Chapter 1 Age-related bone loss and bone loss due to osteoporosis pose major problems for the orthopaedic fixation of bone not only because of diminished bone stock in which fixation devices must find anchorage, but also because future bone formation at the site may fail to solidify the construct or because future bone resorption may cause loosening and failure. The statistics on osteoporosis presented in the platform statements for the Bone and Joint Decade are sobering: Current estimates are that the number of individuals older than 50 years will double between 1990 and 2020, and that for the first time in Europe in 2010 there will be more people older than 60 years than people younger than 20 years.1 Osteoporosis affects 10 million Americans and 18 million more are at risk; 80% of those at risk are women. Each year, 1.3 million fractures (including 500,000 vertebral fractures) are attributed to osteoporosis. One half of all American women older than age 50 years are expected to sustain an osteoporosis-related fracture in their lifetime. One in every six white women will have a hip fracture, and the rate of hip fractures increases exponentially with increasing age. After age 65, rates for both men and women double in each decade of life. Two thirds of the individuals who have a hip fracture do not return to prefracture level of function. The objective of this chapter is to present a current perspective on the pathogenesis of osteoporosis and to relate traditional histomorphometric analyses of bone biopsies to current serum and urine biomarker assays of bone cell activities. Bone remodeling consists of two phases: bone resorption by osteoclasts followed by bone formation by osteoblasts. This process occurs continually and simultaneously at different sites throughout the skeleton. Bone matrix is produced by osteoblasts at sites of previous bone resorption (Howship’s lacunae; resorption cavities). Prior to this, an initial resorption stimulus signals and recruits osteoclast precursors to the site of bone resorption. Mature osteoclasts, rich in lysosomes with high tartrate-resistant acid phosphatase (TRAP) content, carry out the resorption process and leave behind empty resorption cavities (Howship’s lacunae). TRAP is a valuable enzyme marker used for the identification of osteoclasts.2 During remodeling, it is important to remember that although osteoclasts are not numerous in the iliac crests of postmenopausal osteoporotics, the individual cell activity of the osteoclast in removing bone matrix is much greater than the individual cell activity of the osteoblast in making new bone matrix.3 During resorption, signals recruit osteoblast precursors to the Howship’s lacunae. With the advent of mature osteoblasts, bone formation begins. Early osteoblasts are very active in depositing matrix and are plump cells with abundant alkaline phosphatase demonstrable in their cell membranes. These cells secrete procollagen, collagen fibrils are formed outside the cell, and collagen bundles are then laid down in an ordered arrangement at the bone surface. As a site of bone formation nears completion, osteoblasts become less active and appear more flattened and spindle-shaped; when formation has ceased, fibroblast-like cells line the quiescent bone surface. There are two important components of the remodeling cycle: (1) the depth to which osteoclasts “excavate” the resorption lacunae, and (2) the efficiency with which osteoblasts are able to fill in this excavated site. If it is perfectly filled in, the amount of bone formed equals the amount of bone lost, and there is bone balance. When the amount of bone resorbed exceeds the amount formed, however, net bone loss occurs. If the imbalance between formation and resorption is low and overall bone turnover is low, there will not be a big change in bone mass. If, however, there is an imbalance between formation and resorption, which favors resorption in the presence of high turnover (i.e., the presence of a large number of active remodeling sites throughout the skeleton, also termed a high activation frequency), large bone loss will result. Following its synthesis and secretion, bone matrix must be converted into mature osteoid prior to the initiation of mineralization. Although bone matrix maturation is an important but poorly understood process, two processes are reasonably well established: proteoglycan loss and formation of intermolecular cross-links. Newly secreted matrix components can be considered to be at 0% maturation, and matrix in which mineralization is being initiated can be considered to be fully matured. The histologic benchmark for the evaluation of mineralization and determination of the quality of mineralization is tetracycline incorporation at the mineralizing front. Tetracycline in vivo labeling techniques have made possible the quantitative histomorphometric determinations of bone formation and mineralization indices. Most tetracycline antibiotics form stable tetracycline-calcium chelates, which fluoresce intensely at wavelengths readily obtained with the standard fluorescence microscope. These chelates are formed only at sites of new bone deposition where the matrix contains 20% or less of the maximum mineral content.4 These chelates are locked into bone during mineralization and remain there until that bone site undergoes bone resorption. When two time-separated doses of tetracycline are administered prior to a bone biopsy, the distance between these two labels can be measured with histomorphometry, and a variety of quantitative indices determined based upon the portion of the bone surface lined by osteoid and that marked with the tetracycline label (Fig. 1–1A). Ducy et al5 have recently reviewed current information on the cell biology of the osteoblast. The osteoblast, which derives from mesenchymal stem cells, produces transcripts that distinguish it from the fibroblast cell: Cbfa1 controls the directional information for a differentiation factor for the osteoblast lineage by regulating the expression of osteocalcin, a gene expressed in terminally differentiated osteoblasts. Following embryogenesis, Cbfa1 expression is limited to osteoblasts (with some expression also occurring in hypertrophic chondrocytes). OSE1 is another regulatory element present in the osteocalcin promoter; it appears to be found in poorly differentiated osteoblasts. Osteocalcin is secreted by osteoblasts and appears to inhibit osteoblast function. During osteoblast differentiation, each of the major families of growth factors plays a role in osteoblast embryonic differentiation, and the growth factors themselves become bound into the bone matrix and may be subsequently released during later bone resorption at that site. FIGURE 1–1 Photomicrographs of (A) normal and (B) abnormal tetracycline incorporation. Clear separation of crisp, distinct labels is seen in the normal specimen. The patient received two courses of tetracycline prior to biopsy; the double labels show that this bone-forming site was active when the first (label 1) and second (label 2) courses of tetracycline were administered. In specimens from patients with osteomalacia, tetracycline labels are diffuse and smeared (B). (Undecalcified methyl methacrylate embedment, Fig. 1A, original magnification ×88; Fig. 1B, ×126.) (From Gruber and Singer,33 with permission.) Teitelbaum has recently summarized the current understanding of the cell biology of the osteoclast.2
PATHOGENESIS AND HISTO-
MORPHOLOGY OF OSTEOPOROSIS
BONE CELLS AND BONE REMODELING
NEW INSIGHTS INTO BONE REMODELING
Stay updated, free articles. Join our Telegram channel

Full access? Get Clinical Tree
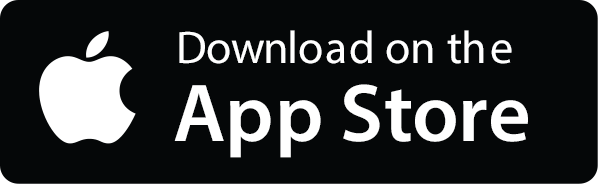
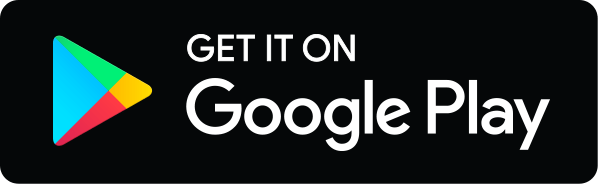