Stress Fractures in Athletes
Kurt P. Spindler MD
Michael S. George MD
Christopher C. Kaeding MD
Annunziato Amendola MD

Stress fractures behave differently according to their anatomical site and this must be considered when planning treatment.
Stress fractures result from repeated microtrauma leading to a macrofracture, which occurs when the repetitive microtrauma exceeds bone regeneration.
The three stages of fatigue failure are crack initiation, propagation along cement lines, and ultimate structural failure/complete fracture. Symptoms progress from insidious onset of pain with no definite history of trauma, to associated swelling and tenderness, then deformity with complete fracture.
Predisposing factors include: increased activity, abnormal alignment, decreased vascularity, old age, poor nutrition, hormonal imbalance, and genetic factors.
Radiographs lag behind bone scans by 1 to 2 weeks; bone scans are very sensitive but poor at monitoring bone healing; magnetic resonance imaging (MRI) has excellent sensitivity and displays precise extent of the injury; computed tomography (CT) scan best for bony detail and monitoring healing response. “Dreaded black line” in tibia represents a nonunion that should be treated operatively.
In general, fractures on the tension side tend to progress to complete fractures and often require operative fixation. Fractures on the compression side tend to be stable and unite with activity modification. In addition, fractures in areas of poor vascularity have a high risk of nonunion and may require operative treatment.
The tibial shaft is the most frequent of athletic stress fractures. The most common site is posteromedial or compression side, which can be treated with rest and activity modification as well as pneumatic braces.
Low-risk stress fractures are usually treated with activity modification and rest. Intermediate or high-risk fractures are treated with surgery if there is a clear fracture line on imaging or complete fracture. Intermediate or high-risk fractures are treated with a period of nonoperative management if no clear fracture line is visible on imaging. In all cases, default to surgical treatment if nonoperative therapy fails.
Stress fractures present a substantial concern to athletes in our society. Proper treatment of stress fractures is essential to avoid career ending injuries and unnecessary time off from athletics. Each anatomical site has unique healing characteristics and appropriate treatment strategies. Anatomical and physiological differences at each site affect the relative risk of complications. It is essential to understand the complex behavior of each stress fracture in order to effectively guide management.

Fractures in athletes may occur as either acute fractures such as in a major trauma or chronic fractures such as in a stress fracture. An acute fracture is the result of a single loading event in which the applied stress exceeds the strength of the bone. A stress fracture is the accumulation of microtrauma that ultimately leads to the macrofracture of bone.
The normal healing response of bone produces a dynamic balance between tissue breakdown and osteosynthesis.
According to Wolff’s law, stress loading of bone leads to bone remodeling and structural changes that dissipate the stress concentration in the bone. When osseous remodeling lags behind bone degradation, microfractures can accumulate and propagate. The fatigue life, or the number of cycles at a given applied load that leads to ultimate failure, may thus be exceeded in the absence of adequate healing of microfractures.
According to Wolff’s law, stress loading of bone leads to bone remodeling and structural changes that dissipate the stress concentration in the bone. When osseous remodeling lags behind bone degradation, microfractures can accumulate and propagate. The fatigue life, or the number of cycles at a given applied load that leads to ultimate failure, may thus be exceeded in the absence of adequate healing of microfractures.
Fatigue failure of bone typically occurs in three stages. Crack initiation first occurs at areas of discontinuity in the bone, such as in the haversion canals, lacunae, and canaliculi. In the absence of healing, microfractures propagate along cement lines. Stress loading parallel to these cement lines results in faster crack advancement. Finally, continued crack propagation results in ultimate structural failure and complete fracture.
Many factors may predispose certain athletes to stress fractures. These fractures commonly occur in otherwise healthy athletes who increase their activity to the point where repetitive microtrauma exceeds bone regeneration. Abnormal anatomical alignment may cause excess stress on particular bony structures. Decreased bone vascularity either from previous injury or from certain medical conditions may decrease the healing response of bone. Old age, poor nutritional status, hormonal abnormalities, and genetic factors may also predispose certain athletes to stress fractures (1).
Diagnosis
Stress fractures usually present with an insidious onset of pain without a history of a specific traumatic event (2). In the early stages, pain may be present only during the inciting activity. As the process continues, pain occurs during simple activities of daily living and may be associated with diffuse swelling and tenderness to palpation. The final stage of stress fracture is advancement to complete fracture with pain and deformity.
