Osteomyelitis
Lawrence M. Oloff
Geoffrey S. Heard
Despite tremendous innovations in medical technology over the last 50 years, the basic tenets of management of contaminated bone have remained essentially unchanged. Early diagnosis is the cornerstone of successful treatment and prevention of the acute infectious process from becoming established as chronic osteomyelitis. Newer technologies have evolved that may be helpful in evaluating certain patients, but the clinical evaluation and assessment are still critical tools. Diagnostic modalities, such as magnetic resonance imaging (MRI), computed tomography (CT), and nuclear medicine scans are particularly useful in the early diagnosis of osteomyelitis and may contribute valuable information in cases with nonspecific symptoms. In the future, newer imaging techniques in nuclear medicine may prove to be revolutionary advancements in the diagnosis of infectious disease. Emerging technologies that use genetic engineering principles such as polymerase chain reaction (PCR) tests and biochips are promising strategies that may assist in identifying responsible pathogens at the molecular level. Even with such advanced presumptive diagnostic modalities, the demonstration of organisms in appropriately selected and retrieved bone remains the most definitive means of diagnosis.
The treatment of osteomyelitis typically combines varying degrees of both medical and surgical management, with the emphasis of care often being determined by the acuity or chronicity of the infection. The care of osteomyelitis by administration of antibiotics may be initiated in high-risk patients with minimal findings in whom antimicrobial prophylaxis is, in reality, the treatment of a potential infection. When surgical intervention is deemed necessary, incision, drainage, and the removal of sequestered or sinister material is the sine qua non of surgical therapy. When implanted materials are present, considerable judgment is required to determine the best approach to resolve the infection and, it is hoped, salvage the implant. Antibiotic selection remains subject to a variety of factors that must be considered if one is to choose the most appropriate agents, the determination of which is far from exacting.
The long-term management of patients with osteomyelitis involves judgments that often are made in the face of conflicting clinical or empirical data. Clinical experience will ultimately determine the amount and timing of bone resection; the type of wound management; the placement and use of drains, irrigation systems, antibiotic-impregnated beads, or pellets; the timing and type of wound closure; the termination or continuation of antibiotic therapy; and other related decisions.
CLASSIFICATION
The term osteomyelitis was introduced by Nelaton in 1844 to describe an infection of bone and marrow (1). It is derived from a combination of the words osteon (bone) and myelo (marrow) with -itis (inflammation). Suppuration of bone cortex without marrow extension is properly referred to as suppurative or infectious “osteitis” (2).
A variety of terms have been used to categorize the nature of bone infection and include acute, subacute, chronic, residual, recurrent, and refractory or recalcitrant osteomyelitis (3). However, these differentiations of osteomyelitis based on chronologic terminology can be misleading or confusing. For example, acute osteomyelitis is usually described as the presence of bone infection before the development of necrotic bone, while the presence of dead bone (sequestrum) is generally regarded to be the defining characteristic of chronic osteomyelitis. Using this nomenclature, the initial presentation of certain types of osteomyelitis, such as that due to a compound fracture or an early postoperative infection could by definition be considered chronic due to the presence of necrotic bone. Another example is the presentation of acute osteomyelitic signs and symptoms that occur in a previously asymptomatic patient years after surgery with internal fixation or implantation of prosthetic devices (4,5); yet radiographic signs may be consistent with apparent chronicity of the infectious disease. As a result of these apparent disparities between clinical and radiographic findings, it has been suggested that the terms “initial” and “recurrent” be used to describe osteomyelitis (6). In addition to these seeming incongruities, classifications based solely on temporal terminology are also of limited value as they neither imply the etiology nor confer a specific treatment regimen.
