Pediatric Foot and Ankle Fractures
Edwin J. Harris
Management of pediatric skeletal injuries requires awareness of the unique anatomy and physiology of the immature skeleton and recognition that children are more than just small adults. There are a number of anatomical and physiologic differences between pediatric and adult bone that directly influence fractures. Pediatric bone is more porous (1), has a higher water and osteoid content, and has a greater number of vascular channels. The result is more pliability and greater likelihood of plastic deformation without breaking. The periosteum is thicker but less adherent to the cortex than its adult counterpart. Although it strips away more easily from the cortex, periosteal thickness helps prevent displacement of fracture fragments. The presence of epiphyseal plates is unique to the child. Injury carries the risk of loss of longitudinal growth with and without angular deformity.
A number of fracture principles hold true for children as well as adults. Closed fractures are best managed by closed reduction with or without percutaneous fixation as long as satisfactory alignment can be achieved. Open fractures are treated by wound débridement, irrigation, stabilization, and appropriate soft tissue and skin coverage. Special attention must be paid to the integrity of articular surfaces. Childhood is a difficult time in a patient’s life to guarantee compliance with instructions to remain non-weight-bearing. For this reason, casting (both weight-bearing as well as non-weight-bearing) is appropriate in infants, children, and teenagers.
With these principles in mind, the discussion begins with the anatomic considerations involved in injuries to the physis and then proceeds to the diagnosis and treatment of other fracture patterns by anatomical site.
FUNCTIONAL ANATOMY
Long and short tubular bones are divided into the diaphysis (site of the primary growth center), the metaphyses (sites of active bone production by endochondral ossification), the physis (growth plate), and the epiphysis or apophysis (secondary growth center developing through endochondral ossification). The periphery of the diaphysis is covered by periosteum. The diaphyseal cortex develops through the process of intermembranous ossification. The metaphyseal cortex is composed largely of woven bone that has greater porosity and is thinner than that of the diaphysis. It offers very little support and is more susceptible to compression and bending failure. Epiphyses form articular surfaces. Apophyses serve as insertion of tendon, areas of weight-bearing or both. Epiphyses and apophyses share a common physiology. The differences between epiphyses and apophyses are those of function and minor alterations in microanatomy. The growth plates of epiphyses and apophyses are histologically and physiologically similar. Apophyses possess less proliferating cartilage and more fibrocollagen to help resist the tension forces associated with muscle contraction. When physiologic forces are exceeded, avulsion fracture of an apophysis by tension is more likely to occur before rupture of a tendon (2).
The epiphyseal plate or physis is a radiolucent structure located between the metaphysis and the epiphysis. It provides continuous linear growth of bone. Damage to the physis can result in some form of growth arrest. The physis is divided into a number of zones (Fig. 111.1). The area adjacent to the epiphysis is the zone of growth made up of resting or reserve chondrocytes and a layer of dividing cells directed toward the metaphysis. The resting chondrocytes had been regarded as the cells necessary for continued growth of the physis. However, it is now believed that the resting cells rarely replicate. Each has a large endoplasmic reticulum, suggesting a high level of protein synthesis in this area. They are probably responsible for providing the nutrients and materials required for the areas involved with active growth (3). There is also an abundant intercellular matrix containing chondroitin sulfate, hyaline, and collagen, which add considerable strength and nutritive support to this area (4,5). Increased mitosis occurs in the adjacent layer of actively dividing cells. These cells organize into palisades or longitudinal rows parallel to the long axis of the bone. In the zone of cartilage maturation, the chondrocytes are organized into columns and will hypertrophy. The previously rich intercellular matrix becomes sparse. At the same time, the random order of collagen fibrils within the intercellular matrix assumes an orderly arrangement between the cell columns (5,6). The hypertrophic chondrocytes have an active role in the calcification process (3). The relative decrease in intercellular matrix and intracellular substance in this region make this the weakest area of the physis. It is susceptible to shearing, bending, and tension stresses but more resistant to compression.
Once calcification has occurred, the cells enter the zone of cartilage transformation. When this area is sufficiently calcified, vascular mesenchyme invades the tissues, and the process of ossification and remodeling begins. Although this area is also relatively weak, the calcification process increases its strength compared with the zone of cartilage maturation. Yet, this area is regarded as the weakest part of the physis.
The circumferential groove surrounding the periphery of the physis is known as the zone of Ranvier. It is composed of fibroblasts, fibrocytes, undifferentiated mesenchymal tissue, and the osseous ring of Lacroix (an extension of the metaphyseal cortex). This groove is most noticeable in the younger patient and gradually disappears as the child matures. Its functions are to support the physis at the weak chondro-osseous junction and to provide for appositional expansion at its periphery so that transverse and longitudinal growth of the physis can proceed in harmony. At the same time, the zone restrains peripheral physeal growth (7). The periosteum of the metaphysis adheres firmly to the zone of Ranvier to bind the epiphyseal complex and the metaphysis together.
