous Tissue: A Biochemical and Physiologic Assessment
David Amiel PhD
Sakae Sano MD, PhD

Although tendons and ligaments are composed primarily of fibrillar collagens, they have entirely different functions. Tendons are a conduit, connecting muscle to bone. Ligaments are short bands of fibrous tissue that bind bone to bone.
Collagen is the single most abundant animal protein in mammals. Collagen molecules assemble into characteristic fibers responsible for the functional integrity of tissues such as bone, cartilage, skin, ligament, and tendon.
The medial collateral ligament (MCL) of the knee is notable for rod-shaped and spindle-shaped cells that are intermediate in length compared with patellar tendon and anterior cruciate ligament (ACL) cells. The cellular morphological characteristics of the MCL are those of all fibroblasts, whereas the ACL cellular characteristics are similar to fibrocartilage cells.
A distinct form of collagen in cartilage, now known as Type II collage, was discovered about 30 years ago. Since then, many other unique molecular species have been observed. Types I, II, III, V, and XI collagen are categorized as fiber-forming collagens. They all exhibit lengthy, uninterrupted collagenous domains and are first synthesized as biosynthetic precursors (procollagens).
Proteoglycans consist of small amounts of protein bound to negatively charged polysaccharide chains referred to as glycosaminoglycans (GAGs). In articular cartilage, proteoglycans form a large portion of the macromolecular framework, but in ligaments they form only a small portion of this framework.
The ligaments of the knee are well-designed for their role in the maintenance of normal joint kinematics, with each ligament oriented in the direction needed to provide joint stability. Ligaments are well-designed connective tissues. Their densely packed collagen fiber bundles are arrayed in parallel along the length of the tissue substance to allow for the most efficient resistance of tensile loads.
Ligament insertions to bone are also well-adapted to their intended function. Force dissipation is achieved through a gradual transition from ligament to fibrocartilage to bone. Disruption is less likely to occur in this transition region than in the bone or peri-insertional tissue substance (1,2,3). For many years, tendons and ligaments have been classified together as dense, regularly arranged connective tissue (4,5,6). Although tendons and ligaments are composed primarily of fibrillar collagens, they have entirely different functions. Tendons are a conduit, connecting muscle to bone, thereby allowing movement of a joint complex through muscle contraction or relaxation. Ligaments are short bands of fibrous tissue that bind bone to bone and provide support for internal organs. In concert with the bony geometry and the dynamic effects of muscle and tendon (7,8) ligaments limit and guide joint motion.
Collagen is the single most abundant animal protein in mammals, accounting for up to 30% of all proteins (9). Collagen molecules assemble into characteristic fibers responsible for the functional integrity of tissues such as bone, cartilage, skin, ligament, and tendon (10). They contribute a structural framework for most organs. Crosslinks between
adjacent molecules are a prerequisite for the collagen fibers to withstand the physical stresses to which they are exposed. A variety of human conditions, normal and pathologic, involve the ability of tissues to repair and regenerate their collagenous framework. Many disabling conditions result from changes in the nature and organization of collagen (9).
adjacent molecules are a prerequisite for the collagen fibers to withstand the physical stresses to which they are exposed. A variety of human conditions, normal and pathologic, involve the ability of tissues to repair and regenerate their collagenous framework. Many disabling conditions result from changes in the nature and organization of collagen (9).

The histology of periarticular tendons and ligaments is a much-neglected area of investigation. Although many histology texts combine these two tissues as “dense, regular connective tissue” (11,12,13,14), certain ligaments and tendons are sufficiently characteristic to be distinguishable from each other based upon their histological appearance (15).
The variables considered are collagen bundle width, cell morphology and size, as well as “crimp.” Crimp is a feature of tendons and ligaments; it represents a regular sinusoidal pattern in the matrix. The periodicity and amplitude of crimp appear to be structure-specific features, and they are best evaluated under polarized light. A simple functional explanation for this accordionlike pattern in the matrix is that it provides a “buffer” in which slight longitudinal elongation may occur without fibrous damage. It also provides a mechanism for control of tension and acts as a “shock absorber” along the length of the tissue. When physiologic mechanical limits of this crimp are exceeded, however, irreversible damage occurs and the physical properties of the tissue are changed (16).
