Orthoses in Orthopedic Care and Trauma
Michelle M. Lusardi, William J. Barringer, Melvin L. Stills, Joshua L. Carter and Mark Charlson
Learning Objectives
On completion of this chapter, the reader will be able to:
1. Describe the most common musculoskeletal injuries that occur at various points in the life span.
2. Describe orthotic intervention for congenital and growth-related musculoskeletal impairments.
Orthoses play a significant role in orthopedic and rehabilitative care of individuals with many different types of musculoskeletal pathologies and impairments. Dysfunction of the musculoskeletal system can be the result of congenital or developmental disorders or can be acquired as a result of overuse injury, systemic disease, infection, neoplasm, or trauma at any point in the life span. This chapter focuses on the use of orthoses to manage congenital and developmental musculoskeletal problems in children and fractures of long bones of the lower extremity.
The understanding of how orthoses are helpful in the care of those with musculoskeletal impairments is founded on knowledge of the development and physiology of musculoskeletal tissues (bone, cartilage, ligaments, menisci, muscles, and their tendons or aponeuroses); the kinesiological relationships among these tissues; and an understanding of how these tissues remodel in response to physical stressors (forces).1,2 This chapter begins with an overview of the anatomy of bone, its growth and remodeling, and the principles behind the rehabilitation (examination and intervention) of persons with disorders of bone. Then the authors look specifically at disorders of the hip joint and orthotic/orthopedic strategies for limb fractures.
Bone structure and function
In anatomy classes and texts, students learn that the mature adult human skeleton is composed of 206 bones (Figure 12-1), ranging from the long bones of the extremities, the blocklike vertebrae of the spine, the encasing protective ribs and skull, and the multiarticulating carpals and tarsals of the wrist and ankles that enable positioning of the hands and feet for functional activities.3,4 Bony prominences formed during development from the force of muscle contraction at tendinous origins and insertions are identified.1 Students scrutinize articular surfaces to understand how joints move, consider the hyaline cartilage that protects the joint from repeated loading and shear during activity, and learn to examine the ligaments that maintain alignment for normal joint function. From a skeletal model or examination of bone specimens in anatomy laboratory, it is not intuitively apparent that living bone is a dynamic and metabolically active tissue serving multiple purposes and physiological roles.5 These include storage and homeostasis of calcium, phosphate, magnesium, sodium, and carbonate (via ongoing osteoblastic and osteoclastic activity in conjunction with kidney function); production of erythrocytes, granular leukocytes, and platelets in the marrow; physical growth and development (by responsiveness to the pituitary’s hormones at the epiphyseal plate); provision of a protective and functional frame for the organs of the thorax and abdomen (how would people breathe without ribs?); and body weight support when the body is either at rest or in motion during functional activities.6
Bone is a dense, regular, connective tissue derived from embryonic mesoderm. It contains a combination of specialized cells (osteoblasts, osteocytes, osteoclasts) embedded in a matrix of minerals (70%), protein (22%), and water (8%). The many bones of the human body can be described as long or short tubular bone (e.g., the femur, tibia, metatarsals, phalanges), flat bone (e.g., the pelvis or skull), irregular bone (e.g., the tarsals and carpals), sesamoid bone embedded within tendons (e.g., patella), or accessory bones (e.g., ossicles of the middle ear). Alternatively, they can be classified as primarily cortical (dense) or cancellous (trabecular) bone on the basis of the density and arrangement of their components. Long bones are subdivided into regions, each of which has its own blood supply (Figure 12-2). The diaphysis (shaft) is supplied by one or more nutrient arteries that penetrate the layers of the bony cortex, dividing into central longitudinal arteries within the marrow cavity. The flared metaphyses serve as an area of transition from cortical to cancellous bone and are supplied by separate metaphysical arterioles. The epiphyses are metabolically active areas of cancellous bone with supportive trabeculae, with an extensive capillary network derived from epiphyseal arteries. The epiphysis is actively remodeled over the life span in response to weight bearing and muscle contraction during activity.7 In childhood and adolescence, before skeletal maturation, the bony metaphyses and epiphyses are connected by cartilaginous epiphyseal (growth) plate, which calcifies and fuses throughout various periods of development. The periosteum is a layer of less dense, vascularized connective tissue that overlies and protects the external surface of all bone and houses osteoblastic cells necessary for bone deposition and growth. The periosteum is replaced by articular (hyaline) cartilage within the joint capsule. The endosteum, a thin connective tissue lining of the marrow cavity of long bones and the internal spaces of cancellous bone of the marrow space, also houses osteoblasts. Both linings are active as part of osteogenesis during growth and fracture healing.
Cortical bone is the most highly mineralized type of bone found in the shafts (diaphyses) of the long bones of the body and serves as the outer protective layer of the metaphysis and epiphysis of tubular bone, as well as the external layers of flat, irregular, and sesamoid bones. Most of the bones in the human skeleton (80% to 85%) are primarily cortical with cancellous/trabecular bone in the metaphyseal and epiphyseal region. Cross section of a long tubular bone reveals three layers of cortical bone: the inner or endosteal region next to the marrow cavity, the metabolic intracortical or haversian region with its haversian canals surrounded by concentric layered rings (osteons) and Volkmann’s canals containing a perpendicularly arranged anastomosing capillary network, and the dense outer periosteal region (Figure 12-3).