Thorough patient evaluation should always include a detailed review of nutritional status, hormonal abnormalities, current medications, and other medical conditions and treatments. Osteopenia may result from certain medications such as corticosteroids that are commonly used in the treatment of asthma and other conditions. Special attention must be paid to the possibility of the female athletic triad consisting of amenorrhea, disordered eating, and osteoporosis.
Diagnostic imaging includes plain radiographs,
bone scan, magnetic resonance imaging (MRI), and computed tomography (CT) scan. Fracture lines, sclerosis, and callus formation may be seen on plain radiographs, but usually do not appear until 1 to 3 weeks after the start of symptoms (3,4). Bone scan is very sensitive in the detection of stress fractures and usually displays changes 1 to 2 weeks before plain radiographs (5). Because bone scans remain positive for several months to over a year, they are not very useful for monitoring bony healing (3,5). MRI is very valuable in the detection of muscle and bone marrow edema and is as sensitive as bone scan in the detection of stress fractures. In addition, MRI allows precise anatomical detail and allows an excellent assessment of the extent of the injury (6,7). Bony architecture is best viewed on CT scan, which can be used to determine cortical incontinuity and to assess periosteal reaction, bridging callus, and sclerosis.
bone scan, magnetic resonance imaging (MRI), and computed tomography (CT) scan. Fracture lines, sclerosis, and callus formation may be seen on plain radiographs, but usually do not appear until 1 to 3 weeks after the start of symptoms (3,4). Bone scan is very sensitive in the detection of stress fractures and usually displays changes 1 to 2 weeks before plain radiographs (5). Because bone scans remain positive for several months to over a year, they are not very useful for monitoring bony healing (3,5). MRI is very valuable in the detection of muscle and bone marrow edema and is as sensitive as bone scan in the detection of stress fractures. In addition, MRI allows precise anatomical detail and allows an excellent assessment of the extent of the injury (6,7). Bony architecture is best viewed on CT scan, which can be used to determine cortical incontinuity and to assess periosteal reaction, bridging callus, and sclerosis.
Radiographic classification of stress fractures may be helpful in determining treatment. Type I stress fractures are subclinical fractures that are asymptomatic and are incidentally found on radiographs. These fractures are usually treated symptomatically. Type II fractures present with pain and are confirmed by bone scan or MRI, but are not evident on plain radiographs. These fractures are commonly referred to as bone strain, stress reaction, or early stress fracture. Type III fractures are symptomatic, nondisplaced fractures that can be seen on plain radiographs. Type IV fractures are displaced stress fractures that are usually treated operatively. Type V fractures are evident on plain radiographs as nonunions with sclerotic margins and no bridging callus formation. In the tibia, the “dreaded black line” heralds a type V fracture and should be treated operatively.
Stress fractures in different anatomical locations behave characteristically and should be considered individually. Suspected long bone fractures may best be evaluated by bone scans which offer a view of the entire extremity, while areas that require specific anatomical localization, such as the femoral neck, are better evaluated by MRI. A careful, systematic approach is critical to the proper diagnosis and treatment of stress fractures.
Treatment Principles
Specific anatomical locations may be classified as high, intermediate, or low risk based on the potential for the fractures to displace or exhibit poor healing. The likelihood of stress fracture complications is multifactorial. Fractures on the tension side of a bone have a predilection to progress to complete fractures because the stress load in tension tends to propagate the fracture lines. These fractures must be treated aggressively with strict nonweight-bearing in incomplete fractures and operative fixation in complete fractures. In contrast, compression side fractures tend to heal with activity modification alone because the stress load tends to approximate the fracture edges (Table 54-1).
Fractures that occur in watershed zones of poor vascularity must also be considered high risk (Table 54-2). These areas exhibit low healing potential and have a predilection for nonunion. A low threshold for operative treatment should be used in these troublesome areas.
Specific Fractures
Femoral Neck
Femoral neck stress fractures typically cause groin or hip pain, particularly at the extremes of motion. Diagnosis may be confirmed with either bone scan or MRI, although MRI may be more helpful in determining the exact anatomical location and extent of the fracture. Fracture appearance tends to be delayed 1 to 2 weeks on plain radiographs.