The periosteum of the child is more highly vascular, more osteogenic, thicker, and stronger than in the adult. The periosteum is loosely attached at the diaphyseal level and separated from the cortex by a layer of soft osteogenic tissue. The development of Sharpey fibers does not occur until the child approaches maturity. At this time, the periosteum becomes firmly attached to the diaphyseal cortex. The periosteum is much more active in osteogenesis in the diaphysis of the child (8). Separation of the periosteum from the cortex results in periosteal new bone formation. The musculature derives most of its origin from the periosteum rather than from the cortex. This allows coordinated growth of osseous and muscular tissues that otherwise would not be possible. The periosteum is very adherent at the metaphyseal cortex. The periosteum adds considerable strength against shearing forces at the junction of the epiphysis and metaphysis, minimizing displacement of fracture fragments. The ligaments surrounding the articulations insert into the perichondrium of the epiphysis, as well as into the zone of Ranvier. This allows for coordinated growth of osseous and soft tissues and also reduces the tensile forces on the epiphyseal complex.
The nutrient vessels pierce the cortex and supply the medullary canal and the cancellous metaphysis. The epiphysis has its own set of nutrient vessels. The physis remains a barrier to the vessels on both sides until the plate closes physiologically or is violated by trauma, infection, or other pathology.
PHYSEAL AND EPIPHYSEAL FRACTURES
Growth plate injuries are unique to the growing child. A working knowledge of the growth plate anatomy and physiology is essential to proper management of these injuries. This allows a working classification formulating prognosis and selecting appropriate treatment (9). These injuries are the result of shear, traction, avulsion, and compression forces (8). Salter concluded that the site of failure in physeal injuries was at the relatively weak area between the junction of calcification and cellular hypertrophy. Shear and avulsion forces were believed to be responsible for fracture of the physis (10). The resting and proliferating zones of the growth plate contain an abundant chondroitin sulfate matrix, and the intercellular substance helps provide resistance to shear (10,11). The zone of provisional calcification has a calcified cartilage matrix that provides resistance to shearing forces as well. Resistance to shearing is also derived from the undulated surface at the junction of the physis and metaphyseal bone (12). The area of cellular hypertrophy is the weakest area for this type of disruptive force (11). Collagen fibers run longitudinally in this area, but there is less collagen in the area of cellular hypertrophy than in other areas of the physis (10).
However, later studies have shown that the line of separation is more likely to violate several of the zones. The original concept of a single plane of failure is overly simplistic (8,9,13,14,15 and 16). Extension of the fracture lines into the zone of provisional calcification explains the appearance of lamellar flecks of bone from the metaphysis in pediatric injuries (13).
The mechanism of injury and patient age are the most important factors in determining the type of physeal injury that results. The mean age for most physeal fractures has been reported to be 10 to 12 years, with a range from 1 to 16 years. Given the fracture type and location, females are typically 1.5 years younger than their male counterparts (17). The mechanism of injury rather than patient gender or age may be the most important factor in determining the type of physeal injury.
Classification of Epiphyseal Plate Fractures
The most widely accepted classification remains that of Salter and Harris (5) as modified by Ogden. A number of additional injury patterns have been subsequently described to explain more fully additional fracture patterns and the associated prognosis (18,19). When all of these factors are considered, the end result is a rather cumbersome nine-part classification that ultimately includes not only injuries to the physis but also isolated injury to the epiphysis, the metaphysis, and the diaphyseal periosteum.
Type I
The type I fracture is a complete separation of the epiphysis from the metaphysis. The fracture line passes through the physis (Fig. 111.2). Although the line of separation through the physis has historically been thought to extend between the layers of cellular hypertrophy and degeneration, this is not always the case. All of the zones may be violated by an undulating line of cleavage (14,16). In some cases, small bony segments of primary and secondary metaphyseal bone may remain attached to the physeal segment (13). This is particularly true in children with preexisting metabolic and endocrine pathology. Type I injuries account for 6.0% to 8.5% of all physeal injuries and occur most commonly in the phalanges (20,21 and 22).
Type I injuries are more common in younger children. The greater thickness and immaturity of the physis are the two factors that predispose children to this injury. This pattern can occur before or after endochondral ossification of the epiphysis begins. These are common birth injuries and may also occur in nonaccidental trauma inflicted on the infant and young child. They are also seen in patients with endocrinopathies, rickets, and osteomyelitis (10,23). There is little displacement at the fracture site because of the firm periosteal attachments. If the epiphysis has ossified, these injuries may be appreciated on an x-ray. If the epiphysis has not yet ossified, the diagnosis can be very challenging. The prognosis is good unless the epiphysis is completely covered with cartilage. In this case, the blood supply to the epiphysis, which is dependent on the blood vessels that gain access through the perichondral ring area, may be significantly damaged.
In most cases, there is spontaneous reduction of the fracture because the periosteum remains intact on the concave side of the injury and maintains reduction while preventing overreduction in the opposite direction. Radiographs taken at the time of the injury are frequently interpreted as normal (Fig. 111.3A). The growth plate may appear wider than normal (Fig. 111.3B) or there may be a slight interruption in the continuity of the bone as the cortex is followed from the metaphysis to the epiphysis (Fig. 111.3A). In other cases, there may be some obvious misalignment of the epiphysis on the metaphysis (Fig. 111.3C). Displacement may be sufficient to necessitate manipulative reduction. This pattern is classified as type IA.
