Chapter 40 Patellofemoral Disorders
Correction of Rotational Malalignment of the Lower Extremity
INTRODUCTION
This chapter reviews the concept of torsional malalignment of the lower limb and its importance on patellofemoral (PF) pathology. This topic was first well reviewed by Stan James, M.D., in which he introduced the term “miserable malalignment.”15 His classic description is highly recommended reading.
Confusion exists in the literature regarding the term malalignment. Insall and coworkers13 in 1976 defined patellar malalignment as being either an increased Q-angle or a high-riding patella. No other options were considered. Then, Insall and coworkers observed that “an increased Q-angle is usually associated with increased femoral anteversion and external tibial torsion. In the presence of these abnormalities when the hip and ankle joints are normally aligned, the patella faces inward and motion of the knee occurs about an axis which is rotated medially compared with the axes of the hip and ankle joints.” These authors recommended a medial tibial tubercle transfer or “patellar realignment” to treat this torsional malalignment, and it is no small wonder that the investigation reported that 61% of the patients remained symptomatic. Clearly, the underlying pathoanatomy had not been addressed. Tibial tubercle transfer has been shown to cause external rotation of the tibia on the femur rather than pull the patella medially into the trochlea.17 In addition, this procedure has been shown to increase medial compartment loading20 and medial facet loading. This author does not use the term “patellar malalignment” or “patellar realignment,” and reserves the term malalignment to refer only to a limb that deviates from normal in any one plane or any combination of the three anatomic planes: coronal (frontal), sagittal, or transverse (horizontal). Limb alignment is a direct result of the shape of the bones in the limb, but malalignment may be the result of acquired cartilage or ligament loss in which the deformity exists at the joint and not the bones.
Over 50 independent observations have been reported in the literature to be associated with anterior knee pain, chondromalacia, patellar instability, or patellar malalignment (Table 40-1). Whereas these factors may be very important, objective measurement tools are lacking. No study has ever tried to assess all these variables in a single cohort of patients, and even if this were undertaken, the lack of objective measurement techniques would make the study invalid. If these variables are indeed important, then any study would need to incorporate accurate measurements of each. The relative importance of each will need to be developed. Without this, clinicians are left with a realization that data do not exist to guide in the knowledge of pathomechanics, diagnosis, and treatment and that we depend only on unrelated clinical observations and some very basic laboratory studies.
TABLE 40-1 Cited Contributions to Patellofemoral Dysfunction
Genu valgum | Genu varum |
Femoral anteversion | Femoral retroversion |
Excess external tibial torsion | Excessive internal tibial torsion |
Outerbridge ridge | Trochlear dysplasia |
Shallow trochlea | Short lateral trochlea |
Increased Q-angle | Decreased Q-angle |
Excessive lateral quadriceps pull | Insufficient vastus medialis obliquus |
Decreased patellar surface contact area | Abnormal patellar spin |
Patellar dysplasia | Patella alta |
Patella baja (infera) | Menisectomy |
Laxity of ACL, PCL, MCL, or FCL | Rotatory instability |
Iliotibial band contracture | Quadriceps contracture |
Achilles contracture | Retinacular contracture |
Retinacular laxity | Hyperpronation |
Pes planus | Tibial tubercle–trochlear groove distance (TT/TG) |
Patellar malalignment | Patellar instability |
Patellar subluxation | Medial patellar dislocation |
Lateral patellar dislocation | Chondral softening |
Genu recurvatum | Patellar tilt |
Patellar shift | Abnormal (mal) tracking |
VMO dysplasia | J sign |
A sign | Bayonet sign |
Crossing sign | Trochlear bump |
Patellar thickness | Knee flexion contracture |
Infrapatellar contracture | VMO/VLO ratio |
Rearfoot varus | Patellar glide |
Quadriceps tendon width | Flexor hallucis longus tendon entrapment |
Flexor hallucis dysfunction | Increased lumbosacral instability |
Hip flexion contracture | Abdominal oblique: rectus femoris + psoas imbalance |
Quadriceps atrophy | Pelvic abductor weakness |
Increased thoracolumbar extension | Inward pointing knee |
Female gender |
ACL, anterior cruciate ligament; FCL, fibular collateral ligament; MCL, medial collateral ligament; PCL, posterior cruciate ligament; VLO, vastus lateralis obliquus; VMO, vastus medialis obliquus.