Cancellous (trabecular) bone with its honeycomb or spongy appearance is much more metabolically active and much less mineralized than cortical bone. Cancellous bone is composed of branching bony spicules (trabeculae) arranged in interconnecting lamellae to form a framework for weight bearing (Figure 12-4A). In the vertebral bodies, for example, trabeculae are arranged in an interconnecting horizontal and vertical network oriented perpendicular to the lines of weight-bearing stress into a boxlike shape (see Figure 12-4B). In contrast, trabeculae in the proximal femur form an archlike structure to support weight-bearing forces between the hip joint and femoral shaft (see Figure 12-4C). In living bone, cavities between trabeculae are filled with bone marrow.
Three types of cells are embedded within the various compartments of bone. Osteoblasts, bone building cells, synthesize and secrete the organic matrix of bone (osteoid) that mineralizes as bone matures. They are located under periosteum and endosteum and are active in times of bone growth and repair. Osteocytes are matured and inactive osteoblasts that have become embedded within bone matrix. Osteocytes remain connected to active osteoblasts via long dendritic processes running through the canaliculi (small channels) within the bone matrix. Both osteoblasts and osteocytes are responsive to circulating growth hormones, growth factors, and cytokines, as well as mechanical stressors and fluid flow within the bone itself.8,9 Osteocytes are thought to be involved in mineral exchange, detection of strain and fatigue, and control of mechanically induced remodeling.10 Osteoclasts, derived from precursor cells in bone marrow, are macrophage-like cells that can move throughout bone to resorb bone by releasing minerals from the matrix and removing damaged organic components of bone.11,12
The epiphyses of long bones, the vertebrae, and large flat bones house nociceptors and mechanoreceptors and a network of afferent sensory neurons that contribute to exteroceptive (primarily pain) pathways.13 The periosteum has a particularly rich neural network with branches that continue along with penetrating nutrient arteries into the haversian canals into the diaphysis.
Bone growth and remodeling over the life span
During childhood and adolescence, growth occurs through the process of modeling, in which bones increase in length and diameter and are reshaped until the epiphyseal plates calcify and skeletal maturity is achieved (typically in mid-adolescence for females and early adulthood for males).14 In adulthood, bone health is maintained by the ongoing process of remodeling, in which there is a balance and coupling between osteoblastic deposition of new bone substance and osteoclastic resorption of existing bone.15 This ongoing process of turnover means that the internal architecture of living bone is actively restructured and replaced at a rate of approximately 5% per year in cortical bone and up to 20% per year in cancellous bone.5 The rate of bone formation, resorption, and turnover is influenced by both systemic hormones and other substances (e.g., parathyroid hormone, calcitonin, vitamin D from the kidney, growth hormone, adrenocorticosteroids, estrogen, progesterone, androgens); local cell-derived growth factors; and availability of essential nutrients (calcium, fluoride, vitamin A, vitamin D, and vitamin E).16–18 These substances, along with blood or urinary levels of certain enzymes active during turnover and metabolites of bone resorption, are monitored as biomarkers to track progression of bone diseases associated with high rates of bone resorption (e.g., Paget’s disease, osteoporosis, hyperparathyroidism) and to determine efficacy of medical-pharmaceutical interventions for these diseases.19–21
In the prenatal period and in infancy, the flat bones of the skull develop as the fetal mesenchyme that forms as the periosteum begins to ossify (intramembranous ossification). Most long bones, as well as the vertebrae and pelvis, develop from a cartilaginous framework or template. In this process of endochondral bone formation, cartilage cells mature and eventually ossify.7 During childhood and adolescence, bones grow in both length and diameter and are dynamically modeled toward their mature configurations.22 During puberty, accumulation of bone mass accelerates; by the end of puberty, as much as 90% of mature bone mass is established.23 For young children with abnormal skeletal development, orthoses attempt to capitalize on the dynamic modeling process, applying external forces to influence bone shape and length.24 For approximately 15 years following puberty, after closure of the epiphyseal plates of the long bones, bone mass continues to increase—a process described as consolidation.22 Gender differences in peak bone mass have been well documented: Average peak bone mass in women is approximately 20% less than that of men. Early in the midlife period, both men and women enter a period of gradual endochondral bone loss that appears to be genetically determined; the rate of bone loss is also influenced by hormonal status, nutrition, smoking and alcohol use, and activity level.25–28 It is estimated that males will lose between 15% and 40% of cancellous bone mass and 5% to 15% of cortical bone mass over their lifetime. In women, menopause accelerates the rate of bone loss; there may be up to a 50% decrease from peak cancellous bone mass and 30% decrease in cortical bone mass over their lifetimes.29 Significant loss of bone mass is associated with increasing vulnerability to fracture, especially among postmenopausal women.