Type IB fractures occur in children with underlying pathology interfering with normal endochondral ossification at the metaphysis (Fig. 111.4). They are more likely to occur in children with myeloproliferative disorders such as leukemia and thalassemia, as well as in children with insensate limbs (18,19,24). The
plane of cleavage is primarily through the layer of degenerative cartilage cells or through the primary spongiosa (Fig. 111.5).
plane of cleavage is primarily through the layer of degenerative cartilage cells or through the primary spongiosa (Fig. 111.5).
![]() Figure 111.3 A: The width of the physis and alignment appear near normal. B: The physis is abnormally wide, indicating separation. C: The distal tibial epiphysis is offset anteriorly. |
In both type IA and IB fractures, the primary germinal elements are undisturbed; once healing is complete, normal growth resumes with no loss of length or angular deformity.
Type IC is a fracture with associated injury to a portion of the germinal cells of the physis (Fig. 111.6). This is a crush injury to all cell layers in the affected portion of the growth plate and occurs when the force propagating through the limb abruptly shifts. The result is osseous bridging at the point of physeal injury.
The treatment goals in type I injuries is to reduce any displacement if spontaneous reduction does not occur and to protect the physis from additional injury. This usually requires cast immobilization with or without weight-bearing.
Type II
Type II fractures are the most common growth plate injuries accounting for 73% to 75% of all reported physeal injuries (22,25). The mechanisms of injury are shear and avulsion. As the child matures, two things happen that make a type II injury more likely than type I injury. First, the relatively flat subchondral bone plate between the epiphysis and the physis will begin to contour in response to the biomechanical stresses acting on the joint surface. Second, cartilaginous extensions from the physis, known as mammillary processes, extend into the metaphysis. These two processes increase the stability of the physis against shearing forces and redirect disruptive stress on the physis into metaphyseal bone. In a type II fracture, the plane of cleavage enters the physis and propagates along the growth plate until it is redirected into metaphyseal bone (Fig. 111.7). A portion of the metaphysis is avulsed with the epiphysis. This fragment is known as the Thurston-Holland sign (Fig. 111.8). The periosteum remains intact on the concave side of the deformity that holds the metaphyseal fragment and assists in obtaining and maintaining reduction. Spontaneous reduction frequently occurs. The fracture may be difficult to identify if the metaphyseal fragment is small. This small metaphyseal fragment is called the Werenskiold sign and may be the only visible evidence of the injury (Fig. 111.9). As with type I injuries, the germinal cells of the physis remain attached to the epiphysis and are not damaged. This is a type IIA injury.
In type IIB fractures, there is a metaphyseal fragment that remains in continuity with the epiphysis, while the remainder of the metaphysis is fractured into two or more parts (Fig. 111.10). This comminution makes the fracture unstable and difficult to reduce. Often, the comminution is not appreciated until consolidation of the fracture (Fig. 111.11).
The type IIC fracture has thin layer of metaphyseal bone across the entire epiphyseal fragment (Fig. 111.12). The plane of cleavage is through the primary spongiosa of the metaphysis. This is more likely to occur in the phalanges because the trabeculae within these small bones are oriented in a transverse direction instead of longitudinally (Fig. 111.13).
The type IID injuries have compression against the physis, usually at the point where the force turns to exit through the metaphysis (Fig. 111.14). This damages all layers of the physis and a partial or complete growth arrest may result (Fig. 111.15).
![]() Figure 111.8 The large posterior metaphyseal fracture (Thurston-Holland sign) in this Salter-Harris IIA fracture. |
The treatment goals in type II injuries are to reduce any displacement if spontaneous reduction does not occur, to fixate the Thurston-Holland fragment if needed, and to protect the physis from additional injury. This usually requires cast immobilization with or without weight-bearing. Open reduction with internal fixation is often needed.
Type III
Type III fractures are complicated by disruption of the articular surface. The fracture line begins at the joint surface of the epiphysis and progresses through the secondary ossification center until it reaches the physis. There, it changes direction 90 degrees and extend through the physis to the periphery. Type III fractures generally occur in older children whose physes are nearing closure, although the injury may also occur before endochondral ossification of the epiphysis has begun. In this case, the epiphyseal damage may be underestimated. This injury pattern is typically the result of intra-articular shear. The simplest is the type IIIA configuration (Fig. 111.16). There may be considerable separation requiring open or closed reduction (Fig. 111.17).
Type IIIB differs from type IIIA because a small layer of primary spongiosa is avulsed with the epiphysis (Fig. 111.18).
Other than the few growth cells that are cleaved by the fracture line, the germinal layer of the physis is intact. However, there may also be damage to the epiphyseal blood vessels that could lead to ischemic necrosis of the epiphyseal fragment
with chondronecrosis of the germinal cell layer attached to the epiphyseal segment. Osseous bars can result from ischemia on the epiphyseal side of the plate (26). Incongruity of the joint surface is also a concern with this injury pattern.
with chondronecrosis of the germinal cell layer attached to the epiphyseal segment. Osseous bars can result from ischemia on the epiphyseal side of the plate (26). Incongruity of the joint surface is also a concern with this injury pattern.