Presently, there are two osteomyelitis classification schemes most commonly utilized. In 1970, Waldvogel et al (3) divided bone infections into three groups, based on etiology. Those that were derived from blood were designated hematogenous. Those that resulted from the spread of infection from adjacent soft tissue were designated osteomyelitis secondary to contiguous focus. The third classification is osteomyelitis associated with vascular insufficiency and is most commonly seen in the diabetic population. These three types can be further classified as acute or chronic. Chronic infections typically involve ischemic or necrotic bone and follow a refractory course. While this classification system is still used today, it is based only on the etiology and makes no differentiations as to the extent of the disease, nor does it offer any guidelines on treatment.
In 1985, Cierny and Mader (7) introduced a clinical staging system for adult osteomyelitis based upon four anatomic types of bone infection (types 1 to 4) and three physiologic classes of the host (A, B, and C). They did not make a distinction between acute and chronic processes, as areas of necrosis must be removed irrespective of acuity or chronicity. Using these four anatomic types and three physiologic classes allows the clinician to define 12 different clinical stages (Table 86.1).
TABLE 86.1 Cierny and Mader Classification | ||||||||||||||||||||||
---|---|---|---|---|---|---|---|---|---|---|---|---|---|---|---|---|---|---|---|---|---|---|
|
Stage I is medullary osteomyelitis. The primary lesion involves only the medullary canal, as seen with early hematogenous osteomyelitis. Pediatric patients can usually be treated with antibiotics alone, while adults frequently require cortical unroofing and intramedullary reaming. In addition to hematogenous osteomyelitis, another commonly cited example of stage I is an infected intramedullary rod in an otherwise stable bone. In this example, the infected rod must usually be removed and the medullary canal reamed. Antibiotic therapy is then directed against the cultured pathogens.
Stage II is superficial osteomyelitis. This is a true contiguous focus lesion. The infection involves only the outer cortex of exposed cortical bone at the base of a soft tissue wound. When treated early in the acute stage, stage II lesions may respond to local wound care and antibiotics. In those instances when clinical, laboratory, or radiographic findings suggest that local wound care is not sufficiently treating the infection, more aggressive treatment may be indicated.
Stage III is localized osteomyelitis. It is defined as full-thickness, cortical sequestration and/or cavitation, that is, it involves at least one cortex and extends into the medullary canal. It frequently has the combined features of stages I and II and may be the result of the progression of either of these two stages. Although full thickness, it is a discrete lesion within a stable bony segment. It can be surgically removed without compromising osseous stability.
Stage IV is diffuse osteomyelitis. It is defined as a circumferential disease of bone and soft tissue. Multiple cortices as well as the medullary canal are involved. It is a through-andthrough disease process that usually requires the resection of an intercalary segment of bone to remove all the compromised tissue. Osseous instability is present either before or after extensive débridement. Stabilization is an essential component of treatment and delineates diffuse osteomyelitis from stages I to III.
The physiologic classification takes into account both the condition of the host and the relative disability caused by the disease.
A—Host is a patient with a good immune system and delivery. These patients have a normal physiologic response to infections and surgery.
TABLE 86.2 Systemic or Local Factors That Affect Immune Surveillance, Metabolism, and Local Vascularity
SYSTEMIC
Malnutrition
Renal, liver failure
Diabetes mellitus
Malignancy
Immune deficiency
Immunosuppression
Extremes of age
Chronic hypoxia
Tobacco abuse
LOCAL
Chronic lymphedema
Venous stasis
Major vessel compromise
Small vessel disease
Extensive scarring
Radiation fibrosis
Complete loss of local sensation
Arteritis
Adapted from Cierny G, Mader JT. Adult chronic osteomyelitis. Orthopedics 1984;7:1557.
B—Host is a compromised host. A compromised host is further classified as being compromised locally (BL), compromised systemically (BS), or with a combined deficiency (BLS) in wound healing (Table 86.2).
C—Host refers to a patient in whom treatment or the sequelae of treatment are more compromising to the host than the disease itself. These patients require only suppression or no treatment at all. This can be a patient who is not a surgical candidate or a patient who cannot tolerate longterm antibiotic therapy.
