Chapter 4 The goal of this chapter is to explain the basic biomechanical principles of commonly used orthopaedic devices in the fixation of fractures, with focus on the bone-implant interface, since this is the specific part of the fixation system that is directly affected by osteoporotic bone. This chapter first briefly addresses specific mechanical changes in bone due to osteoporosis that affect its mechanical properties, to provide perspective when discussing the basic functions of fixation devices. Then, the conceptual mechanical principles of devices such as bone screws, plates, sliding screw-plate devices, intramedullary rods, external fixators, and spinal fixation devices will be presented. FIGURE 4–1 (Upper) Loss of integrity in osteoporotic vertebra and (lower) result of loss of compressive strength on screw toggle and loss of shear strength on resistance of screws to pullout. Osteoporosis has specific effects on the mechanical properties of trabecular bone. It decreases the diameter of the trabeculae and produces a loss of interconnecting struts.1 This loss of bone mass leads to a decrease in the ultimate stress at which bone will fail in compression2 and shear.3 Compressive strength is important in resisting the toggling of vertebral screws with vertical loading (Fig. 4–1). Bone shear strength is important to resisting screw pullout forces. The relationship between loss of bone mass and decrease in compressive or shear strength is not linear. In other words, a twofold decrease in density in bone due to osteoporosis will reduce the ultimate compressive stress by a factor of approximately four. This may be the most important effect when considering fixation in osteoporotic as opposed to normal bone. FIGURE 4–2 (Upper) Cross sections through femora with osteoporosis showing the change in geometry of the cross section; (lower) loss of bone cortex reduces the ability of the opposite cortex to buttress the fixation and decreases the holding power of screws because of reduced length of engagement. Osteoporosis also affects bone cortex dimensions, particularly in the femur (Fig. 4–2). The outside diameter of the diaphyseal cortex of a long bone increases, as does the inside diameter, but not at the same rate. The result is a significant thinning of the cortex. Since screw pullout strength is directly related to the length of engagement of the screw thread, loss of cortical thickness directly decreases screw holding power. Further, the ability of load to be transferred across the cortex opposite that on which the plate is placed (buttressing), for example, the medial cortex of a subtrochanteric fracture, depends on the area of bone in contact, which directly decreases as bone becomes osteoporotic. Buttressing by the opposite cortex is very important in maintaining fixation stability (Fig. 4–2). A fixation system is a bridge across a fractured region and as such consists of two generic components, the bone-implant interface device (such as screws, pins, or hooks) and the bridging device (rod, external fixation, or plate) and its connector to the bone-implant interface device. From this perspective, it is clear that the bone-implant interface device is the one directly affected by osteoporotic bone. The following discusses the mechanics of bone-implant interface devices first, followed by the mechanics of devices such as intramedullary rods, plates, external fixators, and spinal devices. A screw is a device that converts torque (rotary force) applied to its head into compressive force between the two surfaces it has engaged, as shown in Figure 4–3. The pullout strength of a screw in trabecular bone depends on the following (Fig. 4–4): its outside diameter, its length of engagement, the density of bone into which it is embedded, and its thread shape4–9 (and for very small screws, the material from which it is made). When a screw pulls out of trabecular bone it does so by cutting or shearing with it a core of bone, cutting the core as the threads move outward and trapping the core within the threads (Fig. 4–4). The properties of the outer surface of the core of bone define the screw purchase strength since failure occurs at this interface. If the outer core is smaller in diameter, shorter, or if the bone material at the interface has lower shear strength (due to loss of bone mass), the pullout strength of the screw will decrease. If the density of trabecular bone decreases to half that of normal bone, its shear strength decreases to nearly one quarter; therefore a screw of four times the diameter would be required to produce holding power equivalent to that of normal bone in this example (which is usually impractical). Increasing the number of screws or augmenting the density of bone around the screw are strategies that have been used to restore screw pullout strength. FIGURE 4–3 A screw is a device that through the action of its thread, essentially a circular inclined plane, converts rotary motion applied to the head into a linear motion, pulling together two surfaces and converting the applied torque to compressive force between the surfaces. FIGURE 4–4 Schematic diagram showing the effect of outer diameter, length of engagement, and shear strength of bone on pullout strength. In cortical bone, loss of purchase strength due to thinning of the cortex affects fatigue properties due to the mechanism shown in Figure 4–5.10 In this example, a plate fixed with screws bridges a fracture. The bone can transfer load to the plate by two methods, friction between plate and bone and the direct interaction of the shaft of the screws against the plate. The preferable mechanism is frictional contact, because plate contact against the shaft of the screw causes the screw to bend and cyclic bending can result in rapid early fatigue failure of the screw.10 The ability to generate frictional force between the plate and bone, and therefore its fatigue resistance, is directly related to the torque that can be applied to the screw. Maximum screw torque, in cortical bone, is directly related to the length of engagement of the threads. Therefore cortical thinning reduces the length of engagement, the maximum screw torque that can be applied, and the frictional force between plate and bone, thus increasing the potential for fatigue failure. Pins, such as K-wires or those used in ring-type fixators, are devices that resist tension and bending forces. The bending stiffness of a pin depends upon the material from which it is made, its diameter, its unsupported length, and the longitudinal tension applied to it. As Figure 4–6 shows, doubling the length of a pin decreases its bending stiffness by a factor of 8. More important, decreasing the diameter of a pin by one half reduces its bending stiffness by a factor of 16. This is because the moment of inertia of a pin is related to the fourth power of its radius. The moment of inertia is a geometric property that defines how the material is distributed around a cross section of an object. The further the material is located away from the center, the stiffer the object will be (which explains the configuration of an I-beam). Pretensioning a pin applies an initial stress to it. Since bending a pin produces tension on one surface and compression on the opposite surface, when a prestress is applied, this superimposed tension must be overcome before the pin will bend, effectively stiffening the pin (Fig. 4–6).11 FIGURE 4–5 Mechanism of fatigue failure of screws in cortical bone due to sliding of the plate and bending of the screw shaft. FIGURE 4–6 (Upper) Bending stiffness of a pin decreases as its unsupported length increases and as its diameter decreases; (lower) applying tension along the pin creates a force that opposes the bending force acting on the pin.