Orthoses in the management of hip dysfunction
Hip orthoses are important in the management of hip disorders in infants and children, as well as in the postsurgical care of children and adults. An understanding of the designs of and indications for various hip orthoses is essential for physicians and rehabilitation professionals working with individuals who have orthopedic problems of the pelvis, hip joint, or proximal femur. For children with developmental dysplasia of the hip (DDH) or Legg-Calvé-Perthes disease (LCPD), hip orthoses are the primary intervention for prevention of future deformity and disability. Hip orthoses are essential elements of postoperative care and rehabilitation programs for children with musculoskeletal and neuromuscular conditions who have had surgical intervention for bony deformity or soft tissue contracture. Hip orthoses can also be major postoperative interventions for adults who have had repair of a traumatic injury or a complex total hip arthroplasty. The efficacy of orthotic intervention is influenced by patient and caregiver adherence: The key to successful use of these orthoses is clear, and open communication exists among the physician, therapist, orthotist, and family concerning the primary goals of the orthosis, its proper application and wearing schedule, and the possible difficulties that may be encountered. Positive health care outcomes and happy patients and families are contingent on the ability of the health care team to communicate.
When are Hip Orthosis Indicated?
Most nontraumatic hip joint dysfunction or pathology occurs either in childhood or in late adult life and is frequently related to one or more of the four following factors:
1. Inadequate or ineffective development of the acetabulum and head of the femur in infancy
2. Avascular necrosis of the femoral head associated with inadequate blood supply during childhood
3. Loss of cartilage and abnormal bone deposition associated with osteoarthritis
Orthotic intervention is an important component in the orthopedic management of many of these conditions. Most often, hip orthoses are used to protect or position the hip joint by limiting motion within a desirable range of flexion/extension and abduction/adduction. It is important to note that hip orthoses alone are not effective in controlling internal/external rotation of the hip joint. If precise rotational control is desired, a hip-knee-ankle-foot orthosis (HKAFO) must be used.
Hip Structure and Function
The hip (coxofemoral) joint is a synovial joint formed by the concave socketlike acetabulum of the pelvis and the rounded ball-like head of the femur (Figure 12-5). Because of the unique bony structure of the hip joint, movement is possible in all three planes of motion: flexion/extension in the sagittal plane, abduction/adduction in the frontal plane, and internal/external rotation in the transverse plane. Most functional activities blend movement of the femur on the pelvis (or of the pelvis on the femur) across all three planes of motion.
The hip joint has two important functions. First, it must support the weight of the head, arms, and trunk during functional activities (e.g., erect sitting and standing, walking, running, stair climbing, transitional movements in activities of daily living). Second, it must effectively transmit forces from the pelvis to the lower extremities during quiet standing, gait, and other closed chain activities.30
The acetabulum is formed at the convergence of the pubis, ischium, and ilium. Its primary orientation is in the vertical, facing laterally, but it also has a slight inferior inclination and an anteverted, or anterior-facing tilt. Developmentally, the depth of the acetabulum is dynamically shaped by motion of the head of the femur during leg movement and weight bearing. The acetabulum is not fully ossified until late adolescence or early young adulthood. The articular surface of the acetabulum is a horseshoe-shaped, hyaline cartilage–covered area around its anterior, superior, and posterior edges. A space along the inferior edge, called the acetabular notch, is nonarticular, has no cartilage covering, and is spanned by the transverse acetabular ligament. The acetabular labrum is a fibrocartilaginous ring that encircles the exterior perimeter of the acetabulum, increasing joint depth and concavity. The center of the acetabulum, the acetabular fossa, contains fibroelastic fat and the ligamentum teres, and is covered by synovial membrane.
The femoral components of the hip joint include the femoral head, the femoral neck, and the greater and lesser trochanters. The spherical articular surface of the femoral head is covered with hyaline cartilage. Because the femoral head is larger and somewhat differently shaped than the acetabulum, some portion of its articular surface is exposed in any position of the hip joint. The femur and acetabulum are most congruent when positioned in a combination of flexion, abduction, and external rotation. The proximal femur, composed primarily of trabecular bone, is designed to withstand significant loading while also permitting movement through large excursions of range of motion. The orientation of the femoral head and neck in the frontal plane, with respect to the shaft of the femur, is described as its angle of inclination (Figure 12-6).
In infancy the angle of inclination may be as much as 150 degrees but decreases during normal development to approximately 125 degrees in mid-adulthood and to 120 degrees in later life.31 The orientation of the proximal femur to the shaft and condyles in the transverse plane, called the angle of anteversion, is also a key determinant of hip joint function (Figure 12-7). Anteversion may be as much as 40 degrees at birth, decreasing during normal development to approximately 15 degrees in adulthood.31 These two angulations determine how well the femoral head is seated within the acetabulum and, in effect, the biomechanical stability of the hip joint. The functional stability of the hip joint is supported by a strong fibrous joint capsule and by the iliofemoral, ischiofemoral, and pubofemoral ligaments. Fibers of the capsule and ligaments are somewhat obliquely oriented, becoming most taut when the hip is in an extended position.