Table 40-1 shows the variables related to PF syndrome, anterior knee pain, PF instability, chondromalacia, and PF arthritis. The contributions of these variables need to be defined, measured, and validated before any meaningful outcome studies can be completed. It is critical to simplify the confusing clinical picture created by so many variables. Toward this end, this author considers that there are only four independent but related variables to assess. Each of the conditions just discussed can be fitted into one of these four: skeletal geometry, ligaments, articular cartilage (patella and trochlea), and musculotendinous units.
BASIC ASSUMPTIONS
Critical Points BASIC ASSUMPTIONS

This author uses physical examination, coronal standing radiographs, sagittal radiographs, and rotational computed tomography (CT) studies to assess limb alignment. Physical examination and stress radiography43 are used to assess ligament insufficiency. Double-contrast CT arthrography with fine cuts determines the thickness of articular cartilage and the location and size of various focal defects. The usual clinical tests of strength, orientation, and flexibility (e.g., Ober test, prone rectus test, straight leg raising, Achilles contracture) are used to assess musculotendinous units.
Patellar instability results from a loss of the patellar stabilizers and often an excess of displacement forces. The patellar stabilizers are the trochlear groove and the ligaments (both the medial and the lateral patellofemoral ligaments) and the medial and lateral meniscopatellar ligaments. Brattström,2 as early as 1964, demonstrated that in recurrent dislocation of the patella, a flattening or dysplasia of the trochlear groove is nearly always present. Dejour and colleagues6 demonstrated that this dysplasia is often in the proximal portion of the trochlea not seen on an axial patellofemoral radiograph. This can be measured on a true lateral knee radiograph by recognizing the floor of the groove and the anterior projections of the medial and lateral condyles. For lateral patellar dislocation to occur, the medial patellofemoral ligament (MPFL) must be injured. A number of authors including Christoforakis and associates4 and Desio and colleagues8 have demonstrated in the laboratory that the lateral retinaculum provides up to 19% of the resistance against lateral displacement; thus, the patella is usually more unstable after lateral retinacular release. Medial dislocation is a distinct possibility and this author reported 70 cases confirmed with stress radiography in 1990 (Annual Meeting of the American Academy of Orthopaedic Surgeons). The diagnosis of instability requires a force and displacement examination and stress radiography may provide documentation (Figs. 40-1 and 40-2), because unstressed radiographs are normal but stress application demonstrates obvious excessive displacement.
A frequent contributing cause of patellar dislocation is the existence of an abnormally high displacement force. This most often occurs when the direction of the lateral quadriceps vector is increased. This commonly occurs when the femur twists away from under the patella, most often in the direction of medial rotation. This is usually due to some torsional abnormality of the lower limb (Fig. 40-3). In the face of such excess displacement forces, a PF ligament reconstruction may fail.
Arthrosis or chondromalacia exists when articular cartilage fails as the force per unit area exceeds biologic tolerance. For acute fractures of articular cartilage, this may be in the range of 20 MPa. In the case of chronic overload due to a too small articular surface area or too much body weight, muscle loading or long lever arms with chronic pressure exceeding 5 MPa may lead to arthrosis. Articular fractures exist approximately 90% of the time after acute patellar dislocation.31 Although magnetic resonance imaging (MRI) is often used to demonstrate articular cartilage lesions, Figure 40-4 gives an example of pathology clearly seen with double-contrast CT arthrography not visualized on MRI. Rotational malalignment is often a major contributing cause of pain, instability, and cartilage injury, but the evidence of how much benefit can be derived from rotational osteotomy in the presence of instability and arthrosis is unknown.
HOW DOES LONG BONE TORSION AFFECT THE PF JOINT?
The knee joint axis moves straight forward during gait, with a small (<10°) turning inward and outward.27 The foot also tends to move in a fairly constant direction (foot progression angle).28,37 The geometry of the lower extremity skeleton largely determines the direction of load applied at the PF joint, with the amount of load depending on the body mass, the length of the lever arms, the surface area of the PF joint, and the velocity of the moving system. If the knee joint axis is twisted out of its normal plane of flexion and extension while the quadriceps is contracting, a side-directed force is created that acts on the patella, attempting to displace it. This causes an increased strain on the PF ligaments and retinaculum and an imbalanced direction of force on the PF articular surface.
Critical Points HOW DOES LONG BONE TORSION AFFECT THE PATELLOFEMORAL JOINT?