PATIENT RISK FACTORS
Children and the elderly are at highest risk for hematogenous osteomyelitis (3). It is primarily found in patients less than 17 years of age, with this age group accounting for 80% of cases. Long bones are predominantly affected in children, while it is more common in the vertebrae of adults. Children with sickle cell disease are particularly susceptible to hematogenous osteomyelitis. In adults, the majority of adults are over the age of 50, with the exception of intravenous drug abusers. Risk factors include well-known causes of bacteremia such as urinary tract infections, catheterizations, hemodialysis, or central lines. Hematogenous osteomyelitis is more common in males across all age groups.
Contiguous osteomyelitis is the most common presentation of osteomyelitis seen in the lower extremity. Among the frequent etiologies are postoperative infections following prosthetic joint implantation, wound dehiscence, and pin tract infections. Patients with rheumatoid arthritis and patients on systemic corticosteroids or antimetabolites for dermatologic, rheumatic, or neoplastic disease are at higher risk for postoperative infection and osteomyelitis (8,9 and 10). Fibrosis, vasculitis, leukopenia, lymphopenia, and the local
depletion of immune factors, such as complement, have been implicated in the higher infection rates noted in such patients.
depletion of immune factors, such as complement, have been implicated in the higher infection rates noted in such patients.
Implanted materials have created a unique set of risk factors. In addition to the biofilm or glycocalyx (discussed below) that can form around an implant and interfere with normal immune defense mechanisms and obstruct the delivery of antibiotics, polymethylmethacrylate (PMMA) has been shown to decrease leukocyte migration to the site of infection as well as decrease the phagocytic and killing power of PMNs (11).
In addition, the effects of bacteria and their toxins on the implanted metals or polymers must be considered. For example, infection and decreased tissue pH, along with the effects of increased enzymes are known to accelerate biocorrosion (12). Impairment of osseous vascular perfusion, as well as the implant providing a nidus for the sequestration of bacteria, must also be considered (Fig. 86.1). Reoperation through prior incisions is likewise associated with an increased risk of infection, especially when associated with questionable soft tissue changes (3).
Hematogenous osteomyelitis may develop at a previously asymptomatic site of surgery, particularly when implanted materials have been used. In such cases, consideration must be given to distant sites where hematogenous spread may predispose the implant site to infections months or even years after fixation or prosthetic device implantation. Chronic foot ulcerations are particularly ominous in patients with implants. Recurrent soft tissue infections or abscesses may cause a transient bacteremia with associated fevers, which may not be appreciated by many diabetic patients (Fig. 86.2).
The risk of infection in nonelective surgery is clearly higher than in elective surgery. The operative management of fractures and open trauma is associated with a much higher infection rate compared with elective musculoskeletal surgery. Fitzgerald et al (10) were able to isolate bacteria from the operative site in 62% of the patients treated for open fractures. Osteomyelitis complicating open fracture management is not unusual and should be considered in the perioperative management of patients undergoing open fracture reduction.
Pinckney et al (13) have demonstrated that crush injuries to the nail plates of children are frequently associated with osteomyelitis of the distal phalanx. In addition, when concurrent nail and bone surgery are performed, there is a greater likelihood of postoperative infection that may develop into osteomyelitis. It is therefore necessary to carefully monitor such patients. Similarly, a high index of suspicion is warranted in any patient with a history of a recurrent or chronic, frankly infected ingrown nail or a patient who seems to be taking an inordinate amount of time to heal after a matrixectomy. Radiographs should be considered prior to surgical intervention on a chronically infected ingrown nail and subsequent to any postoperative nail procedure that shows signs of prolonged healing or inflammation (Fig. 86.3).
Deep ulcerations secondary to diabetic neuropathy always have the potential of progressing to osteomyelitis. This is especially true with ulcers that are chronic in nature. One should always consider the possibility of osteomyelitis when an ulcer does not heal, or shows signs of delayed healing, after offloading and appropriate local wound care.
Other sources of infection that can lead to osteomyelitis are bite wounds and puncture wounds.