Infants and Children with Developmental Dysplasia of the Hip
DDH is the current terminology for a condition previously called congenital dislocation of the hip. This new term includes a variety of congenital hip pathologies including dysplasia, subluxation, and dislocation. This terminology is preferred because it includes those infants with normal physical examination at birth who are later found to have a subluxed or dislocated hip, in addition to those who are immediately identified as having hip pathologies.32–34
Incidence and Etiology of Developmental Dysplasia of the Hip
Instability of the hip due to DDH occurs in 11.7 of every 1000 live births, with most of these classified as hip subluxation (9.2/1000), followed by true dislocation (1.3/1000) and dislocatable hips (1.2/1000).33,35 Hip dislocation is more common in girls (70%) than in boys and among white than among black newborns. Approximately 20% of all hip dislocations are associated with breech presentation, although the incidence of breech presentation in the normal population is approximately 4%.36 A familial tendency is also found: DDH is much more likely to occur when an older sibling has had congenital subluxation or dislocation. The risk of dysplasia increases with any type of intrauterine malpositioning leading to extreme flexion and adduction at the hip. This occurs more commonly during first pregnancies if and when tightness of maternal abdominal or uterine musculature is present, when the infant is quite large, or when insufficient amniotic fluid restricts intrauterine motion.32,37 A higher incidence of DDH is also found among newborns with other musculoskeletal abnormalities including torticollis, metatarsus varus, clubfoot, or other unusual syndromes.
At birth the acetabulum is quite shallow, covering less than half of the femoral head. In addition, the joint capsule is loose and elastic. These two factors make the neonate hip relatively unstable and susceptible to subluxation and dislocation. Normal development of the hip joint in the first year of life is a function of the stresses and strains placed on the femoral head and acetabulum during movement. In the presence of subluxation or dislocation, modeling of the acetabulum and femoral head is compromised. The most common clinical sign of DDH is limitation in hip abduction.38 On clinical examination, a “click” (Ortolani sign) felt when upward pressure is applied at the level of the greater trochanter on the newborn or infant’s flexed and abducted hip (Figure 12-8) indicates that a dislocated hip has been manually reduced.39 The goal of orthotic management in developmental dysplasia is to achieve optimal seating of the femoral head within the acetabulum while permitting the kicking movements that assist shaping of the acetabulum and femoral head for stability of the hip joint.40,41 This is best achieved if the child is routinely positioned in flexion and abduction at the hip. If DDH is recognized early and appropriate intervention is initiated, the hip joint is likely to develop normally. If unrecognized and untreated, DDH often leads to significant deformity of the hip as the child grows, resulting in compromised mobility and other functional limitations.
Early Orthotic Management of Developmental Dysplasia of the Hip: Birth to 6 Months
In 1958 Professor Arnold Pavlik of Czechoslovakia described an orthosis for the treatment of dysplasia, subluxation, and dislocation of the hip.42,43 The orthosis he developed, the Pavlik harness, relies on hip flexion and abduction to stabilize the hip at risk. Although a multitude of braces and orthoses have been used historically in the treatment of hip instability, including hip spica casts, the Frejka pillow, the Craig splint, the Ilfeld splint, and the von Rosen splint, the Pavik harness, has become widely accepted as a mainstay for the initial treatment for the unstable hip in neonates from birth to 6 months of age.
At first glance, the Pavlik harness seems a confusing collection of webbing, hook-and-loop material, padding, and straps. In reality, this dynamic orthosis (Figure 12-9) has three major components:
1. A shoulder and chest harness that provides a proximal anchor for the device
2. A pair of booties and stirrups used as the distal attachment
The anterior strap allows flexion but limits extension, whereas the posterior strap allows abduction but limits adduction. The child is free to move into flexion and abduction, the motions that are most likely to assist functional shaping of the acetabulum in the months after birth.42–44 To be effective, however, the fit of the harness must be accurately adjusted for the growing infant and the orthosis must be properly applied. The family caregiver must be involved in an intensive education program when the newborn is being fit with the Pavlik harness. Nurses, physical and occupational therapists, pediatricians, and orthopedic residents who work with newborns also need to understand the function and fit of this important orthosis. The guidelines for properly fitting a Pavlik harness include the following key points40,45:
2. The chest strap is fit around the thorax at the infant’s nipple line.
3. The proximal calf strap on the bootie is fit just distal to the knee joint.
4. The anterior leg straps are attached to the chest strap at the anterior axillary line.
5. The posterior leg straps are attached to the chest strap just over the infant’s scapulae.
In a correctly fit orthosis, the lower extremity is positioned in 100 to 120 degrees of hip flexion, as indicated by the physician’s evaluation and recommendation. The limbs are also positioned in 30 to 40 degrees of hip abduction. The distance between the infant’s thighs (when the hips are moved passively into adduction) should be no more than 8 to 10 cm. In a well-fit orthosis, extension and adduction are limited, whereas flexion and abduction are freely permitted: The infant is able to kick actively within this restricted range while wearing the orthosis. This position and movement encourage elongation of adductor contractures, which in turn assists in the reduction of the hip and enhances acetabular development. Three common problems indicate that the fit of the harness requires adjustment40,46:
Optimal outcomes in infants with DDH are associated with early aggressive intervention of the unstable hip using the Pavlik harness.47,48 Families and health care professionals must seek proper orthopedic care to avoid misdiagnosis and mistreatment. One of the most common misdiagnoses is mistaking dislocation for subluxation and implementing a triple- or double-diapering strategy for intervention. Although this strategy does position the infant’s hip in some degree of flexion and abduction, bulky diapers alone are insufficient for reducing dislocation.
Initially, most infants wear the Pavlik harness 24 hours a day. The parents can be permitted to remove the harness for bathing, at the discretion of the orthopedist. Importantly, especially early in treatment, the fit and function of the orthosis must be reevaluated frequently to ensure proper position in the orthosis. The many straps of the Pavlik harness can be confusing to the most caring of families. The proper donning and doffing sequence should be thoroughly explained and demonstrated to the family. Additional strategies to enhance optimal reduction of the hip such as prone sleeping should be encouraged.