If these side-directed vectors exceed biologic tolerance, either instability or arthrosis may result. For example, an inward pointing of the knee increases the lateral direction of pull of the quadriceps. Therefore, the pull on the MPFL (and also medial retinaculum and medial meniscopatellar ligament) is increased and the direction of pressure on the patella is altered, creating an increase on the lateral facet and a decrease on the medial facet (Fig. 40-5). The radiographic appearance of subchondral density under the patellar facets is a useful clue to the mechanical environment (Fig. 40-6).
Maximum energy conservation during gait is due to precise anatomy and joint kinematics.34 Abnormalities in normal bony anatomy or joint motions distort the normal force vectors needed to transfer the body mass to the ground. Deviations in normal limb alignment may result in the knee joint flexion-extension axis advancing sideways while the body moves forward. These deviations include femoral anteversion or retroversion, excess internal or external tibial torsion, genu valgum or varus, hyperpronation, and Achilles contracture.
The foot progression angle (FPA) is generally defined as the angle between the long axis of the foot and the direction of body progression and averages 10° to 15°.28,37 It has been shown that the FPA remains similar despite differences in the torsion of the tibia or femur.37 It is likely that this is because proper ankle dorsiflexion cannot occur during gait if the ankle joint axis is not aligned with the direction of forward movement or because this presents the most stable position of the foot on the ground. Hip rotation must vary if the torsion of the long bones changes and the FPA stays constant. Alterations in both femoral and tibial torsion change the effective lever arm of the hip stabilizers1 and may account for the frequency of soft tissue complaints around the hip and pelvis, as well as the increased pelvic tilt and lumbar lordosis seen in these patients. Examples of the change in the position of the hip and knee with a constant foot progression angle and changes in femoral and tibial torsion are seen in Figures 40-7 to 40-11.
Figures 40-7 to 40-11 are drawn with an FPA of 13° (Seber average) for a normal male torsional alignment (Fig. 40-7), a normal female torsional alignment (Fig. 40-8), a female with 30° excess external tibial torsion (Fig. 40-9), a female with 30° excess femoral anteversion (Fig. 40-10), and a female with both 30° excess tibial external torsion and 30° excess femoral anteversion (Fig. 40-11). One may study the variations in knee joint progression with these common abnormal patterns. Yoshioka and associates47–49 found identical femoral anteversion in both males and females, equal genu valgus in both males and females, but an increase in external tibial torsion and in foot external rotation in females over males. This increase in external foot rotation may account for the apparent increased genu valgus in females, the increased incidence in PF symptoms in females, and even for the increased incidence of ACL tears in females.
BIOMECHANICAL EVIDENCE RELATING LIMB TORSION WITH PF INSTABILITY AND PAIN
A change in PF contact areas and pressures was noted after alteration of femoral or tibial torsion or rotation by Lee and coworkers22–24 Hefzy and associates,12 and van Kampen and Huiskes.45 Kiljowski and colleagues18 (unpublished data) placed lower limbs (femoral head to foot) in a frame with a clamp, attaching the femoral head to a vertical post that allowed the knee flexion to be altered by sliding the femoral head up and down the post. The foot was held in a position of normal FPA and an osteotomy was performed in the shaft of the femur, allowing rotation. PF contact pressures and MPFL strain were measured while the osteotomy changed the femoral torsion 15° and 30°, both inward and outward. At 30° knee flexion, lateral facet pressure increased an average of 30%, with a 30° increase in femoral anteversion, while medial facet pressures were decreased. Strain in the MPFL increased 57% after a 30° increase of internal femoral torsion. Fujikawa and coworkers11 in a biomechanical study that measured PF contact pressures concluded that if an angular deformity and a torsional deformity coexist, the rotatory component causes the greater PF changes. These authors also noted that when a varus deformity was created, the greater the varus deformity, the less the PF congruence.