In summary, the management of impending or imminent osteomyelitis requires the recognition of patients at higher statistical risk, as well as an awareness of the most likely causative organisms. Close monitoring of these patients is crucial to effect early treatment and prevent progression of the disease process.
CLINICAL SIGNS AND SYMPTOMS OF OSTEOMYELITIS
Hematogenous osteomyelitis is typically associated with the abrupt onset of increasing pain and tenderness, as well as overt signs of inflammatory disease (14). Usually seen in children, the acute onset is associated with limping, guarding, fever, elevated acute phase reactants [erythrocyte sedimentation rate, C-reactive protein], and polymorphonuclear leukocytosis (7,15). Evidence of coexisting infection at a site removed from the symptomatic area may be obtained through history, physical examination, and appropriate diagnostic studies. For example, the presence of ear, dental, urinary tract, or pulmonary infection may indicate not only the source of the invading microorganism but also the likely pathogen. In the elderly, hematogenous osteomyelitis may be associated with the absence of local inflammatory signs or systemic manifestations, and the presentation may be surprisingly subtle (16). This is particularly true in patients with coexisting peripheral vascular disease or diabetes. In the presence of peripheral neuropathy, pain and tenderness may be minimal or absent.
Hematogenous osteomyelitis may be responsible for a large percentage of bone infections at the site of implanted materials and may occur at the site of a previously asymptomatic prosthesis months or years after apparently successful surgery and uneventful recovery (17). Infections that occur 24 months after surgery are believed to be of hematogenous origin (18). In such cases, increasing discomfort or local inflammatory findings may be difficult to differentiate from loosening of a prosthesis or fixation device. Pain that presents with the first steps taken after any period of inactivity or non-weight-bearing (poststatic dyskinesia) is frequently present with a loosened device (Fig. 86.4). However, discomfort that is relieved after several minutes of weight-bearing does not exclude the diagnosis of osteomyelitis (19).
An increasing volume of literature now suggests that areas in which fixation devices or prostheses have been used are susceptible to delayed or late infection of hematogenous origin (20,21 and 22). This appears to be especially applicable when PMMA has been used as a fixation or luting device. For this reason, the American Academy of Orthopaedic Surgeons currently recommends consideration of prophylactic antibiotics for all total joint replacement patients about to undergo any invasive procedure that may cause bacteremia and potential hematogenous contamination. However, there is conflicting data as to the need and efficacy of prophylactic antibiotics. The use of antibiotic prophylaxis must be weighed against potential risk factors including anaphylactic shock, toxicity, and the possibility of the selection and transmission of bacterial resistance.
The expanded use of implant arthroplasty has warranted additional staging of postoperative osteomyelitis, which can be classified as acute, delayed, or late (7,18). Acute postoperative osteomyelitis occurs within 1 month of implant arthroplasty and is usually accompanied by the classic signs and symptoms of dehiscence, drainage, pain, inflammation, and systemic sequelae (23) (Fig. 86.5). Acute presentations may be the result of direct contamination at the time of surgery, hematogenous contamination from a remote site, or contiguous spread from an infected hematoma, a stitch abscess, or cellulitis (3). The presence of soft tissue infection is usually readily apparent, and the patient is monitored appropriately for the earliest signs of osteomyelitis. Approximately 40% of cases of acute postoperative osteomyelitis are associated with implant arthroplasty, and in these cases, the typical signs and symptoms are easily recognized.
In the majority of cases of deep infection and osteomyelitis occurring after implant surgery, the infectious process is classified as delayed or late (21,24). Delayed implant wound infection occurs between 1 month and 2 years after surgery, whereas late infection occurs 2 years or more after surgery (16). As noted earlier, delayed or late osteomyelitis after surgery may be of hematogenous origin. The seeding of bacteria at the site of previous surgery may be the result of persistent vascular response to surgical monomer or the mechanical effects of implants on bone. Some implanted materials create a chronic local subclinical inflammatory response that depletes local, humoral, and cellular defenses to otherwise controllable bacteria (11,25). The development of radiolucency at the bone-cement interface may be suggestive of infection but is not pathognomonic. Radiolucency at the bone-cement interface may be caused by infection, loosening, reaction to monomer, or a normal fibrous tissue response (26).