Although tendons and ligaments have crimping within their fascicles, there appear to be differences in the crimp pattern between these two structures (17). In the canine anterior cruciate ligament (ACL) and patellar tendon, two patterns of crimping are noted. The centrally located fascicles in the ACL are either straight or undulated in a planar wave pattern, whereas those located at the periphery are arranged in a helical wave pattern. In the patellar tendon, all the fascicles are found to undulate in the helical wave pattern.
The following histological description refers to rabbit tendons and ligaments unless otherwise specified. Microscopic examination of patellar tendon sections that have been stained with hematoxylin and eosin or evaluated directly under polarized light reveals the presence of longitudinally oriented bundles of collagenous tissue. These bundles are approximately 20 micrometers (μm) in width and have the characteristic crimp pattern of regular connective tissue. In patellar tendon, the crimp period is approximately 120 μm in length, with a corresponding amplitude of about 15 μm. On either side of the bundles or fascicles are spindle-shaped fibroblasts that are approximately 25 μm long. They are aligned longitudinally. Cytoplasm is indistinct, and only nuclei can be seen. More cellular areas within areolar connective tissue are observed. These sites, which are actually investing layers of tissue, are called “peritendineum” (Fig 1-1) and have been previously described as a site of reserve cells (18). They also mark the site of nerve and blood supply to the tendon.
![]() Fig 1-1. Histology of normal patellar tendon [H&E stain, original magnification 50×]. Note spindle-shaped fibroblasts, coarse fibrillar crimp, and peritendineum (arrow). |
Rabbit Achilles tendon demonstrates cell morphology, cell size, bundle width, and crimp period that are similar to those of patellar tendon. The crimp amplitudes of these tissues are different, with the Achilles tendon having almost three times (40 μm) the wave height relative to the patellar tendon. This difference may be related to an increased margin of shock absorbance for the Achilles tendon.
Histological assessment of the ACL demonstrates longitudinally oriented bundles of collagen with a width of about 20 μm as seen in the patellar tendon. The crimp period in the ACL, however, is considerably shorter (45 to 60 μm), and the amplitude is less than 5 μm. Fibroblasts are located on either side of the collagenous bundles, but the ligament is considerably more cellular than the tendon (Fig 1-2). ACL fibroblasts are round to ovoid and are substantially different in appearance from the fibroblasts in the patellar tendon. They measure about 5 to 8 μm in diameter and 12 to 15 μm in length. Cells are arranged longitudinally along the borders of the fascicles.
Like the patellar tendon, groups of cells, which concentrate in areolar connective tissue, are observed (Fig 1-2). These may be the ligamentous correlates to the peritendineum areas.
Like the patellar tendon, groups of cells, which concentrate in areolar connective tissue, are observed (Fig 1-2). These may be the ligamentous correlates to the peritendineum areas.
![]() Fig 1-2. Histology of normal ACL [H&E stain, original magnification 50×]. Note rounded fibroblasts, fine fibrillar crimp, and cluster of potential reserve cells (arrow). |
Table 1-1 Summary of Histologic Observations of Rabbit Periarticular Connective Tissue | ||||||||||||||||||||||||||||||
---|---|---|---|---|---|---|---|---|---|---|---|---|---|---|---|---|---|---|---|---|---|---|---|---|---|---|---|---|---|---|
|
The medial collateral ligament (MCL) of the knee is notable for rod-shaped and spindle-shaped cells that are intermediate in length compared with patellar tendon and ACL cells. The MCL has cells that are 15 μm long and 25 μm wide. The crimp period measures approximately 45 μm, with a 10 μm amplitude, and the collagen bundle width is approximately 20 μm, as seen in the other structures discussed. Measurements of cell size, shape, crimp specifics, and bundle width of the tendons and ligaments are provided in Table 1-1.
These substantial differences in morphology and ultrastructure may reflect the functional and environmental differences between these two periarticular ligaments, the ACL and MCL. The cellular morphological characteristics of the MCL are those of all fibroblasts, whereas the ACL cellular characteristics are similar to fibrocartilage cells. These observations lead to a series of profound and important questions concerning the differences in function, homeostasis, and repair between the ACL and MCL.
