CHAPTER 23 Pathology and biomechanics of posterior instability

Introduction
Posterior instability is relatively rare.1–4 Therefore, our experience and understanding of this pathology is relatively limited. Furthermore, posterior instability has many different description categories in terms of volition (e.g., voluntary positional, voluntary muscular, involuntary),2,5 anatomic location (e.g., bony humeral, bony glenoid, labrum, ligaments), and mechanism (e.g., traumatic, atraumatic, seizure).6 In the setting of traumatic injury, the shoulder is typically in an adducted, forward flexed, and internally rotated position, and the pathology is often unidirectional. Atraumatic overuse injury often manifests an inferior component to the instability. However, relatively few patients (23%) will report a specific episode of dislocation requiring relocation.4 Some patients will describe a significant trauma, however, without frank dislocation. Relatively more patients will report an insidious onset of symptoms, usually from a repetitive exertional activity (e.g., lineman blocking in the setting of American football7). Recurrent posterior subluxation is more common than dislocation, and the patient will often complain of instability, with or without pain.5 Notably, the surgeon’s ability to subluxate the shoulder is common regardless of diagnosis for many pathologies not necessarily related to instability—examination under anesthesia often reveals grade 2 laxity (humeral head spontaneously reduces from the glenoid rim), which emphasizes the importance of a careful history.8 Patients who voluntarily create instability should be assessed with caution.2
Arthroscopy has allowed the surgeon to inspect and address specific structures in a relatively refined manner. Posterior structures implicated in posterior shoulder instability include the glenoid, labrum, and inferior glenohumeral ligament. The “circle concept” of the shoulder suggests that posterior injury will be accompanied by anterior injury.9–13 The structures anteriorly that may be involved include the rotator interval (superior glenohumeral and coracohumeral ligaments), anterosuperior labrum and biceps anchor, and rotator cuff.14 The indications for when to address the anterior structures in the setting of posterior shoulder instability remain controversial.14–20
Anatomy and biomechanics
Key structures
The humeral head relationship to the glenoid has been likened to a golf ball on a tee and quantified using a “glenohumeral index,” defined as the maximum glenoid diameter divided by the maximum humeral head diameter.21 This relationship explains the relative mobility of the glenohumeral joint. Glenoid version and humeral retrotorsion can affect posterior instability. Ten to 15 degrees of additional glenoid retroversion has been described as an indication for bony procedures, for example, opening wedge osteotomy or bone-block glenoplasty (which are exceptionally rare).1,2,22 Traumatic reverse bony Bankart lesions and reverse Hill-Sachs lesions can create symptomatic instability as well.
Most cases of instability often involve the capsulolabral complex of the inferior glenohumeral ligament complex (IGHLC).15,17,19,20 The labrum deepens the socket by 50%, and provides congruity to the humeral head23—the bony glenoid alone is flat in relation to the ellipsoid humeral head. Pathoanatomy of the labrum in posterior instability can manifest as a concealed avulsion of the posteroinferior labrum (“Kim’s lesion”), which has obvious implications when assessing for technical approach to repair.24 Through the labrum, the inferior IGHLC attaches on the glenoid between the 7- to 9-o’clock and the 2- to 4-o’clock positions. The IGHLC has been described in at least two ways. Bigliani et al have described three regions of the IGHLC: superior band (anterior-most region), anterior axillary pouch, and posterior axillary pouch25,26 (Fig. 23-1). O’Brien et al have described thickenings or “bands” that delimit the IGHLC anteriorly and posteriorly.27 The collagen fibers of the IGHLC directly attach to the rigid fibrocartilaginous labrum and the periosteum along the glenoid neck more medially. Notably, the posterior “band” has been shown to be an inconsistent structure, identified in only 63% or less of specimens examined.26,28,29 Recognizing this fact would be important when addressing this structure surgically for posterior instability.
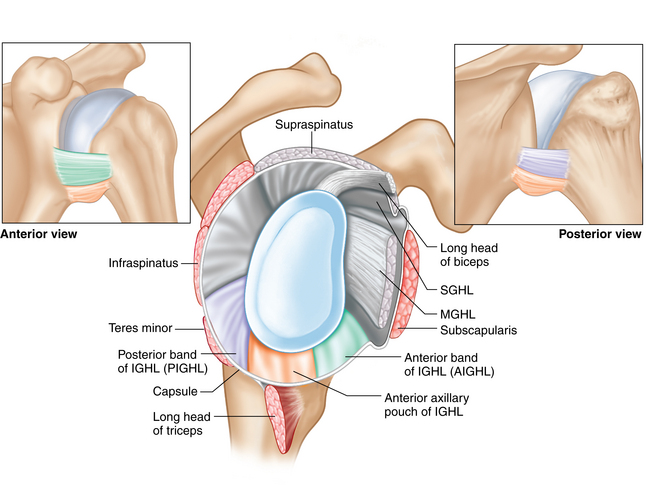
(Modified from Ticker et al: The inferior glenohumeral ligament: a correlative investigation. J Shoulder Elbow Surg 15(6):665–674, 2006.)