It has also been suggested that a delayed or late infection may be caused by microorganisms that were introduced into the wound at the time of the original surgery (27). Why bacteria fail to initiate wound infection for 2 years or longer has been the subject of speculation. Low organism virulence, or the decreased toxicity of their products, has been suggested. Another theory is that local wound conditions deteriorate over time so that optimal conditions eventually evolve for the growth of previously introduced but dormant bacteria. Such phenomena could occur when dead or dying osteoblasts release viable Staphylococcus aureus or other bacteria that can then reinfect normal osteoblasts. Another possible scenario could be the release of planktonic, that is, free-floating,
bacteria that were previously sessile and encased within the glycocalyx. Examples of wound condition deterioration over time include implant loosening, biocorrosion, or the deposition of polymer material in adjacent soft tissues. Regardless of the exact mechanism, the majority of infections that follow prosthetic implantation are delayed or late in presentation (28). The clinical similarities of osteomyelitis and corrosive metallic disease may include drainage and local inflammatory signs (12) (Fig. 86.6). Similarly, the loosening of an implant or a fixation device may be the result of osteomyelitis, but osteomyelitis may also be encouraged by loosening and local tissue damage. Delayed or late osteomyelitis may be difficult to differentiate from inflammatory arthritis, bursitis, rheumatoid nodules, tophaceous drainage, or soft tissue inflammation secondary to the physical effects of the implanted material (detritic synovitis) (29,30).
bacteria that were previously sessile and encased within the glycocalyx. Examples of wound condition deterioration over time include implant loosening, biocorrosion, or the deposition of polymer material in adjacent soft tissues. Regardless of the exact mechanism, the majority of infections that follow prosthetic implantation are delayed or late in presentation (28). The clinical similarities of osteomyelitis and corrosive metallic disease may include drainage and local inflammatory signs (12) (Fig. 86.6). Similarly, the loosening of an implant or a fixation device may be the result of osteomyelitis, but osteomyelitis may also be encouraged by loosening and local tissue damage. Delayed or late osteomyelitis may be difficult to differentiate from inflammatory arthritis, bursitis, rheumatoid nodules, tophaceous drainage, or soft tissue inflammation secondary to the physical effects of the implanted material (detritic synovitis) (29,30).
Acute osteomyelitis after surgery is usually associated with S. aureus or other gram-positive cocci, although concurrent or preexisting conditions alter the probability of discovering various pathogens (10). Late postoperative osteomyelitis, or osteomyelitis associated with a draining sinus tract, is more frequently associated with gram-negative or polymicrobic etiologic agents (15).
Contiguous osteomyelitis of the foot is most often encountered in patients with chronic ulcerations, previous lacerations, puncture wounds, or open trauma or after surgical procedures (15,31) (Fig. 86.7). Sinus tract formation and drainage are often present. In such cases, soft tissue infections are managed
by careful evaluation of the underlying bone for the earliest indication of contamination. Grayson et al (31) described probing the wounds of hospitalized diabetic patients with foot infections with a sterile blunt metal probe. In his study, the probe to bone (PTB) test had a positive predictive value of 89%. From these findings, he concluded that a positive PTB test usually obviated the need for imaging studies to confirm the diagnosis of osteomyelitis. A recent study by Lavery et al (32) found a lower positive predictive value when examining nonhospitalized diabetic patients seen in outpatient clinics. He concluded that in the typical clinical setting the PTB test had a relatively low positive predictive value, but a negative test may exclude the diagnosis of osteomyelitis.
by careful evaluation of the underlying bone for the earliest indication of contamination. Grayson et al (31) described probing the wounds of hospitalized diabetic patients with foot infections with a sterile blunt metal probe. In his study, the probe to bone (PTB) test had a positive predictive value of 89%. From these findings, he concluded that a positive PTB test usually obviated the need for imaging studies to confirm the diagnosis of osteomyelitis. A recent study by Lavery et al (32) found a lower positive predictive value when examining nonhospitalized diabetic patients seen in outpatient clinics. He concluded that in the typical clinical setting the PTB test had a relatively low positive predictive value, but a negative test may exclude the diagnosis of osteomyelitis.