The biochemical parameters used to assess the constitutional properties of collagenous tissue include collagen structure and type, collagen reducible and nonreducible crosslink analysis, proteoglycan content, and glycoprotein. The value of each of these variables is related to its importance in the study of both (a) soft tissue injury and healing and (b) the response to exercise and the deleterious effects of immobilization. A more complete understanding of these problems could improve various treatment modalities and place them on firm scientific ground. This is particularly the case because most investigations of tissue injury and healing involve skin, not tendons or ligaments (19,20,21).

Collagen is the major protein in ligaments and tendons, and it is not a single entity. It is the single most abundant animal protein in mammals, accounting for up to 30% of all proteins. The collagen molecules, after being secreted by the cells, assemble into characteristic fibers responsible for the functional integrity of tissues such as bone, cartilage, skin, ligaments, and tendon (22). They contribute a structural framework to other tissues such as blood vessels and most organs. Crosslinks between adjacent molecules are a prerequisite for the collagen fibers to withstand the physical stresses to which they are exposed. Significant progress has been made toward understanding the functional groups on the molecules that are involved in the formation of such crosslinks, their nature, and location. A variety of human conditions, normal and pathologic, involve the ability of tissues to repair and regenerate their collagenous framework. Some of these conditions are characterized by excessive deposition of collagen (e.g., cirrhosis, scleroderma, keloid, pulmonary fibrosis, diabetes, etc.). After trauma or surgery, abnormal deposition of collagen may impair function (adhesions following repair of long tendons, scar formation during healing, etc.). In addition, many disabling conditions result from changes in the nature and organization of collagen (heart-valve lesions, osteoarthritis, rheumatoid arthritis, and congenital collagen diseases such as Marfan’s and Ehlers-Danlos syndromes, osteogenesis imperfecta, etc.).
The Collagen Molecule
The arrangement of amino acids in the collagen molecule is shown schematically in Figure 1-3. Every third amino acid is glycine. Proline and hydroxyproline follow each other relatively frequently, and the (gly, pro, hyp) sequence makes up about 10% of the molecule. This triple helical structure generates a symmetrical pattern of three left-handed helical chains that are, in turn, slightly displaced to the right, superimposing an additional “supercoil” with a pitch of approximately 8.6 nanometers (nm). These chains, known as α-chains, have a molecular weight of around 100 k Daltons and contain approximately 1,000 amino acids for the interstitial collagen Types I, II, and III (Fig 1-4). The amino acids within each chain are displaced by a distance h = 0.201 nm with a relative twist of 100 degrees, making the number of
residues per turn 3.27, and the distance between each third glycine 0.87 nm. The individual residues are nearly fully extended in the collagen structure, since the maximum displacement within a fully stretched chain would be approximately 0.36 nm. This separation is such that it will not allow intrachain bonds to form (as does occur in the alpha helix), and only interchain hydrogen bonds are possible. The exact number of hydrogen bonds that stabilize the triple helical structure has not been determined. One model describes two hydrogen bonds for every three amino acids, whereas another assumes one.
residues per turn 3.27, and the distance between each third glycine 0.87 nm. The individual residues are nearly fully extended in the collagen structure, since the maximum displacement within a fully stretched chain would be approximately 0.36 nm. This separation is such that it will not allow intrachain bonds to form (as does occur in the alpha helix), and only interchain hydrogen bonds are possible. The exact number of hydrogen bonds that stabilize the triple helical structure has not been determined. One model describes two hydrogen bonds for every three amino acids, whereas another assumes one.
In addition to these intramolecular conformational patterns, there seems to exist a supermolecular coiling. Microfibrils, possibly representing intermediate stages of packing, have been described.