Families must be instructed in proper skin care and in bathing the newborn or infant wearing the orthosis. Initially, they may be advised to use diapers, but not any type of shirt, under the orthosis. The importance of keeping regularly scheduled recheck appointments for effective monitoring of hip position and refitting of the orthosis as the infant grows cannot be overstressed to the parents or caregivers.48–50 Missed appointments often result in less than optimal positioning of the femoral head with respect to the acetabulum, a less than satisfactory outcome of early intervention, and the necessity of more invasive treatment procedures as the child grows.
As a general rule, the length of treatment in the harness is equal to the child’s age when a stable hip reduction is achieved plus an additional three months. Thus if a stable reduction is achieved at 4 months of age, the total treatment time would be 7 months. Over time, when hip development is progressing as desired, the wearing schedule can be decreased to night and naptime wear. This often welcomed change in wearing time can begin as early as 3 months of age if x-ray, ultrasound, and physical examination demonstrate the desired bone development. When the orthopedist determines that the hip is normal according to radiographs and ultrasound and is satisfied with the clinical examination, the orthosis can be discontinued. If development of the hip is slow or the infant undergoes rapid growth, it may be advisable to continue the treatment with another type of hip abduction orthosis designed for older and larger babies, to maintain the position of flexion and abduction for a longer period of time.
Management of Developmental Dysplasia of the Hip: Months and Beyond
For older infants and toddlers (6 to 18 months) whose DDH was unrecognized or inadequately managed early in infancy, intervention is often much more aggressive and may include an abduction brace, traction, open or closed reduction, and hip spica casting.33,51 For infants who are growing quickly or whose bone development has been slow, an alternative to the Pavlik harness is necessary. After the age of 6 months, especially as the infant begins to pull into standing in preparation for walking, the Pavlik harness can no longer provide the desired positioning for reduction. Often, the infant is simply too large to fit into the harness. By this time, families who have been compliant with harness application and wearing have grown to dislike it and are ready for other forms of intervention.
A custom-fit prefabricated thermoplastic hip abduction orthosis is often the next step in orthotic management of DDH. This orthosis consists of a plastic frame with waist section and thigh cuffs, waterproof foam liner, and hook-and-loop material closures. The static version is fixed at 90 degrees of hip flexion and 120 degrees of hip abduction (Figure 12-10). An adjustable joint can be incorporated into the abduction bar; however, hip flexion is maintained at 90 degrees. This orthosis appears to be static, but the child is able to move within the thigh sections while the safe zone for continued management of hip position is maintained.
Many families view the hip abduction orthosis as an improvement over the Pavlik harness: The caregivers and the infant are free from cumbersome straps, the orthosis is easily removed and reapplied for diaper changing and hygiene, and the orthosis itself is waterproof and easier to keep clean. Parents and caregivers can hold the infant without struggling with straps, and the baby is able to sit comfortably for feeding and play.
Because most hip abduction orthoses are prefabricated, the knowledge and skills of an orthotist are necessary to ensure a proper custom fit for each child. To determine what the necessary modifications are, the orthotist evaluates three areas:
Modifications may require reheating or trimming of the plastic or foam padding. Usually this fitting takes place in the orthotist’s office or the clinic setting, where the necessary tools are readily available. Once the fit is evaluated and modified as appropriate for the individual child, the parents or caregivers are instructed in proper donning/doffing and orthotic care.
The static hip abduction orthosis is used in either of two ways. First, the orthosis may be a continuation of the course of treatment established by the Pavlik harness, as determined by the orthopedist’s evaluation of the child’s hip. As a continuation of treatment, the orthosis can be worn day and night; most often, however, it is reserved for nighttime use while the child is sleeping.38,40,51 The use of the orthosis at night is believed to assist development of acetabular growth cartilage. If the orthosis is worn consistently for several months and evidence of effective reduction and reshaping of the joint is present, it is less likely that more aggressive forms of treatment will be necessary as the child grows.
The second application for the hip abduction orthosis is for follow-up management for children with DDH who require an orthopedic intervention such as traction, surgical reduction, or casting. In this case the orthosis provides external stability to the hip during the postoperative weeks and months, while the baby regains range of motion and continues to grow and progress through the stages of motor development. This extra stability reduces parental and physician concern about dislocation and other undesired outcomes of the orthopedic procedure.
The static hip abduction orthosis has obvious advantages over plaster or synthetic hip spica casts including greater ease in diaper hygiene and bathing and is often welcomed by families as a positive next step in treatment. Fitting requires the knowledge and skills of an orthotist familiar with proper fitting techniques and who can manage potentially irritable babies just freed from a confining hip spica cast.
Goals of Orthotic Intervention for Children with Developmental Dysplasia of the Hip
To be effective, orthotic intervention for DDH must have a set of clearly described treatment objectives against which success can be measured. The components necessary for effective orthotic interventions for children with DDH include the following:
The ultimate goal is to facilitate normal development of the hip joint, providing the child with a pain-free, stable, functional hip that will last throughout his or her lifetime.
