Chapter 111 Pancreatic Enzymes
Introduction
Pancreatic enzymes produced by the body are well known for the integral role they play in the digestion of the foods we eat. Pancreatic juice contains numerous enzymes, including amylase, lipase, cholesterol esterase and phospholipase, and the proenzymes trypsinogen, chymotrypsinogen, and procarboxypolypeptidase, which are converted in the small intestine to their active forms trypsin, chymotrypsin, and carboxypeptidase, respectively.1
Foods in the human diet are composed primarily of protein, carbohydrates, and fats. Protein leaves the stomach predominantly in the form of proteoses, peptones, and large polypeptides.2 Upon reaching the small intestine, these are further digested by the proteolytic enzymes trypsin, chymotrypsin, and carboxypolypeptidase. Protein digestion primarily occurs in the duodenum and jejunum. Carbohydrates are digested by α-amylase in the pancreatic juice, which breaks starches (converting them into maltose and other small glucose polymers), whereas pancreatic lipase, cholesterol esterase, and phospholipase digest fats.1,3
History
In 1934, Dr. Ernst Freund, a Viennese physician, studied the blood of people who were free from cancer and discovered a substance that had the ability to dissolve cancer cells. Patients with cancer did not have this material, which Freund called “normal substance.” In the early 1930s, Professor Doctor Max Wolf worked with Freund in Vienna and successfully identified “normal substance” as an enzyme that decomposes fatty materials and proteins. For his work in the field of enzymes, Wolf is generally considered the father of modern enzyme therapy. The work of Freund and John Beard sparked Wolf’s interest in the possibilities of treating malignant diseases with enzymes. He subsequently founded the Biological Institute of New York City and, after studying various enzymes and enzyme combinations, developed what he regarded as an optimal preparation for the treatment of various acute and chronic conditions. His preparation was a combination of a fractionated hydrolysate of beef pancreas, calf thymus, Pisum sativum (common pea), Lens esculenta (edible lentil), mannitol, and Carica papaya (papaya, a source of the enzyme, papain).
Pancreatic Enzyme Supplements
The pancreatic enzyme supplements most commonly used in health care are chymotrypsin, trypsin, pancrelipase, and pancreatin. Chymotrypsin and trypsin are proteolytic enzymes that break proteins into peptides. Chymotrypsin liberates the amino acids, L-tyrosine, L-tryptophan, and L-phenylalanine, and other molecules, including several synthetic esters and amides.4 Trypsin hydrolyzes primarily lysyl and arginyl residues. Pancreatin contains amylase (which breaks down starch), lipase (which breaks down fats), and protease (which breaks down proteins). Pancrelipase is similar to pancreatin, but with a higher concentration of lipase.
According to the U.S. Pharmacopeia (USP), chymotrypsin and trypsin are routinely crystallized from ox pancreas gland extract, and pancreatin from both hog and ox sources, whereas pancrelipase is derived from hog pancreas.5 Porcine pancreas is especially rich in amylase and lipase, and is similar to the human pancreas.4 Bovine pancreas contains considerable amounts of proteolytic enzymes, but substantially lower amounts of lipase and amylase.4 Germany, Japan, England, India, and other countries utilize their own pharmacopeia, and foreign companies may use other sources to formulate their enzyme products.
Enzymes extracted from animal sources are sensitive to environmental changes, so manufacturers take particular care during extraction to control pH (usually with buffers), temperature (using precooled solutions and apparatus), substrate, and proteolysis (controlled through the use of inhibitors) to render a product that is enzymatically active.6
Enzyme Standardization
In 2010, the Food and Drug Administration (FDA) began requiring manufacturers of pancreatic enzyme products to test them in clinical trials if those products were prescribed to treat individuals with pancreatic diseases. Until that time, there were frequent inconsistencies in enzyme formulation. For example, a study of six different enzyme preparations found that, in some cases, the actual lipase activity in a product was more than twice that listed on the label.7 Another study on nine pancreatic enzyme products found that, although within USP limits, the percentage of lipase activity after dissolution was not always equal.8 These inconsistencies in content and activity level could unexpectedly alter patient enzyme requirements.