TABLE 54-1 Classification of Stress Fractures | ||||||||||||
---|---|---|---|---|---|---|---|---|---|---|---|---|
|
TABLE 54-2 Location of High Risk Stress Fractures | |||
---|---|---|---|
|
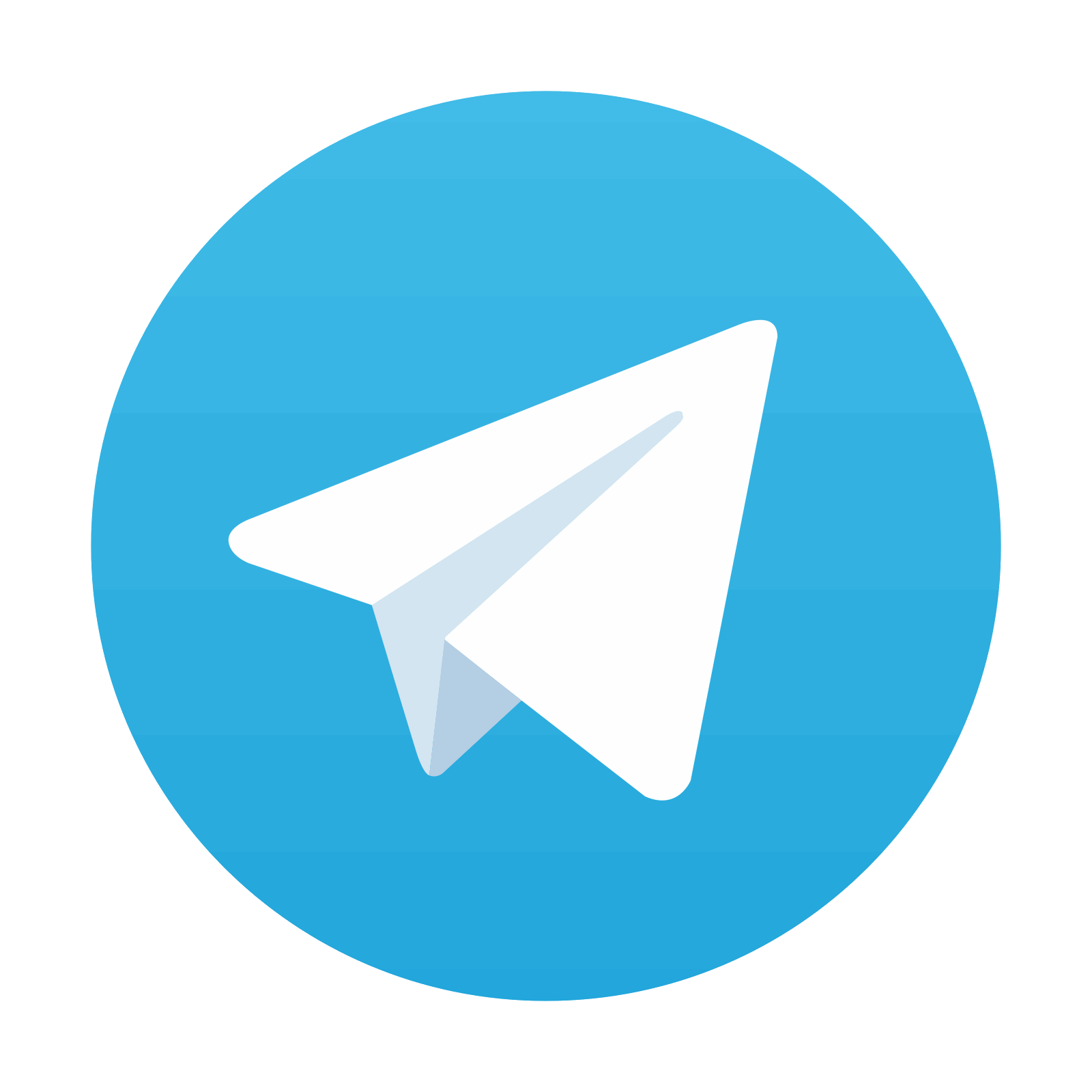
Stay updated, free articles. Join our Telegram channel

Full access? Get Clinical Tree
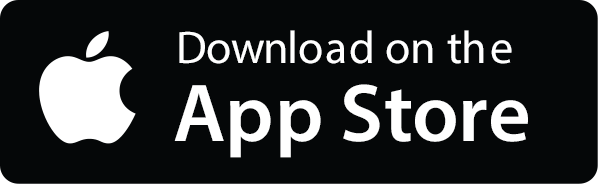
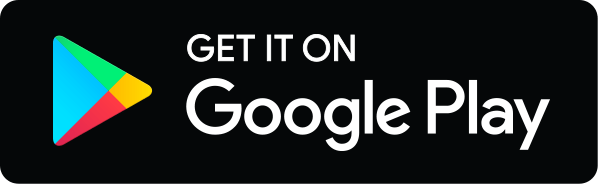