![]() Figure 111.11 Repair of damaged metaphyseal trabecular shows the extent of the comminution of the metaphysis. |
![]() Figure 111.13 A fracture of the base of the fifth proximal phalanx. Note the broad expanse of metaphyseal bone. |
![]() Figure 111.17 Salter-Harris IIIA with displacement requiring reduction to preserve the articular surface. |
The treatment goals in type III injuries are to restore the anatomy of the physis and restore the articular surface. Open reduction and internal fixation are almost always necessary.
Type IV
Type IV fracture planes cross the joint surface, the epiphysis, the physis, and the metaphysis. There is often a rotation component to the injury, and the smaller fragment may displace in the direction of the diaphysis.
A type IVA injury occurs when the fracture line begins at the articular surface of the epiphysis, extends directly through it, and continues through all layers of the physis and into the metaphysis (Fig. 111.19). This lesion can occur before or after endochondral ossification of the epiphysis (Fig. 111.20). If the epiphyseal perimeter has not yet undergone endochondral ossification, the fracture of the epiphysis may not visualize on standard radiographs. There may be significant fragment displacement in two dimensions with this injury with rotation of the smaller fragment away from the larger segment in a direction dictated by the mechanism of the injury with displacement toward the diaphysis of the bone. This results in a step-off between the physeal margins of the two fragments and a misalignment of the articular surface. An osseous bridge may develop at the physeal step-off because of contact of cancellous bone to the physis. Because this injury is usually peripheral in the physis, the result is loss of length plus angular deformity. Additionally, the misalignment of the articular surface may lead to degenerative arthrosis.
In type IVB injuries, the fracture line also propagates horizontally through the remainder of the physis resulting in a three-part fracture that is made up of a peripheral fragment of epiphysis, physis, and diaphysis, as well as a second fragment that is made up of the remainder of the epiphysis. The third segment is the remainder of the metaphysis and diaphysis (Fig. 111.21).
Type IVC injuries are unique to the femoral neck and are not discussed here.
Type IVD occurs when there are multiple fragments of epiphysis, physis, and metaphysis in the same plane (Fig. 111.22).
The treatment goals in type IV fractures are prevention of physeal bridging and the preservation of the joint surfaces. Accurate anatomic reduction is necessary to meet both goals (10,27,29) or an osseous bridge will develop (Fig. 111.23).
Type V
Salter and Harris were the first to describe the type V injury in their classification system. This fracture was initially thought to occur after a severe crushing force that destroyed the physis and resulted in growth arrest (28) (Fig. 111.24). On initial examination, there is no radiographic evidence of injury. The diagnosis is made retrospectively as unmistakable signs of growth plate closure develop on follow-up x-rays (26,29,30). Growth disturbance may not become evident until several years after the injury.
Both the pathophysiology and the existence of Salter type V injuries are subjects of debate. The prevailing theory has always been that the injury consists of compression of the germinal zone without actually disrupting the growth plate as a whole (28). This concept has been challenged (10,31,32,33 and 34). More current theories regard the disruption of vascularity as the pathologic mechanism leading to premature closure. Some have theorized that most growth disturbances originate either from destruction of the epiphyseal circulation with death of the germinal cells from ischemia or from the development of continuity between the metaphyseal and epiphyseal circulations with osteogenic progenitor cells along the transphyseal vessel pathways resulting in bridge formation (12). Physeal closure occurs when the bridge exceeds a critical size. Below that critical size, the bridge fails to maintain itself because growth proceeds. This results in rupture of the bridge (32,34). On occasion, radiographs may suggest bridging. Follow-up radiographs show that these areas resolve when growth resumes (2). Severe damage to the epiphyseal vessels results in avascular necrosis of the epiphysis and can lead to premature plate closure. Others (33) have proposed that the premature closure is a by-product of prolonged immobilization, which may create a localized ischemia as opposed to damage to the germinal cells at the time of trauma. Mechanism of injury has a high correlation with premature plate closure. Residual displacement after reduction and the number of attempts at reduction may also influence premature closure (35).
![]() Figure 111.23 A Salter-Harris IVA fracture of the distal medial tibia. The physis and articular surface are anatomically reduced (arrows). |
There are a number of very specific criteria for the diagnosis of a type V fracture. There must be an appropriate history of injury. The injury should apply force in a nonphysiologic direction against an articulation that has only one plane of motion. The initial x-rays should appear normal. There should not be any treatment that in itself could produce growth arrest, and the physis must subsequently develop premature closure (36).
There is no effective treatment to minimize the likelihood of closure in type V injuries. The fate of the plate is sealed at the moment of injury. Since there is no sign of closure early on, all injuries to the physis should be considered to be type V, and the parents should be warned of the possibility of premature plate closure with loss of length and angular deformity as possible adverse outcomes.