DIAGNOSTIC IMAGING IN OSTEOMYELITIS
While there have been major advancements in the techniques used to diagnose and stage osteomyelitis, plain film radiographs remain the initial step in diagnostic imaging. Standard radiographs are readily available in almost all settings, are inexpensive, can be interpreted quickly, and if positive may obviate the need for further imaging studies. They do have significant drawbacks however. The earliest appearance of osteomyelitis is not apparent until approximately 50% of the bone mineral is lost. This results in a significant lag time of a minimum of 10 to 14 days before the disease can be visualized. As a result, sole reliance on plain radiographs may delay therapy while the infectious process progresses and destroys more bone. Therefore, while the role of standard radiographs is significant, the diagnosis of osteomyelitis is augmented by a high index of suspicion, clinical observations, aspiration, or bone biopsy.
The classic changes seen on plain film radiographs are well known and include soft tissue swelling, resorptive bone changes, periosteal reaction and bone destruction, sequestrum, involucrum, and cloaca formation (3). Sequestrum refers to a segment of necrotic bone that is separated by granulation tissue from surrounding live bone. Involucrum is new bone that has formed around dead bone. Involucrum can become perforated by tracts, and openings in the involucrum are referred to as cloaca. When these tracts extend to the skin, they are referred to as sinuses. A Brodie abscess is a localized, well-demarcated focus of infection lined by granulation tissue that is not associated with systemic illness (Fig. 86.8). Many of these changes however are also seen in other disease processes. Periosteal reaction may be seen in a variety of conditions including soft tissue infection in the absence of actual bone suppuration (osteolysis) (33,34 and 35) (Fig. 86.9). Resorptive or frankly destructive changes may be noted in inflammatory or crystalline arthropathies and neurotrophic arthropathies. In addition, changes consistent with osteomyelitis can be seen with polymer implants, particularly those of the first metatarsophalangeal joint where resorptive changes can occur over time at the implant-bone interface (Fig. 86.10). Sclerosis, osteopenia, or fragmentation may occur with implant failure, implant loosening, or metallic corrosion. Similarly, atrophic and hypertrophic nonunion, avascular necrosis, and osteomyelitis may be difficult to differentiate by radiographic evaluation early in the course of the disease (36) (Fig. 86.11).
In the diabetic patient with an ulceration or deep infection, the changes visualized on radiographic evaluation may be associated with nonsuppurative conditions such as diabetic osteolysis, spontaneous fracture, reactive periostitis, or neuropathic joint disease (34,35). Because the radiographic changes of each of these disorders may be strikingly similar to those of osteomyelitis, the superimposition of osteomyelitis may be difficult to ascertain. If the clinical index of suspicion is high, treatment is often initiated even in the presence of a paucity of radiographic changes to minimize the extent of damage and prevent progression of the infection. A second set of x-rays can then be obtained 2 to 3 weeks later to see if there is any change in radiographic findings. When plain film findings are equivocal, a variety of other diagnostic techniques may be employed to assist in establishing the presence and extent of osteomyelitis.
COMPUTED TOMOGRAPHY
Computed tomography scanning has been employed in the evaluation of osteomyelitis, but its role is less clearly defined than that of MRI. CT scanning can provide valuable anatomic detail that is not available with standard radiographic evaluation, particularly in the presence of complex fractures or fusion in the midfoot or rearfoot. With an appropriate bone or soft tissue window, medullary gas and early subperiosteal, medullary, or endosteal changes may be visualized because the elevation of attenuated values in the marrow space is an early sign of acute osteomyelitis. Trabecular bone mineralization changes may be detected with great accuracy by high-resolution CT scanners. These changes, although indicative of cortical bone destruction and new bone formation, are not specific because aseptic
necrosis, osteoporosis, arthrosis, and osteomalacia appear similar. Soft tissue techniques will allow for exceptional definition of the presence and extent of deep abscesses and periosteal reactivity, and they are particularly useful in the evaluation of deep plantar structures, although MRI is clearly superior in this regard (Fig. 86.12).