On the humeral side, the IGHLC attachment has been described in detail.27,30 Two attachment geometries have been described: (1) split-type (58%) and (2) broad-type (42%), both extending inferiorly 2 cm from the articular surface from approximately the 4- to 8-o’clock positions.31 The split-type manifests as a capsular bifurcation having internal and external folds with an interposed loose connective tissue; the broad-type does not have separate folds (Fig. 23-2). Failure to release the external fold may tether the capsular pouch in cases of posterior and anterior multidirectional instability. Releasing the entire capsule from the humeral attachment may be necessary to adequately perform a given posterior capsulorrhaphy shift procedure.
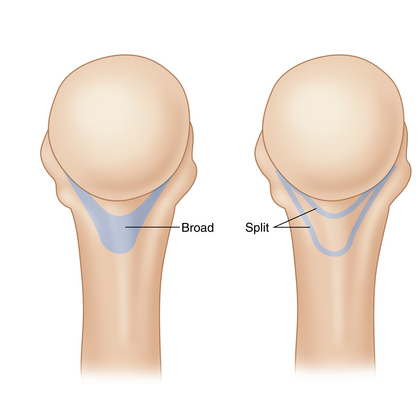
FIGURE 23-2 Broad- and split-type IGHLC humeral attachment sites.
(Modified from OKU 3 Shoulder and Elbow, Chapter 1, courtesy Maxwell C. Park, MD.)
The rotator interval (RI) is a triangular space with the glenohumeral joint capsule bordered by the coracoid at its base and the supraspinatus and subscapularis muscles converging to the apex.32,33 The RI also consists of the coracohumeral ligament (CHL) superficially, and the superior glenohumeral ligament (SGHL) deep.32,33 The long-head biceps tendon traverses this space from the supraglenoid tubercle intra-articularly, exiting the intertubercular groove distally (Fig. 23-3). Understanding the relationship between these structures may aid the surgeon when considering closure of the interval because the anatomy in this area can be variable and recognition of pathology may be challenging.14,34 Notably, the dimensions of the RI diminish with internal rotation.33 Imbricating the RI with the arm internally rotated may lead to overtightening with loss of external rotation. Therefore, it is recommended that RI closure be performed with the arm externally rotated.
Biomechanical function
Version of the glenoid and humerus,35 dynamic stability through a concavity compression effect,36–38 and intra-articular pressure39,40 play a role in joint stability. More commonly, soft tissue pathology involving the labroligamentous structures is encountered with posterior instability, which is relatively rare compared with anterior instability.4,15,19,20
The labrum has been shown to contribute 10% to 20% stability,36,38 contributing up to 50% of glenoid depth.23 The IGHLC attaches to the glenoid through the labrum. The tissue between the anterior and posterior capsular regions has been likened to a hammock, with the glenoid-labrum and humerus as posts, which “swings” or rotates with humeral rotation to provide static stability in a reciprocal load-sharing capacity; with abduction and external rotation (e.g., overhead throwing), the anterior complex with its band resists anterior translation, while abduction and internal rotation causes the posterior complex to resist posterior translation.27
The posterior capsule has been shown to have similar material properties compared with the anterior IGHLC region41; no differences in tissue modulus were detected, suggesting similar composition and fiber orientation between regions. Notably, the inferior portion of the posterior capsule had the thinnest dimensions, and the anterior region had higher failure strains.41 Proteoglycan content has been shown to be relatively less in the posterior region,26,42,43 which may indicate a lesser degree of viscoelastic behavior; how this relates to injury and healing after repair is yet to be fully elucidated.