Type VI
Type VI was not one of the original fracture types described by Salter and Harris but a pattern later described by Rang (37). It is an injury to the zone of Ranvier and the periphery of a subcutaneous physis (Fig. 111.25). Certain types of trauma, such as burns, contusions and degloving injuries, damage the peripheral areas of the physis and lead to partial growth arrest. In the ensuing years after Rang’s description, a number of cases resulting from sports injuries have been described (38). Initial radiographs may not show any osseous changes, although the damage to the perichondral ring may be seen as loss of cancellous bone from the adjacent metaphysis and epiphysis. Later, an osseous bar or bridge will develop peripherally where the perichondral ring was disrupted. This may result in angular deformity. Periosteum replaces the perichondrium at the site of the peripheral bar (39).
![]() Figure 111.24 Crush injury with displacement of the epiphysis of the second metatarsal A went to premature plate closure. Note the shortness of the metatarsal B. |
Treatment is directed to early excision of the peripheral bridge to prevent angular deformity.
Type VII
The type VII injury represents a fracture of the epiphysis that does not involve the growth plate. Because the physis is not involved, this is the only true epiphyseal fracture (13). However, if the injury involves the articular surface, then restoration of the fragment into anatomical alignment will reduce the likelihood of later joint arthrosis (Fig. 111.26).
This injury pattern may occur before the epiphysis ossifies. When this happens, it is virtually invisible on standard radiographic images.
Type VIII
Type VIII is a metaphyseal injury that does not involve the epiphysis or the physis (Fig. 111.27). Isolated metaphyseal fractures may be very difficult to identify. If the metaphyseal cortex is damaged, the fracture is easy to image.
A buckle of the metaphyseal cortex may be the only initial finding. As healing progresses, the cancellous fracture becomes obvious as new bone is laid down on the damaged trabeculae.
A buckle of the metaphyseal cortex may be the only initial finding. As healing progresses, the cancellous fracture becomes obvious as new bone is laid down on the damaged trabeculae.
The primary concern of this fracture type is the effect on the metaphyseal circulation. When the vascularity to this area is compromised, there is a temporary disruption in the formation of primary spongiosa. As a result of the compromise in osteoid mineralization, there will appear to be an apparent widening of the growth plate but without interference in linear growth.
Type IX
Type IX fractures involve significant periosteal damage that interferes with the formation of appositional bone derived from the periosteum (Fig. 111.28). This process can lead to bizarre patterns of periosteal new bone formation. If it occurs in the interval between adjacent bones, the result may be a synostosis. Periosteal stripping interferes with appositional bone growth in the metaphysis and diaphysis (13).
OTHER FRACTURE PATTERNS
PLASTIC DEFORMATION (BOWING)
Plastic deformation results from longitudinal force applied to an immature long bone. The cortex is compressed and bent until it reaches a point where it cannot recover its original shape when the force is released because the limit of elastic deformation has been exceeded. Other than the appearance of a bow in the bone, there are no other unique radiographic changes (Fig. 111.29). This condition is difficult to diagnose and difficult to reduce (40).
GREENSTICK FRACTURE
The greenstick fracture is a common pediatric injury occurring when a bending force is applied to the bone. The forces are concentrated in the diaphysis resulting in an incomplete fracture. Compressive forces on the concave side of the injury result in bending of the bone but without complete failure so that the cortex remains intact. There is a fracture on the convex or tension side of the bone (41) (Fig. 111.30). Although these fractures tend to be stable, they are difficult to reduce. Should the fracture result in significant deformity, then it may be necessary to complete the fracture to achieve reduction.
TORUS FRACTURE
Torus fractures are common injuries that occur at the transitional area from the more porous metaphyseal cortex to the dense diaphyseal bone (42). It is caused by the application of a longitudinal force applied to a tubular bone. The cortex buckles on one side of the bone with microfractures, and the opposite cortex appears intact. The metaphysis may be comminuted (Fig. 111.31). Torus fractures generally heal well with non-weight-bearing cast immobilization. Angular deviation may remodel, and later surgical intervention may be considered for residual deformity.
![]() Figure 111.29 A: Trauma results in deformation without obvious fracture. B: As healing progresses, the fracture becomes visible. |
CANCELLOUS FRACTURE
Cancellous fractures occur when the immature bone is subjected to compressive forces that crush the trabeculae. They can occur in the diaphyseal and metaphyseal areas of long and short tubular bones but are more common in the small bones of the foot that develop without epiphyses. Cancellous fractures are more likely to develop while there is still a substantial rim of unossified cartilage surrounding the ossification center. The diagnosis may prove difficult because there is no break in the peripheral contour of the bone. Initial radiographs are normal (43) (Fig. 111.32A). After a period of 10 to 20 days, radiographs will show new bone laid down on damaged trabeculae (Fig. 111.32B). This repair process is quickly completed so that standard radiographs made 4 to 6 weeks after injury reveal an almost normal appearance to the bone.
Fractures with potential instability must be treated by immobilization. Healing time for all forms of cancellous fracture is 4 to 6 weeks.