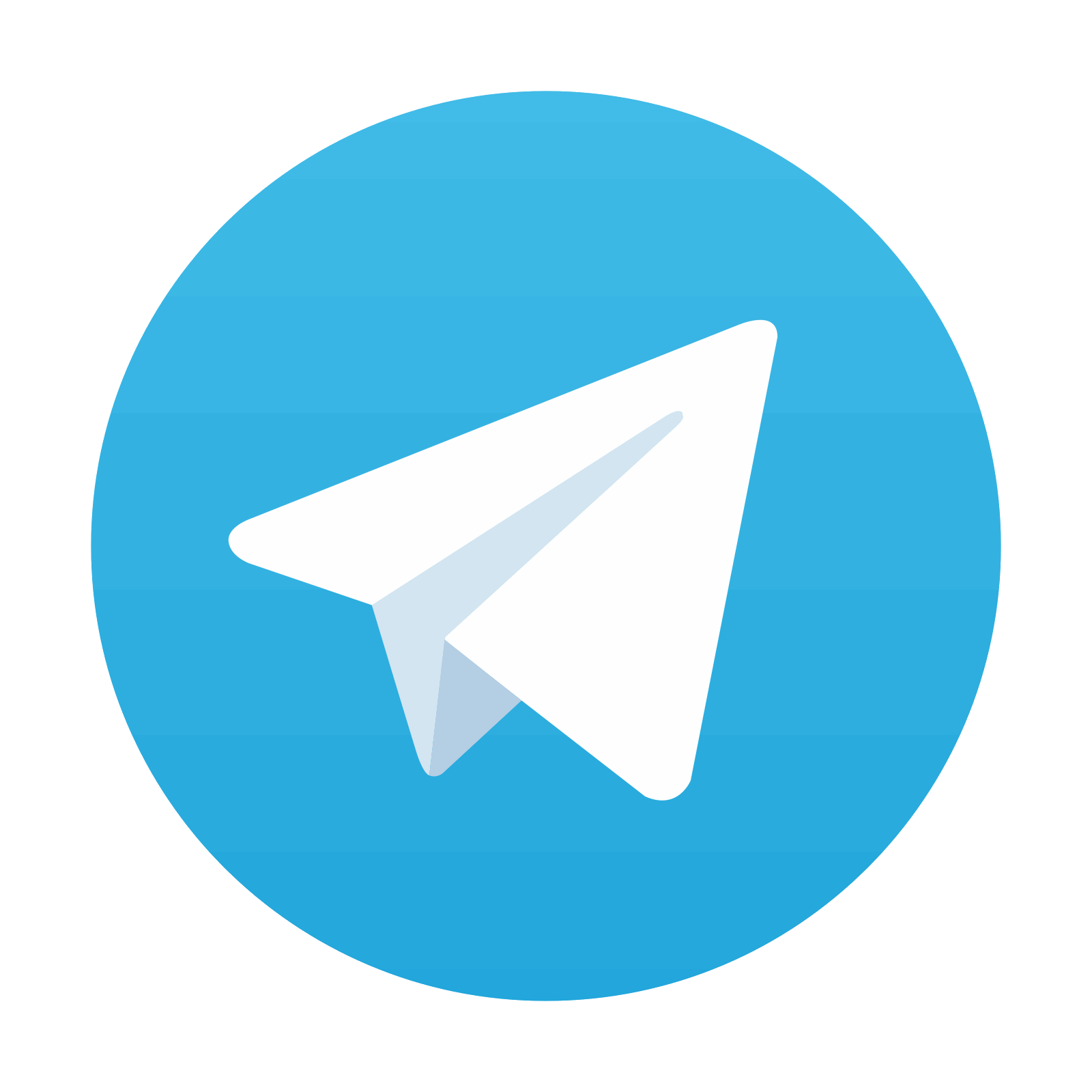
Stay updated, free articles. Join our Telegram channel

Full access? Get Clinical Tree
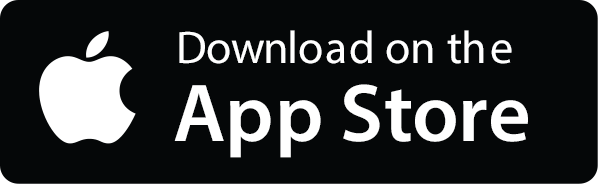
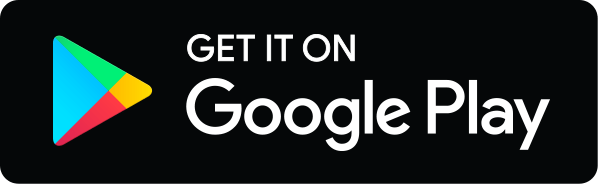