Bigliani et al biomechanically tested IGHLC bone-ligament-bone segments at relatively low strain rates, providing evidence that permanent stretching of the capsule may explain instability after injury.25 Another study demonstrated nonrecoverable IGHLC strain as a result of cyclic loading, supporting the hypothesis that repetitive subfailure strains can lead to irreversible stretching of the IGHLC (e.g., overuse injury) and contribute to the development of acquired shoulder instability.44 Testing specimens with relatively higher strain rates has revealed that unrecoverable capsular elongation was largest in the ligament midsubstance and smallest at the humeral insertion45; the minimal absolute strain values suggest that only slight plication of the inferior capsulolabral structures may be necessary after a primary instability injury. The posterior capsule may behave similarly with respect to midsubstance injury,41 having implications when considering repair. Notably, 81% of all posterior capsule specimens failed near the glenoid insertion (100% of the inferior posterior capsule specimens failed in this region), consistent with the anatomy addressed clinically.15,19,20 Furthermore, recognizing that the posterior “band” has been shown to be an infrequent structure26,46 can be important when assessing pathologic anatomy during surgery and performing capsulorrhaphy (Fig. 23-4). Biomechanical studies have shown the ability to restore glenohumeral kinematics after posterior plication.18,47
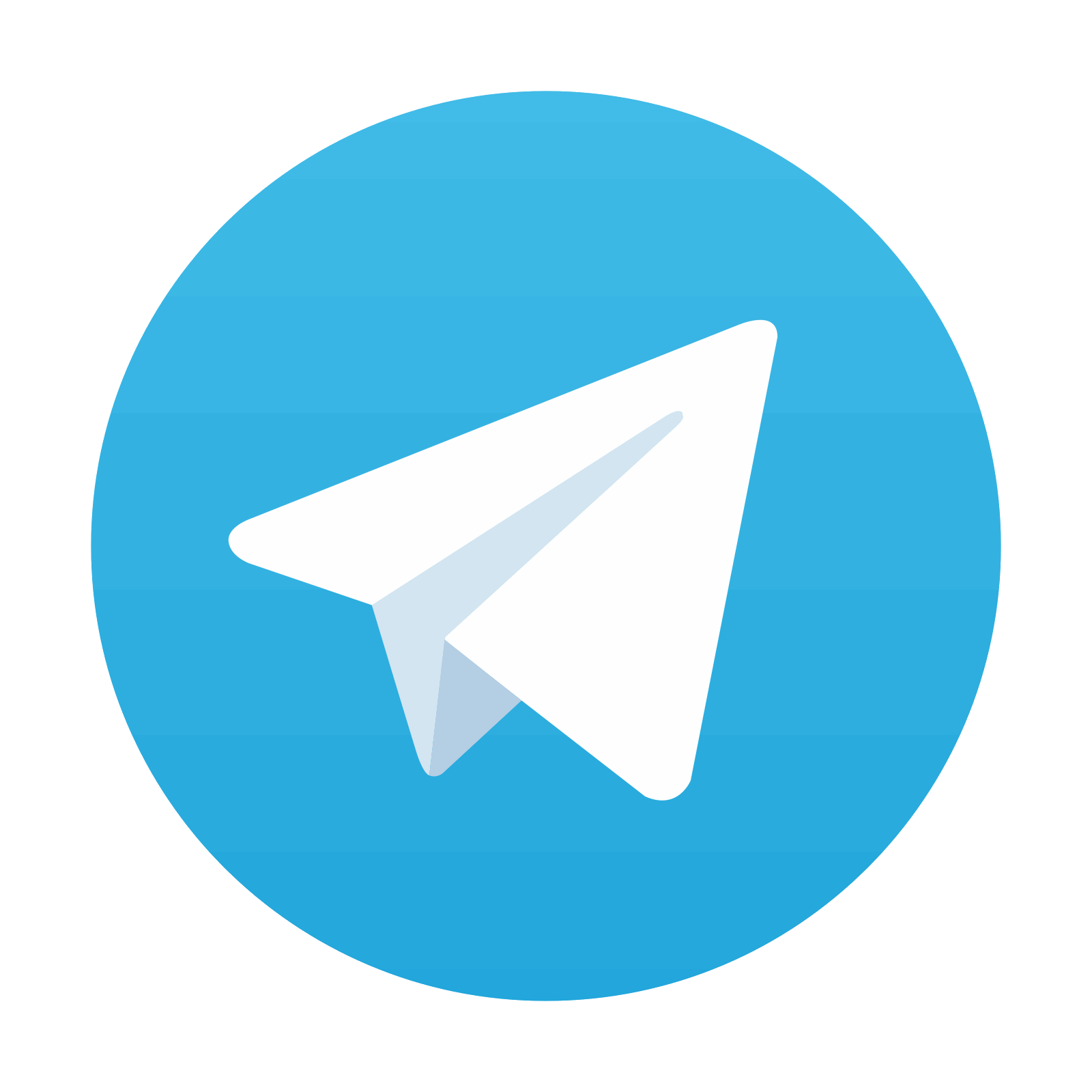
Stay updated, free articles. Join our Telegram channel

Full access? Get Clinical Tree
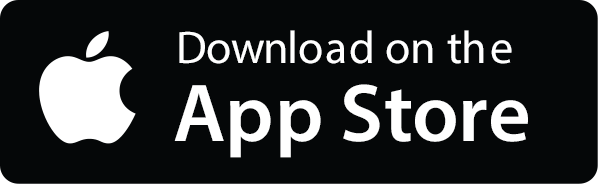
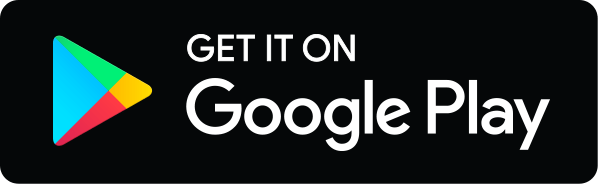