MECHANISMS OF INTERNAL
FIXATION OF BONE
THE EFFECT OF OSTEOPOROSIS ON FIXATION
MECHANICS OF BONE-IMPLANT INTERFACE DEVICES
BONE SCREWS
PINS
MECHANICS OF FIXATION DEVICES
EXTERNAL FIXATION
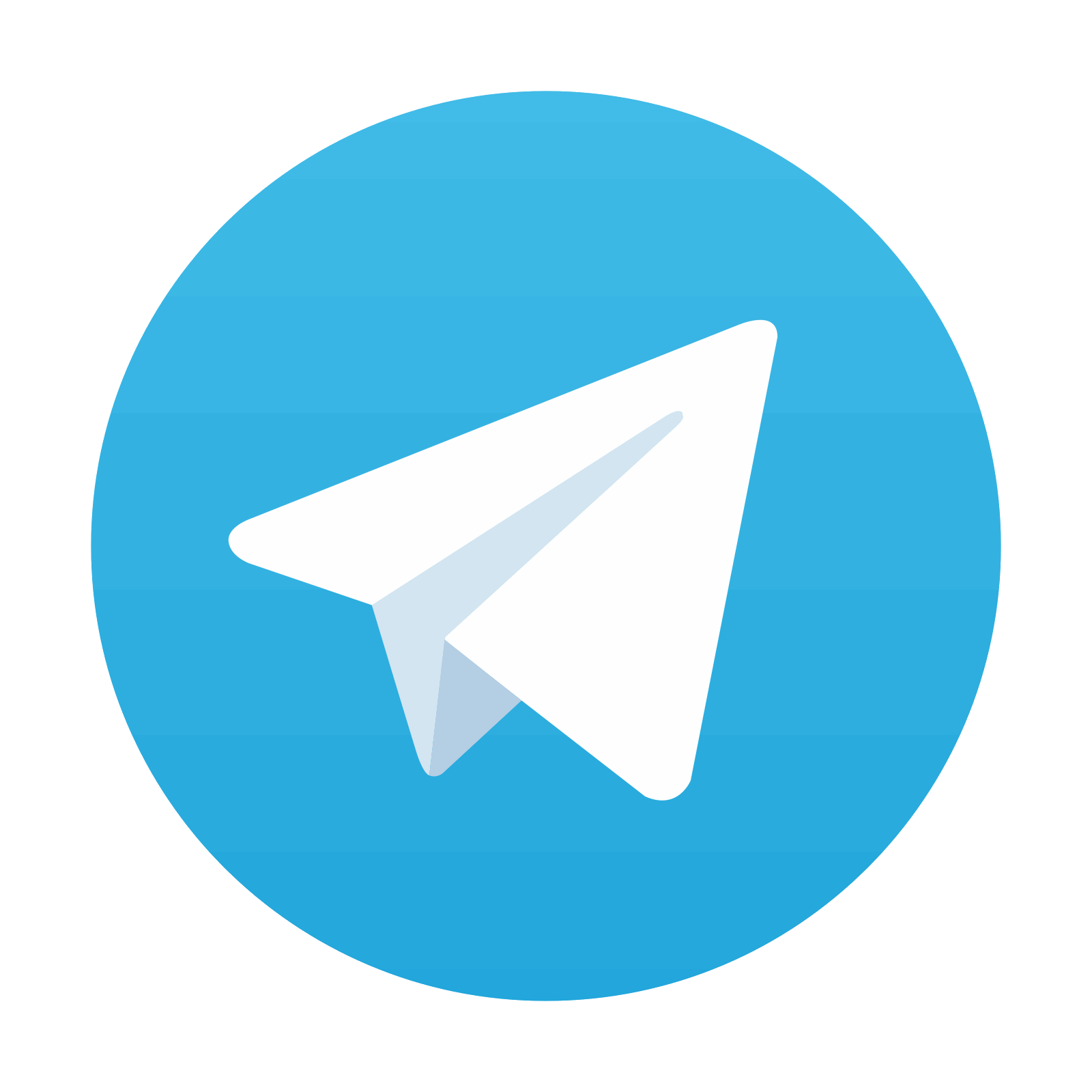
Stay updated, free articles. Join our Telegram channel

Full access? Get Clinical Tree
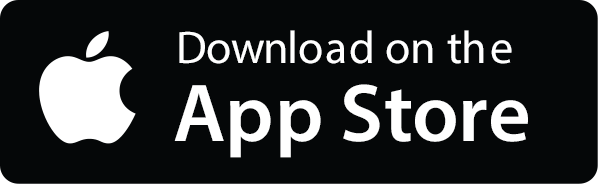
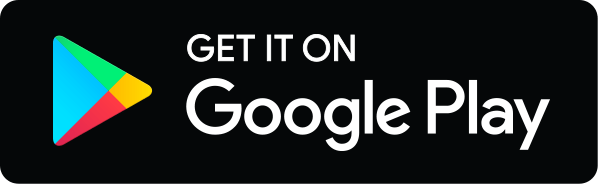