CLINICAL EVIDENCE RELATING LIMB TORSION TO PF INSTABILITY AND PAIN
Brattström2 in 1964 measured trochlear depth in a group of patients with recurrent dislocation of the patella and confirmed that a shallow trochlea was always present. This author also defined the Q-angle and pointed out the change in Q-angle as a result of changes in limb rotation. He reviewed the literature on the rotational femoral osteotomy as described by Graser (1904), Fürmeier (1953), Kiesselbach (1956), and Vinditti and Forcella (1958) and concluded that the “femoral osteotomy is a big operation that demands prolonged after treatment. I have not encountered any instance of recurrent dislocation of the patella that justified my suggesting an operation of this nature to the patient.” Takai and associates41 measured femoral and tibial torsion in patients with unicompartmental arthrosis in either the medial, the lateral, or the PF compartment and found the highest correlation to be that of PF arthrosis with increased femoral anteversion (23° anteversion in the osteoarthritis group vs. 9° anteversion in the control group). Janssen16 found a high correlation between patellar dislocation and an increase in medial femoral torsion and speculated that this medial femoral torsion was also responsible for the development of trochlear dysplasia. Lerat and colleagues25,26 noted a significantly increased association of internal femoral torsion with both patellar instability (P < .0001) and patellar chondropathy (P < .001). Stroud and coworkers40 followed 92 patients who at age 5 showed 30° greater medial hip rotation (measured in extension) than lateral rotation. At age 24, PF pain was noted by 30% in the increased medial rotation group compared with only 8% in the control group (P < .001). Winson and associates46 found that 70% of adolescents undergoing arthroscopy for anterior knee pain had an increased internal torsion of the femur, compared with only 33% of those undergoing arthroscopy for a meniscal or cruciate injury. Turner44 noted increased external tibial torsion in patients with PF instability (25° vs. 19°). Of perhaps great significance to those who believe that muscle strengthening is the key to treating PF symptoms, Nyland and colleagues32 found a significant decrease in vastus medialis and gluteus medius electromyogram amplitude in athletes with clinically increased internal femoral torsion. Arnold and coworkers1 noted that an increase in femoral anteversion of 30° to 40° and decreased abduction moment arm strength of 40 to 50% was enough to impair normal walking, and therefore, those individuals required turning the knee inward to keep the hip from collapsing. This inward pointing of the knee creates high shearing force on the PF joint.
FEMORAL AND TIBIAL TORSION MEASUREMENTS
Femoral anteversion has been measured by a variety of techniques by many authors and recently by Yoshioka and associates47,48 (Fig. 40-12). To measure limb alignment in the horizontal (transverse) plane, a CT rotational study is obtained. This is a CT scan that overlaps cuts from the femoral head, base of the femoral neck or lesser trochanter, the knee joint (either tangent to the posterior condyles or between the medial and the lateral epicondyles), the proximal tibia at the joint and the tibial tuberosity, and the ankle joint (Fig. 40-13). These cuts allow measurement of femoral torsion, tibial torsion, knee torsion, and the tibial tubercle–trochlear groove (TT/TG) distance. CT also allows for the observation of trochlear depth or dysplasia, the position of the patella in the trochlea (unstressed), patellar tilt, and shift and the density of the subchondral trabeculae.
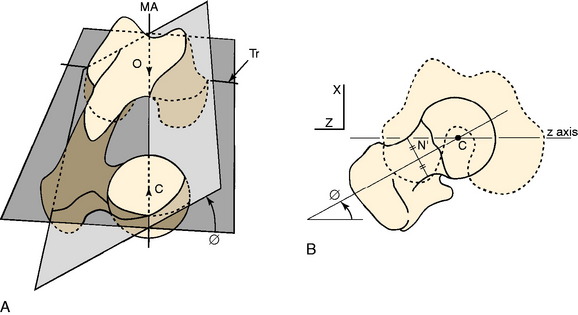
FIGURE 40-12 A, Femoral anteversion or antetorsion (angle ϕ here) may be measured along the center of the femoral neck from the center of the femoral head to the center of the femoral shaft at the base of the neck, and distally either along the transepicondylar axis (Tr) 7.4° or along the tangent of the posterior femoral condyles 13.1°. These differ by about 6°, with a range of 11° retroversion to 22° anteversion. B, This measurement by Yoshioka and associates48 is taken directly off the bone. Murphy and coworkers30 validated a CT measurement along these same lines.
(A, From Yoshioka, Y.; Cooke, T. D. V.: Femoral anteversion: assessment based on function axes. J Orthop Res 5:86–91, 1987, p. 88. Fig 3A; B, from Yoshioka, Y.; Siu, D.; Cooke, T. D. V.: The anatomy and functional axes of the femur. J Bone Joint Surg Am 69:873–880, 1987, p. 875, Fig. 2B.)