PATHOLOGIC FRACTURES
Pathologic fractures may occur through any preexisting benign or malignant lesion in the child’s bone. Any lesion can be a risk factor for pathologic fracture. These are rare because there are few lesions that develop in the foot and ankle at this age that will sufficiently weaken the bone to allow fracture. Notable exceptions to the rarity include enchondromas, metatarsal and calcaneal unicameral bone cysts, and nonossifying fibroma of the tibia (Fig. 111.33). Stress fractures are not considered pathologic fractures, but it is possible for a stress fracture to convert into a complete fracture in the child. By definition, this becomes a pathologic fracture through a preexisting lesion. Pathologic fracture can also occur in association with metabolic diseases such as renal osteodystrophy, primary and secondary hyperparathyroidism, and scurvy. They may also occur following radiation therapy in children (13).
OVERUSE
This symptomatic condition is considered an overuse or stress injury to the physis (13). The condition has been noted in adolescent athletes in whom greater degrees of stress are exerted on an extremity. These injuries primarily involve the distal radius
and ulna, but they have been noted in the distal fibular physis as well (13). Patients describe pain localized to the physis that is greater with activity or stress to the area and is improved with rest. Radiographs demonstrate widening and irregularity of the affected growth plate with sclerosis of the metaphysis. The epiphysis is not displaced. Contralateral films may be required to help establish the diagnosis. Treatment consists of rest and a reduction in activity.
and ulna, but they have been noted in the distal fibular physis as well (13). Patients describe pain localized to the physis that is greater with activity or stress to the area and is improved with rest. Radiographs demonstrate widening and irregularity of the affected growth plate with sclerosis of the metaphysis. The epiphysis is not displaced. Contralateral films may be required to help establish the diagnosis. Treatment consists of rest and a reduction in activity.
STRESS FRACTURES
Because of the increased number of adolescents engaged in recreational and competitive sports, the incidence of true stress or fatigue fractures has also increased (43). The tibia and lesser metatarsals (Fig. 111.34) are frequent sites of involvement. The calcaneus is also a common location (44). Stress fractures of the calcaneus and other tarsal bones are not
to be confused with cancellous fractures because cancellous fractures have a clear history of a single incident of trauma. Ozonoff defined two types of stress fractures in the pediatric age group. The first is an incomplete fracture resulting in damage to only one cortex. The second is local sclerosis developing as the result of reaction to microfractures that are not grossly visible.
to be confused with cancellous fractures because cancellous fractures have a clear history of a single incident of trauma. Ozonoff defined two types of stress fractures in the pediatric age group. The first is an incomplete fracture resulting in damage to only one cortex. The second is local sclerosis developing as the result of reaction to microfractures that are not grossly visible.
![]() Figure 111.34 A: Early stress fracture in a 10-year-old male with equinus deformity. B: Stress fracture in another child healed with exuberant callus indicating fracture movement. |
Metatarsal stress fractures can be managed in surgical shoes if the patient is cooperative and will suspend sports and other activities for the duration of healing. There is a possibility that a stress fracture could convert to a pathologic fracture. If there is any concern about curtailing activities, a walking boot or a weight-bearing cast is in order.
THE CLINICAL APPROACH TO PEDIATRIC FRACTURES
GENERAL PRINCIPLES OF DIAGNOSIS AND TREATMENT
The previous discussion has outlined the various types of pediatric fractures, and this knowledge is critical to appropriate management. However, fractures in infants and children can be very difficult to diagnose for a number of reasons. Children are very active. Running, jumping, falls, and other activities are a part of the child’s routine. Even minor trauma can produce fractures of the foot and ankle in infants and small children. Additionally, the trauma is frequently not witnessed or not appreciated by an adult or an older responsible child. The very young are often not verbal enough to provide the necessary history of injury and may have difficulty localizing their pain. They may tend to perceive pain more distal to the site of the actual pathology. Injuries may occur while the child is engaged in some forbidden activity. Details of the injury or accident may be less than accurate out of fear of punishment. Even in the emergency room staffed by experienced physicians who are challenged routinely by pediatric patients (45), fractures can be missed, or normal anatomical variants may be misdiagnosed as fractures (46). Metatarsal and phalangeal fractures are the most commonly missed fractures in the emergency rooms (47).
Additional challenges encountered in managing pediatric foot and ankle injuries include the identification of those injuries that require operative treatment as well as the recognition and management of comorbidities such as compartment syndrome, complex regional pain syndrome (48), and avascular necrosis (46,49,50,51 and 52).
It is necessary to maintain a high index of suspicion for potential fracture when injuries occur in young children (53). Growth plate fractures and buckle fractures are the pediatric counterpart of adult sprains (9,54). The tensile strength of the ligaments is much greater than that of the growth plates and bone (8,43,54,55). Although damage to collateral ligaments can occur at any age group (Fig. 111.35), it remains the exception. A fracture should be suspected before a ligament injury (10,29,41,43). Pain localized to the area of a physis is pathognomonic for fracture of the growth plate (9,56). The symptoms at the time of presentation are variable, but pain and swelling are usually localized to the physeal area. Clinical findings may be minimal and easily overlooked. Findings include limping, edema, erythema, ecchymosis, deformity, local pain, and point tenderness. Ecchymosis develops after a few days, but some children never develop this finding. Because displacement is usually minimal, obvious deformity is rarely a helpful finding. “Goose-egg” edema resulting from hematoma is often seen if epiphyseal separation has occurred (Fig. 111.36). These clinical signs can resolve completely over several days. Poorly localized subjective pain may be all that remains after 48 hours.