Absorption of Proteins
In the past, it was believed that the intestinal epithelial mucosa was impermeable to large protein molecules.9 However, research over the past several decades has shown that the intestinal epithelium can be crossed by macromolecules, including intact proteins such as proteolytic enzymes.10
These macromolecules normally penetrate the mucosal surface via the transcellular route as, in healthy mucosa, the tight junctions (zonula occludens) between the enterocytes prohibit paracellular passage.11 Binding to the luminal membrane of the enterocyte is followed by phagocytosis.12 Some of the vacuole membrane vesicles formed fuse with lysosomes, and within the resulting phagolysome, the peptides and proteins may be hydrolyzed by lysosomal enzymes.13 Other macromolecules avoid intracellular digestion and are passed from the enterocytes through the basolateral membrane into the interstitial space.14 In the interstitial space, the macromolecules become available to macrophages and lymphoid cells.15 Those molecules not taken up by macrophages or lymphatic cells eventually pass from the interstitial space into the blood or lymph.16
The transport of macromolecular material from the lumen to the interstitium has been extensively studied in the epithelium covering the lymphatic structures, such as Peyer’s patches or isolated follicles.12 In these regions, specialized enterocytes, the follicle-associated epithelium cells17 or M-cells18 (so called because of their occurrence in the microfolds of the luminal surface), transport macromolecular material in both directions.17 The gut-associated immune system is thus supplied with antigenic macromolecules from the intestinal lumen.19 The immunoglobulins produced by the plasma cells in the lamina propria (mainly immunoglobulin-A) are transported transcellularly to the luminal surface.
The exact level of the intestinal absorption of intact molecules or large breakdown products of dietary proteins is not yet totally clear and can vary by individual.20 Although it is generally assumed that, apart from a very small proportion, all protein is hydrolyzed into amino acids or low-molecular-weight peptides before absorption by the mucosa, some research supports the hypothesis that a considerable proportion of dietary protein is taken up in the form of macromolecules and is only then hydrolyzed intercellularly in the peripheral tissue into amino acids (a process called “distributed digestion”).21
Understanding the Absorption of Enzymes
Understanding the intestinal transport of macromolecules is especially important for understanding the functions and absorption of enzymes specifically. Hydrolases such as trypsin or elastase can be transported functionally intact into the bloodstream from the lumen of the gut. These circulating proteinases are bound to antiproteinases, such as alpha2 macroglobulin or alpha1 antiproteinase,22 and can be resorbed from the main bloodstream by pancreatic cells (enteropancreatic circulation as an enzyme conservation process).23 Thus, the intestinal absorption of intact enzymes appears to be important for the balance between hydrolases and antiproteinases in the intracellular space,24 and is an important factor for the establishment and maintenance of the internal stability in the body.
Although there are a number of absorption mechanisms, the primary mechanism for the enteral absorption of enzymes and other macromolecules is pinocytotic transfer by the M-cells of the small intestinal epithelium. The enzymes connect to a receptor in the intestinal wall mucosa and are then absorbed into the wall by pinocytosis, guided through the intestinal cells in vesicles, and finally released into the blood by exocytosis.25
To clarify rate of absorption, Steffen et al26 investigated the absorption of an enzyme mixture “A” (EMA), which contained 100 mg of pancreatin, 60 mg of papain, 10 mg of lipase, 10 mg of amylase, 24 mg of trypsin, 1 mg of chymotrypsin, 45 mg of bromelain, and 50 mg of the bioflavonoid rutin in rabbits. Using electrophoresis, these researchers found that entire enzyme molecules were absorbed. Although enzyme particles were also present, the ratio to the entire amount administered was not measured. EMA was found in both lungs and liver after 1 to 2 hours. After 1 to 4 hours, approximately twice as much EMA was found in the liver as in the lungs. The absorption maximum in all animals occurred approximately 1 hour after administration. After 24 hours, EMA was no longer found in either the lungs or liver.