Complications of Orthotic Management of Developmental Dysplasia of the Hip
In most cases the Pavlik harness, perhaps followed by abduction splint use as the child grows, is a successful intervention for DDH. A small percentage of infants with DDH managed by the Pavlik harness (<8%) develop complications, the most serious of which is avascular necrosis of the femoral head, which may be caused by overtightening the posterior straps to force a position of abduction.33,49–54 Children who have complications differ from those without complications in a number of ways. They tend to have larger acetabular angles (>35 degrees) and less total coverage of the femoral head within the acetabulum (<20%) on radiography or ultrasound, have an irreducible dislocation at initiation of orthotic intervention, have delayed diagnosis and intervention (older than 3 months of age), and have not demonstrated a prior ossific nucleus on radiograph or ultrasound.49,50,52,55
Orthotic Management of Legg-Calvé-Perthes Disease
LCPD is a hip pathology that affects otherwise healthy school-aged children. Although the clinical signs and symptoms of LCPD, as they differ from tuberculosis involving the hip, were first described by Arthur T. Legg in 1910,56 the etiology of this condition is not clearly understood and its treatment and orthopedic management continue to be controversial. The hallmark of LCPD is a flattening of the femoral head, thought to be a result of an avascular necrosis insult. Left untreated, LCPD leads to permanent deformity and eventual osteoarthritis in the adult hip. The disease is four times as common in boys between the ages of 4 and 8 years old as in girls, although outcomes in girls tend to be less satisfactory.57–59 LCPD generally involves only one hip; only approximately 12% of cases are bilateral. It is rare within the black population. Several options for orthotic management of LCPD have evolved. All are designed to help maintain a spherical femoral head and normal acetabulum.
Etiology of Legg-Calvé-Perthes Disease
The etiology of LCPD remains controversial more than 100 years after it was first described. Most researchers believe that LCPD is a result of some event or condition that compromises blood flow to the femoral head and leads to avascular necrosis. The exact mechanism that triggers this compromise is unknown. Some theories focus on an acute trauma that damages the vascular system of the femoral head, whereas others suggest that repeated episodes of a transient synovitis may compromise blood flow.58,60,61 Another theory suggests an abnormality of thrombolysis in children who develop LCPD.62 A genetic predisposition to delayed bone age that exposes vessels to high rates of compression as they pass through cartilage to the bony head has also been suggested.60 Although the exact etiology of LCPD remains a mystery, it is certainly linked to episodes of avascular necrosis in the femoral head. The goal of intervention in children with LCPD is to assist revascularization of the femoral head and to restore normal anatomical shape and alignment of the hip joint.
Evaluation and Intervention for Legg-Calvé-Perthes Disease
LCPD should be suspected in children with one or more of the following signs or symptoms63,64:
1. A noticeable limp, often with a positive Trendelenburg sign
2. Pain in the hip, groin, knee, or a combination of these locations
When these symptoms are present, radiographic, ultrasound, or magnetic resonance imaging studies of the hip are used to discriminate between LCPD (Figure 12-11) and other hip disorders (e.g., slipped capital femoral epiphysis, fracture, rheumatic disease, infection).65 These studies are used by the orthopedist to determine severity and progression of the disease, considering stage of disease, shape of femoral head, degree of congruence with the acetabulum, and length and angle of the femoral neck.66,67 A variety of classification systems have been developed to rate severity of involvements. The Catterall system describes four groups on the basis of the location of involvement and identifies four “head at risk” signs for the orthopedist or radiologist to focus on in interpreting radiographs.68 Studies of the interrater and concurrent reliability of the Catterall systems have not all been positive.69–71 The Salter-Thompson system rates severity of involvement on the basis of the location and extent of subchondral fracture that may be observed early in the disease process.72,73 The Herring system examines the condition of the lateral pillar of the femoral head on radiographs.65,71,74 Refer to texts on orthopedic conditions in pediatrics for further information about classification.60,61
LCPD is a self-limiting process that often resolves in 1 to 3 years. The disease progresses through three stages:
1. Necrotic stage: avascular necrosis
2. Fragmentation stage: resorption of damaged bone
3. Healing/reparative stage: revascularization, reossification, and bony remodeling
Factors that influence the eventual outcome of the disease include age at onset, severity of damage to the femoral head and epiphysis, and quality of congruency of the acetabulum and femoral head.58–61
Because the disease process is self-limiting, the optimal intervention strategy is controversial. The three most commonly used avenues of treatment for LCPD are observation, surgical intervention, and conservative orthotic management. Decisions about treatment are often guided by age of the child, extent of femoral head deformity, and severity of incongruency between the femoral head and acetabulum.60,61,75 More recent data suggest that patients younger than 6 years of age at the time of disease onset are best managed nonsurgically, whereas the treatment for patients older than 8 years may involve surgery and is less well defined.76
For children with minimal bony deformity, observation and exercise may be the most appropriate intervention.77 Because the child is likely to continue to limp until sufficient revascularization and remodeling have occurred (which may require several years), parents may be uneasy, preferring instead a more aggressive intervention. Parents are reassured when close clinical follow-up is performed, with periodic reexamination by x-ray evaluation to monitor progression of the disease process.