Gait disturbance is another common presenting symptom in children with fractures of the foot and ankle. The antalgic gait pattern is characterized by shortened stance phase, early heel-off, and knee extension throughout stance on the side of
the injured extremity. The ultimate gait disturbance is refusal to walk. Because refusal to walk is a regression of an established developmental landmark, it should always be taken seriously. Trauma is the most common cause for any of these gait disturbances (57).
the injured extremity. The ultimate gait disturbance is refusal to walk. Because refusal to walk is a regression of an established developmental landmark, it should always be taken seriously. Trauma is the most common cause for any of these gait disturbances (57).
![]() Figure 111.35 A 7-year-old female with an ankle inversion injury produced anterior talar tilt (A) and an anterior drawer sign (B) instead of a distal fibular physeal fracture. |
Some injuries are devastating but totally preventable. Lawnmower-associated trauma produces serious and disabling injuries (Fig. 111.37). In addition to the fractures that result, complicated wounds and major loss of bone result in loss of function and need for a multidisciplinary approach that includes the foot and ankle surgeon, plastic surgery, orthotics and prosthetics, and rehabilitative medicine (58,59).
There is a common misconception among health care workers that infants and small children have high tolerance for pain and do not need analgesics. This is untrue, and they need analgesics for at least 48 hours to remain comfortable, obtain sleep, and reduce anxiety. Mainstay analgesics include acetaminophen (with or without codeine) and ibuprofen (60).
DIAGNOSTIC IMAGING
RADIOGRAPHS
Plain radiographs, even though they have limitations, are the primary imaging modalities for evaluating fractures in children. Clinical evaluation helps identify the likely site of the injury and guides the selection of the radiographic views. In general, at least three views of the area should be obtained. It is very helpful to have two views at right angles to each other. One joint level proximal and one distal to the injury should be included in the study (61,62). Additional views are obtained as required. Contralateral films for comparison may occasionally be helpful but should not routinely be obtained.
The diagnosis of physeal injury with standard radiographs depends on changes in the physical characteristics of the growth plate. Epiphyseal displacement is pathognomonic for growth plate fracture (Fig. 111.38A). Angulation may cause obliteration or poor definition of the margins of the epiphysis and metaphysis. Spontaneous reduction of displaced epiphyses is a common event (10). If spontaneous reduction occurs, the physis may appear wider than normal (Fig. 111.38B). If the clinical circumstances support the diagnosis of a physeal injury, then treatment should be started despite negative radiographic findings.
Radiographic signs of periosteal new bone and repair of metaphyseal microfractures may be seen about 10 days after the injury (10) (Fig. 111.39A). Cancellous fractures do not show x-ray changes until 10 to 14 days after the incident (Fig. 111.39B). The diagnosis can be made clinically if there is focal tenderness over the involved bone.
ADDITIONAL IMAGING MODALITIES
Bone scans may increase the diagnostic yield in patients with occult fracture (63) and can help localize the pathology before there are changes on plain radiographs (Fig. 111.40). Bone scans are not required if there is a clear history of trauma and clinical support for a diagnosis of a fracture. The radiation involved precludes routine use. The best indication for this modality is an attempt to establish the diagnosis of occult fracture when the cause or location of the pain is uncertain. Radionuclide scans may also be used for skeletal survey in suspected child abuse. Uptake patterns may help localize the source of the pathology and provide clues to the pathophysiology of the process. Intense uptake means increased blood flow and bone turnover. Photon deficiency resulting from decreased blood supply or lack of osteoid mineralization suggests osteonecrosis. Reduced uptake can also be indicative of a physeal bridge as a complication of growth plate injuries (64).
The rearfoot and ankle may be difficult to visualize on plain radiographs because of the anatomical complexity. The anatomy is complicated, and the fracture patterns may be complex. Computed tomography (CT) may provide valuable information for diagnosis and treatment, help evaluate the articular surfaces, help identify physeal bridges, and aid in surgical planning (13,65).
Magnetic resonance imaging (MRI) studies may also be employed and may be preferred in patients with more complex
injury patterns owing to its ability to demonstrate the cartilage and soft tissues and to image in sagittal, coronal, or oblique long axis planes (13,66,67 and 68). The resolution and accuracy are not influenced by the problems of volume averaging and reformatting that are associated with CT. Furthermore, MRI may be preferred in the assessment of fibrous or bone bridging of the physis, growth arrest, avascular necrosis of the epiphysis, or infarction of the metaphysis (13). MRI may be particularly useful when the injured bone is surrounded by unossified cartilage and when the physis is involved (69).
injury patterns owing to its ability to demonstrate the cartilage and soft tissues and to image in sagittal, coronal, or oblique long axis planes (13,66,67 and 68). The resolution and accuracy are not influenced by the problems of volume averaging and reformatting that are associated with CT. Furthermore, MRI may be preferred in the assessment of fibrous or bone bridging of the physis, growth arrest, avascular necrosis of the epiphysis, or infarction of the metaphysis (13). MRI may be particularly useful when the injured bone is surrounded by unossified cartilage and when the physis is involved (69).