The absorption rate of individual and combined enzymes can be found in Table 111-1.27,28 The absorption rate of orally-ingested EMA is about 20% within 6 hours.19
TABLE 111-1 Absorption Rate of Individual and Combined Enzymes (within 6 Hours)27,28
ENZYME | ABSORPTION RATE (%) |
---|---|
Amylase | 45 |
Chymotrypsin | 14-16 |
Pancreatin | 18-19 |
Papain | 6 |
Trypsin | 26-28 |
Enzyme combination (bromelain, chymotrypsin, pancreatin, papain, and trypsin, with the bioflavonoid rutin) | 22 |
Factors Affecting Enzyme Activity
Optimal pH Range
Each enzyme has an optimal pH range, depending on such variables as temperature and substrate concentration at which the enzymatic catalytic reaction occurs most rapidly. Chymotrypsin has an optimum pH of 8.0, has reversible denaturation at a pH below 3.0, and becomes inactive at a pH above 9.0.4 Trypsin has an optimum pH between 7.0 and 9.0, is stable at a pH of 3.0 (and at low temperature), and is irreversibly denatured at a pH of 9.0 or higher.4 The pH of the normal human stomach is 1.5 to 3.0,29 low enough to denature or inactivate some or all of a pancreatic enzyme supplement if it is not enterically coated or otherwise treated to protect it from a low-pH environment.
The Effects of Temperature
In general, an increase of 50° F (10° C) in the enzymatic environment approximately doubles the rate of the chemical reaction.30 However, because enzymes are proteins, excessively high temperatures can denature them, thus destroying their activity. Optimum temperature for an enzyme is the temperature at which the catalyzed enzymatic reaction progresses most rapidly without damage to the enzyme. This temperature can vary by enzyme. This is a good rationale for avoiding hot beverages when taking enzyme supplements.
Cofactors
Although all enzymes consist of protein, some are complex proteins, that is, they have a protein component and a “cofactor.” If the cofactor is removed, the protein (no longer active enzymatically) is called the apoenzyme. A cofactor might be a metal (e.g., iron, magnesium, copper, or zinc), a prosthetic group (a moderately-sized organic molecule), or a coenzyme (small organic compound). Prosthetic groups and metals can aid in the catalytic function of the enzyme, whereas coenzymes take part in the enzymatic reaction. Many vitamins, trace elements, and minerals essential to human bodily function are part of enzymatic cofactors. So the physician must ensure that his or her patients take multivitamins and multiminerals to “feed” their enzymes.
Supplement Coating
The pH of the stomach’s hydrochloric acid secretions is about 0.8.31 This low pH inhibits bacterial growth and activates certain enzymes. This acidic nature, however, can destroy pH-sensitive supplemental enzymes. For this reason, many enzyme products are enterically coated. This coating allows the product to reach the small intestine before disintegrating. Other products are encapsulated in “microspheres,” delaying disintegration. For example, pancreatic protease encapsulated with a mixture of cellulose acetate phthalate and maize starch can remain stable in simulated gastric conditions (pH of 3.97) for at least 3 hours.32 This would theoretically provide enough time for the capsule to pass through each part of the gastrointestinal tract. The capsule then disintegrates rapidly under pH 6.82 and temperature of 39.5° C (as would occur in the small intestine).32
Nanotechnology is opening a new field for the delivery of enzymes and other small proteins. Nanotechnology is the study of matter as small as one billionth of a meter. According to the National Nanotechnology Initiative, nanoparticles are being used in timed-release drug delivery.33 Enzymes can be attached to nanoparticles and actually maneuvered to destroy diseased cells. Research on nondegradable nanocapsules showed that proteins can be efficiently transported to individual cells, surviving different pH levels.34 So, it is no wonder that enzymes (which are proteins) can also be attached to nanoparticles and used to treat disease at the cellular level.
Clinical Applications
Dr. Peter Streichhan, a well-known enzyme researcher, stated that certain enzymatic mixtures have a broader range of action than pancreatin, bromelain, or any other standardized monohydrolytic preparation—this is because certain enzyme mixtures characteristically possess differences in optimal pH and also differences in reactive properties of the proteolytic, lipolytic, and/or amylolytic-acting hydrolases.35
Clinical uses of individual enzymes can be found in Boxes 111-1 through 111-5, whereas clinical uses of combinations can be found in Box 111-6. For more information on how enzymes can treat more than 150 conditions, please see the author’s book, The Complete Book of Enzyme Therapy.