Surgical intervention is based on the principle of containment, optimally positioning the femoral head within the acetabulum. Proximal femoral derotation osteotomy is used to decompress and center the femoral head within the acetabulum for more functional weight bearing in an extended position.78,79 A pelvic osteotomy, which repositions the acetabulum over the femoral head, is sometimes necessary when a significantly enlarged or subluxed femoral head cannot be effectively repositioned by femoral derotation osteotomy.80,81 Shelf arthroplasty to reshape the acetabulum to better accept the femoral head has also been used as an intervention.82,83 The outcome of surgery is likely to be most positive for children who have full hip range of motion preoperatively. Parents must understand the goals and risks of the surgical procedure and must be actively involved in postoperative rehabilitation efforts.
The goal of conservative orthotic management of LCPD is similar to that of surgical intervention: to contain the femoral head within the acetabulum during the active stages of the disease process so that optimal remodeling can occur.41,84 Much debate has taken place concerning whether surgery or orthotic intervention is most efficacious. If both are viable methods of treatment, the end result should be the same: a well-shaped femoral head and pain-free hip. Comparing the efficacy of surgical versus orthotic management of LCPD is challenging because of relatively low incidence as well as differences in study design and definition of control for variables such as age of onset, duration of the disease, gender, and inadequate interobserver reliability of classification systems.84,85 Although two reports published in 1992 question the efficacy of orthotic treatment,86,87 other studies advocate orthotic treatment even in severe cases of the disease. Because studies have reported success, as well as lack of success for all three types of intervention (noncontainment/observation, surgery, and the use of orthoses), the most appropriate management of LCPD has not been clearly determined.
Orthotic Management in Legg-Calvé-Perthes Disease
Currently the most commonly used orthosis in the nonoperative management of LCPD is the Atlanta/Scottish-Rite hip abduction orthosis (Figure 12-12). The design of this orthosis allows the child to walk and be involved in other functional activities while containing the femoral head in the acetabulum with abduction of the hips.88–90 The Atlanta/Scottish-Rite orthosis has three components: a pelvic band, a pair of single-axis hip joints, and a pair of thigh cuffs. An abduction bar may also be included, interconnecting the thigh cuffs with a ball-and-socket joint as an interface. This orthosis holds each hip in approximately 45 degrees of hip abduction, permits flexion and extension of the hip, and can be worn over clothing. While in the orthosis the hips are abducted and flexed but the patient has no limitation in knee range of motion and therefore can sit or walk without difficulty. The orthosis is not designed to control internal rotation of the hip. This type of orthosis is most effective if the child who is wearing it has close to normal range of motion at the hip joint. Limitations in range of motion cause the child to stand asymmetrically in the orthosis, which effectively reduces the amount of abduction and containment of the femoral head.
Historically (in the 1960s and 1970s), a number of other orthoses were developed on the basis of the principles of containment of the femoral head. The Toronto orthosis (Figure 12-13A) and the Newington orthosis (see Figure 12-13B) hold both limbs in 45 degrees of abduction with internal rotation, but unlike the Atlanta/Scottish Rite orthosis, require the use of crutches for safe mobility.91,92Both of these orthoses are cumbersome to wear and significantly affect the ease of daily function. Because the efficacy of the Atlanta Scottish-Rite orthosis is as high as or higher than the efficacy of the Toronto and Newington orthoses, the latter two orthoses are not commonly used in current management of children with LCPD.41,93 In addition, orthoses like an “A-frame” may be used after several weeks of Petrie casting, another nonsurgical treatment option enforcing hip abduction.76
If the disease process progresses and the hip begins to lose additional range of motion, the orthotist may be the first to recognize this problem. The parents may bring the child to the clinic for an orthotic adjustment because the thigh cuffs have become uncomfortable. If loss of additional range of motion is noticed by parents or by therapists who are working with the child, an immediate referral to the orthotic clinic or physician is necessary. The Atlanta/Scottish-Rite orthosis is not designed to increase range of motion; its primary function is to hold the hips in abduction comfortably. Using the orthosis to restore range of motion defeats the purpose of the orthotic design and compromises treatment principles.
Communication among the orthopedist, orthotist, therapist, and family is essential. Parents must understand that this is a demanding form of treatment. Typically, the orthosis is worn continually for 12 to 18 months. Once radiographic evidence of femoral head reossification is seen, time in the orthosis is gradually reduced.
Absolute compliance with the wearing schedule is necessary for maximum effectiveness: A well-designed and well-fit orthosis can only work if it is being used. The first few days and weeks in the orthosis are often stressful for the parent and the child. With the hips held in an abducted position, routine tasks including walking may require assistance until the child learns effective adaptive strategies. Physical therapists may work with the child on crutch-walking techniques on level surfaces, stairs, inclines, and uneven surfaces. They may make suggestions for adaptation of the home and school environments so that sitting and transitions from flooring, chairs, and standing quickly become manageable. If family education and support efforts are effective and enable parents and children to weather this difficult initial stage in orthotic management well, the likelihood of compliance in the remaining months of intervention is significantly enhanced.
Pediatric Postoperative Care
Numerous musculoskeletal and neuromuscular conditions, in addition to LCPD and developmental dysplasia, may necessitate surgical intervention for children with hip and lower extremity dysfunction or deformity. Orthoses that control hip and leg position are often used in the weeks and months after surgery as an alternative to traditional plaster casts or as a follow-up strategy once casts are removed. Although a cast may be applied in the operating room for immediate postoperative care, orthoses are often fit soon afterward and effectively shorten the time that a child spends in a cast. Hip orthoses are used when immobilization and support will be required for a long period of time, when complications arise, or when a child’s special needs demand their use.