necrosis, osteoporosis, arthrosis, and osteomalacia appear similar. Soft tissue techniques will allow for exceptional definition of the presence and extent of deep abscesses and periosteal reactivity, and they are particularly useful in the evaluation of deep plantar structures, although MRI is clearly superior in this regard (Fig. 86.12).
MAGNETIC RESONANCE IMAGING
Magnetic resonance imaging has for years been considered by many to be the gold standard for imaging osteomyelitis, although it does have its drawbacks and may someday be supplanted by emerging radionuclide imaging techniques.
MRIs have the potential to reveal the definition, location, and extent of soft tissue and bone infection. This information can be invaluable as a preoperative guide for incision placement and give an idea of the amount of bone that might need to be débrided or resected.
MRI can reveal changes in the bone marrow very early in the course of the disease. As the normally fatty tissue in the marrow is replaced by inflammatory fluid, this will result in low signal intensity on T1-weighted images and high signal intensity on T2-weighted and fat suppression techniques such as STIR (short T1 inversion recovery) or fat saturation sequences.
MRI is not without its drawbacks, however. Many other conditions can mimic the findings of osteomyelitis. These include neuropathic joint changes, occult fractures, healing fractures and osteotomies, neoplasms, and acute gouty arthritis.
Furthermore, any metallic implants in the area will produce imaging artifacts. Titanium implants are somewhat less problematic in this regard than stainless steel.
Lastly, the presence of pacemakers and cardioverter defibrillators are relative contraindications for MRI.
NUCLEAR MEDICINE IMAGING
The above-mentioned imaging techniques all rely upon their abilities to detect changes in the anatomy or structure of the bones being examined. Nuclear medicine imaging is different in that it relies on the detection of changes in the physiology or
function of the bone being examined. Nuclear medicine imaging, also known as radionuclide imaging or nuclear scintigraphy, involves the intravenous injection of a radioactive isotope. This isotope is often bound to a molecule or cell type and will localize in target tissues. Gamma cameras subsequently detect the radiation emitted by these isotopes and an image is then created from this data.
function of the bone being examined. Nuclear medicine imaging, also known as radionuclide imaging or nuclear scintigraphy, involves the intravenous injection of a radioactive isotope. This isotope is often bound to a molecule or cell type and will localize in target tissues. Gamma cameras subsequently detect the radiation emitted by these isotopes and an image is then created from this data.
The term “three-phase bone scan” refers to the use of technetium 99m methylene diphosphonate (99mTc MDP). The isotope technetium 99m is bound to methylene diphosphonate, which demonstrates absorption onto the surface of the hydroxyapatite crystals found in bone matrix. 99mTc MDP will therefore accumulate in any areas of bone metabolic activity such as increased periosteal, endosteal, or osseous vascularity and physiologic activity (37). Normal uptake is seen at areas of tendon insertion, stress, osseous remodeling, and the epiphyseal plates of children.