BOX 111-1 Clinical Applications of Chymotrypsin
• In debridement, treatment of abscesses and ulcerations, liquefaction of mucous secretions36
• In ophthalmic cataract surgeries and therapy of eyeball hematomas and ophthalmorrhagias36,37
• Before and after tooth extractions, as well as in operative dentistry38,39
• After episiotomy procedures40
• As an anthelmintic against enterozoic worms41
• In early recognition of tumor cells42
• In histologic gastroenterologic diagnostics43
• In inflammatory conditions (local and systemic) to promote the dispersion of blood extravasates and effusions from fractures37,44–51
• Accidental soft tissue trauma44–4851
• Intervertebral disc lesions52
• In uveitis vitreous hemorrhage, diabetic retinopathy, and asthmatic symptoms53
BOX 111-2 Clinical Applications of Trypsin
• In debridement of necrotizing wounds, ulcerations, abscesses, empyemas, hematomas, fistulas, and decubitus54–57
• To accelerate healing in injuries, inflammations, phlogistic edemas, and traumatic changes44–51
• As an auxiliary agent in meningitis therapy58
• As an ointment or dressing (wet or dry)4
• As a liquid or an aerosol to liquefy sputum in bronchial disorders and in the preparation of sputum for cytologic examination4
• As an anti-inflammatory agent; oily suspensions are injected intramuscularly4
• As an aid in the treatment of intraocular hemorrhage, thrombophlebitis, intestinal obstruction (due to cirrhosis or carcinoma)59
BOX 111-4 Clinical Applications of Lipase
• In pancreatin-containing remedies to increase pancreatic/lipolytic activities (replacement therapy)63–66