One of the major benefits of an orthosis (as compared with a plaster cast) is in regard to hygiene, especially for children who have not yet developed consistent bladder and bowel control. Additional benefits of postoperative hip orthoses include the following:
2. Hip orthoses can be removed for inspection of surgical wounds and for bathing and skin care.
6. The position and amount of abduction can be easily adjusted.
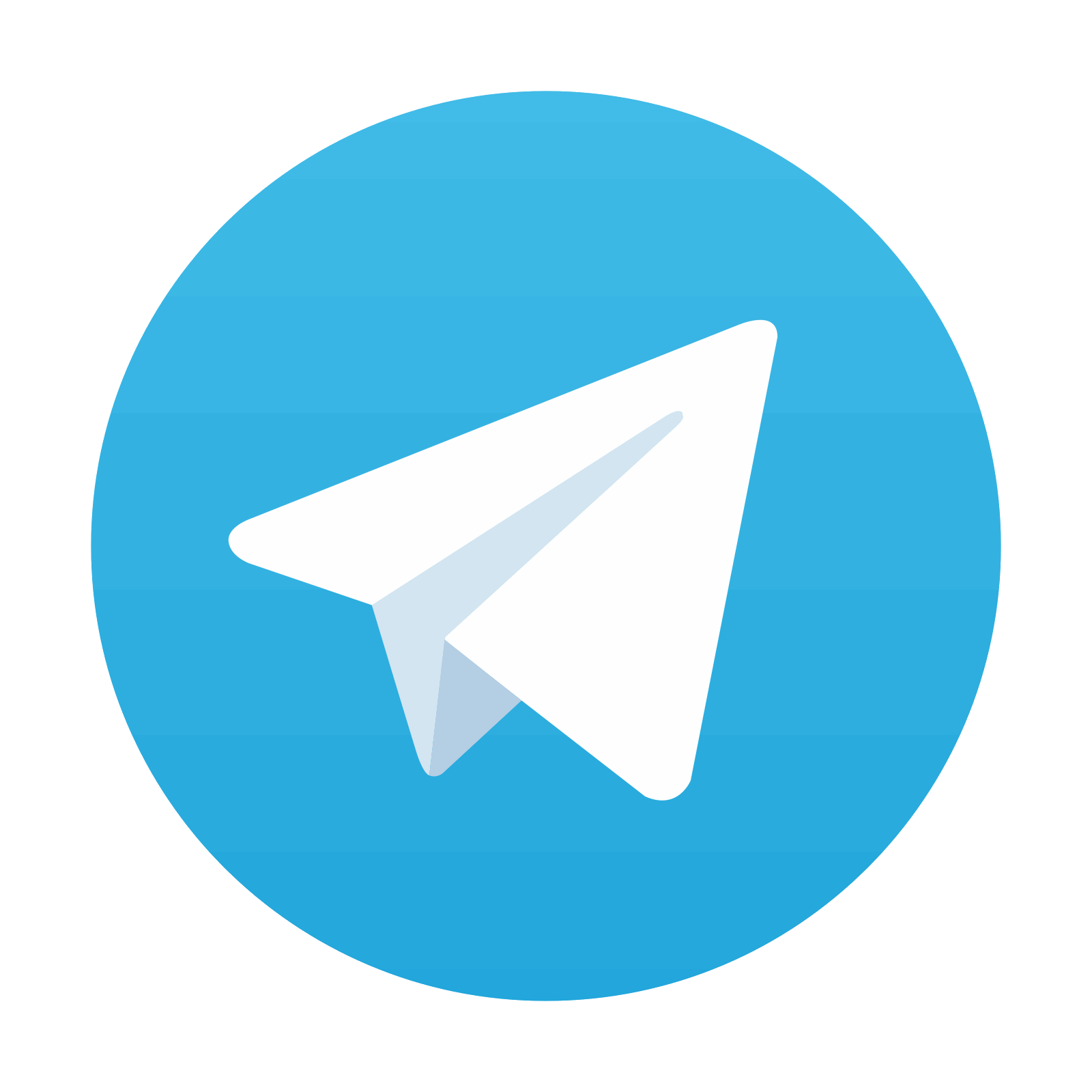
Stay updated, free articles. Join our Telegram channel

Full access? Get Clinical Tree
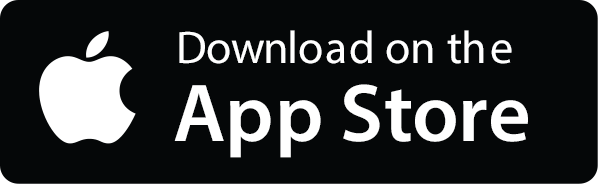
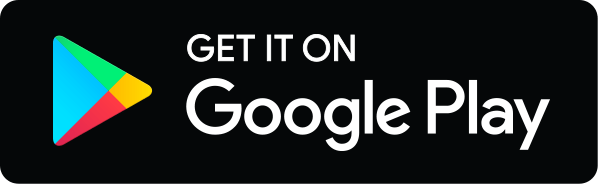