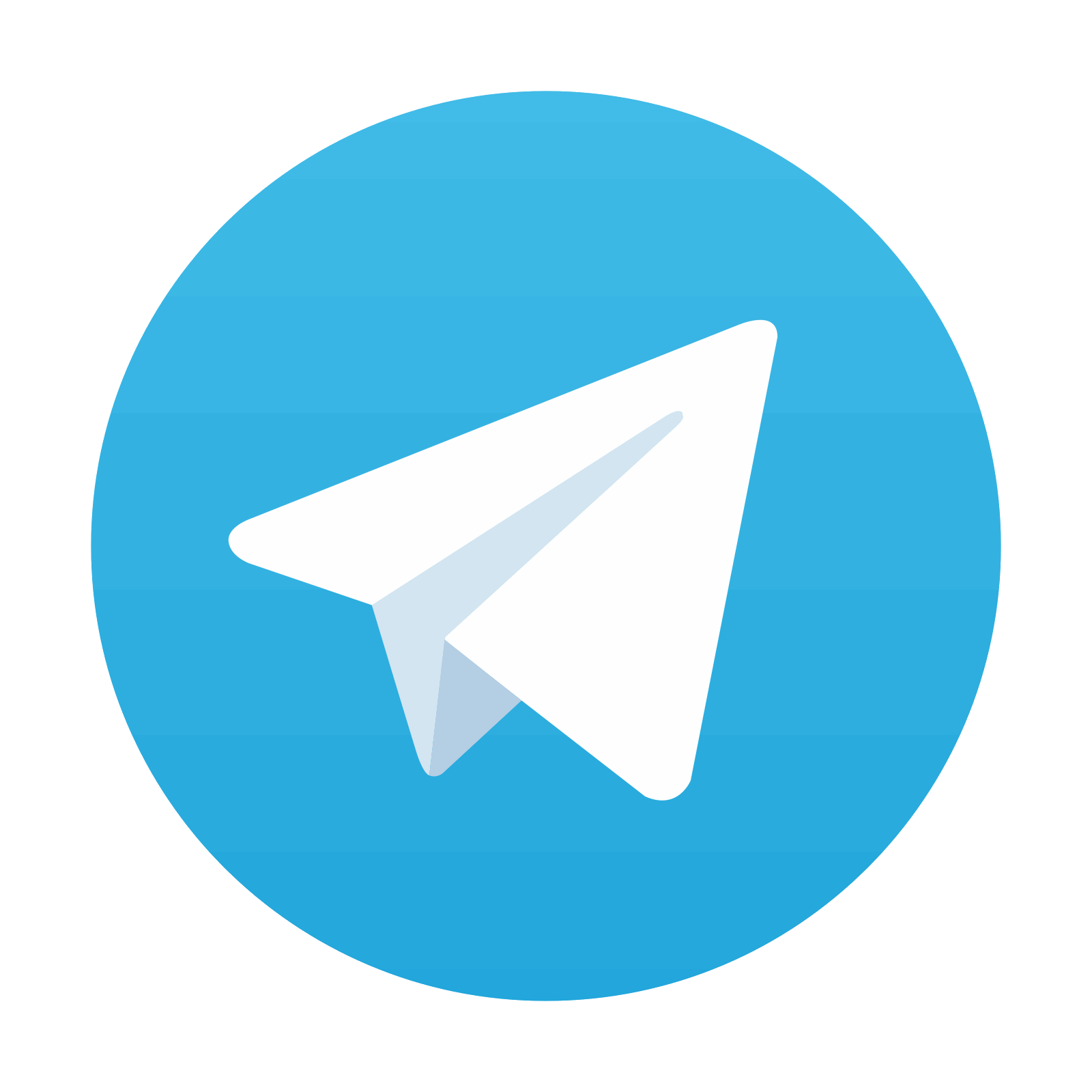
Stay updated, free articles. Join our Telegram channel

Full access? Get Clinical Tree
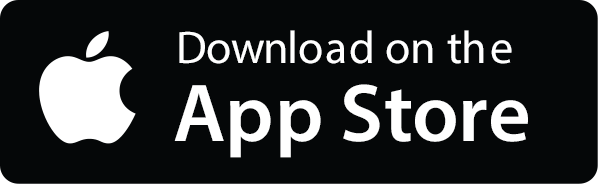
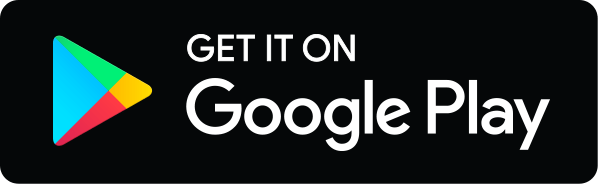