• When given with pancreatin (in combined preparations), it reduces fat level in stools67–69
• Synergistically intensifies the activity of lipoprotein-lipase in the blood70 and migration of agranulocytes71
BOX 111-5 Clinical Applications of Pancreatin
• In pancreatic insufficiency, inadequate secretion of exocrine pancreas, disturbed digestion, and after gastrectomy64,72–84
• In chronic pancreatitis85 and after surgery for chronic pancreatitis86
• In ductal obstruction from neoplasm (e.g., of the pancreas or common bile duct)85
• To treat severe cases of steatorrhea (as found in cystic fibrosis)87–92
BOX 111-6 Clinical Applications of Enzyme Combinations
• Reabsorption of hematomas93,98
• Meniscectomy (pre- and postoperative therapy)107,108
• Lower extremity bypass surgery114
• Acute and chronic bronchitis121–123
• Cystitis and lower urinary tract infections117,124,125
• Pelvic inflammatory disease127,128
• Post-thrombotic syndrome129–133
• Pathologic venous processes129,134–138
• Occlusive arterial disease139
• Soft tissue rheumatism (nonarticular rheumatoid syndrome)147,148
• Rheumatoid arthritis (chronic polyarthritis)117,122,131,142,149–164
• Ankylosing spondylitis (Bekhterev’s disease)94,153,154,157
• Degenerative rheumatic joint disease102,117
• Monoarticular, activated osteoarthrosis102,123,165
• Multiple sclerosis121,166–172
• Fibrocystic breast disease144,179
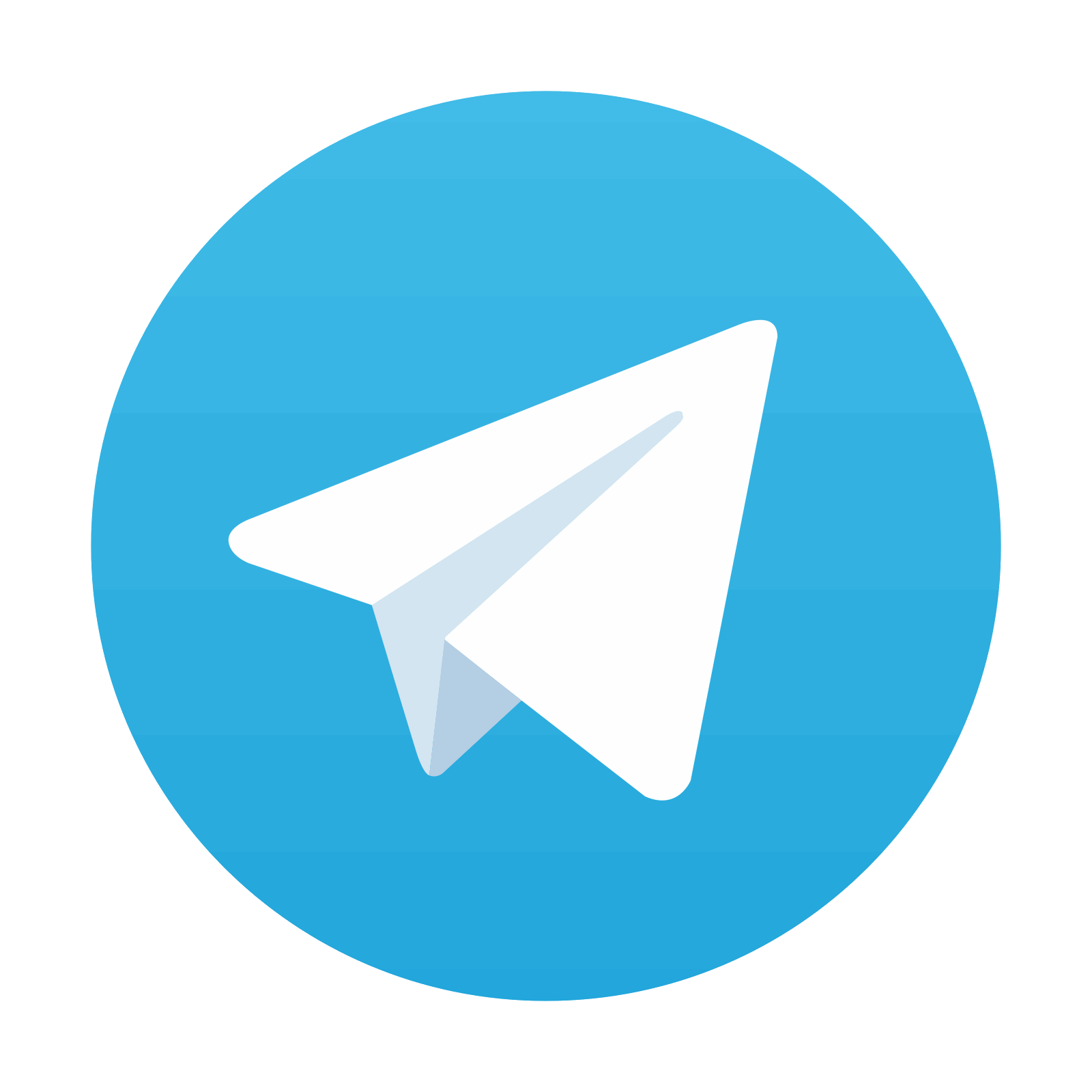
Stay updated, free articles. Join our Telegram channel

Full access? Get Clinical Tree
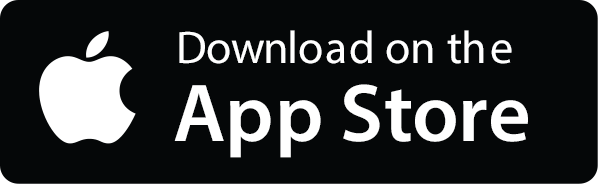
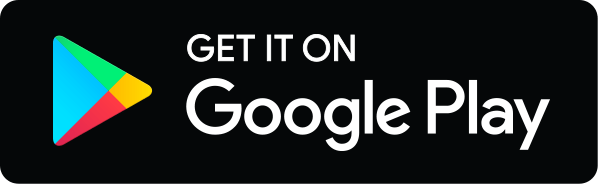