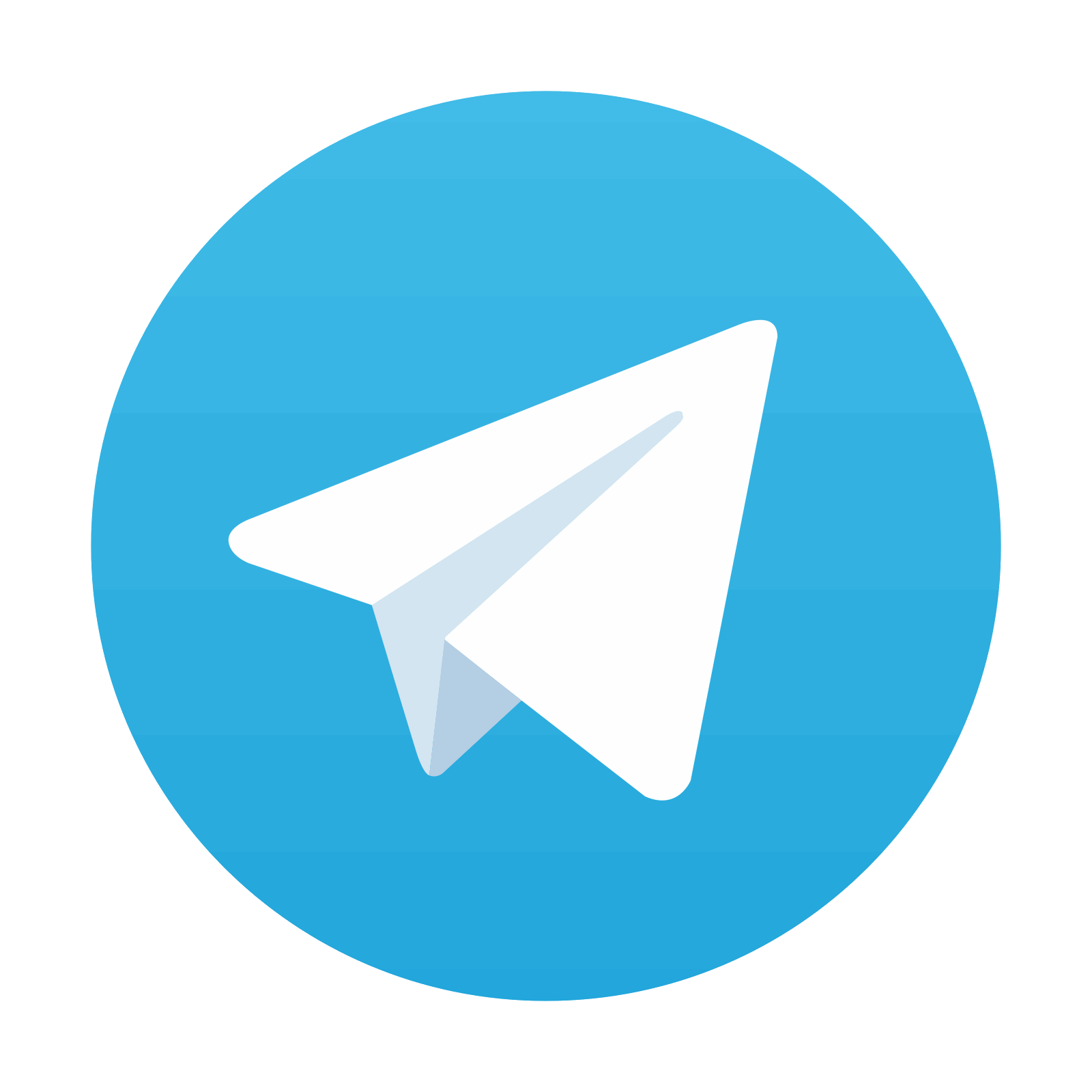
Stay updated, free articles. Join our Telegram channel

Full access? Get Clinical Tree
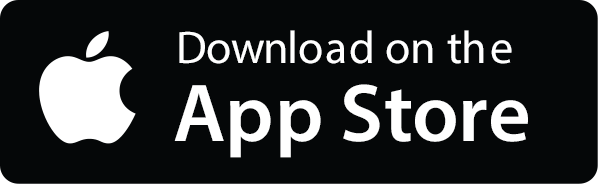
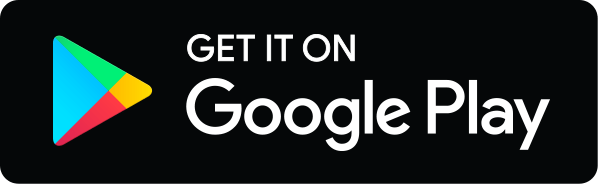