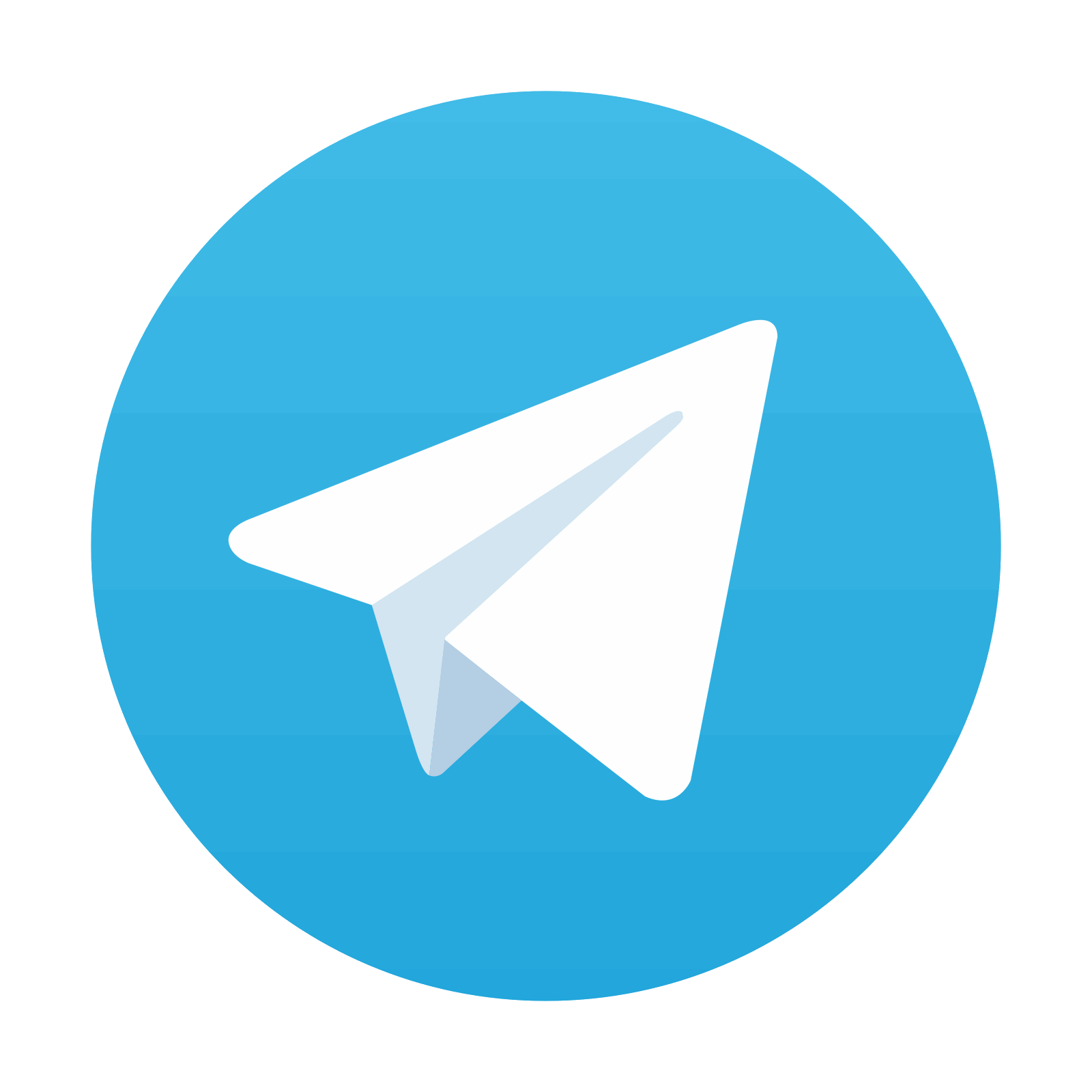
Stay updated, free articles. Join our Telegram channel

Full access? Get Clinical Tree
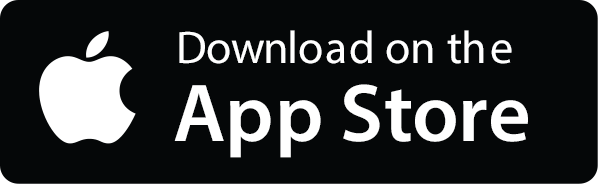
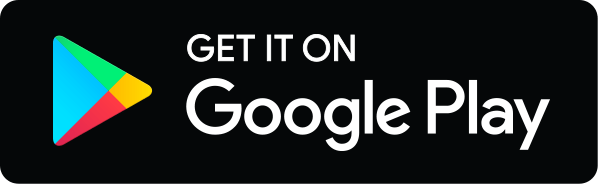