Orthobiologics
D. Scot Malay
William Harris IV
Orthobiologics is a relatively new and rapidly expanding sector in the fields of bone and joint surgery and wound healing. Although the term orthobiologics does not have a clear-cut definition, it is considered to be the use of biologically engineered materials to repair and augment components of the human musculoskeletal system by recruiting and stimulating the body’s natural bone and soft tissue healing mechanisms. The field of orthobiologics is likely to continue to expand and advance due to the shift in treatment principles from repairing bone and joint injuries, and soft tissue wounds, to healing these injuries by means of regeneration and, in turn, restoring original structure and function. Orthobiologic substances were initially considered as those that were used to regenerate bone; however, due to recent advances related to tissue engineering, its realm has broadened to include a wide array of materials that are used throughout the body to regenerate cartilage, ligament, tendon, and skin. This area of medicine and surgery has become a target for new research, including clinical trials, due to the limited supply of autogenous tissue, in particular bone and skin, and morbidity associated with autogenous donor tissue procurement. Senn described the use of decalcified bone matrix for the treatment of osteomyelitis over 100 years ago (1). However, what many surgeons consider to be the beginning of the orthobiologics era was the research of Marshall Urist, and others, in the early 1960s, that focused on augmenting and enhancing bone healing in cases involving fracture and nonunion. Urist et al (2,3) initially identified ectopic bone formation after implanting demineralized bone matrix (DBM) in rabbit muscle. This finding led to the discovery of the protein responsible for bone regeneration, namely, bone morphogenic protein (BMP). Since the discovery of initial BMP, approximately 20 different BMPs have been recognized, along with a number of DBM concentrates, bone substitutes, platelet gels, undifferentiated mesenchymal stem cells (MSCs), and acellular tissue grafts used to provide the optimal environment for healing osseous and soft tissue defects. In this chapter, we provide an overview of orthobiologic materials, including agents used in the process of bone regeneration, such as osteoinductive platelet gels; DBMs and BMPs; osteoconductive materials such as ceramics, hydroxyapatite (HA), and calcium sulfate and calcium phosphate; and osteogenic cells such as undifferentiated MSCs, osteoblasts, and chondroblasts, as well as agents used in the process of soft tissue regeneration, including allogeneic and xenogeneic acellular collagen matrices. An understanding of these orthobiologic materials, their mechanisms of action, and indications for use offers the foot and ankle surgeon tools and methods that are advantageous in regard to healing musculoskeletal injuries.
BONE REGENERATION
Bone regeneration is the foundation from which all orthobiologic applications have been derived. Research on this topic has been used to develop alternative methods to harvesting autogenous bone grafts to heal skeletal defects caused by injury, tumors, nonunions, and infection by formulating products that are biologically similar to bone. A basic understanding of the molecular biology of bone healing allows the surgeon to make reasonable decisions as to which type of nonnative material will most closely simulate bone autograft and allow normal healing to take place. Although autogenous bone graft is still generally considered to be the gold standard, it does not come without pitfalls, and the sentiment of many surgeons appears to be shifting toward a preference for orthobiologic substances rather than pure autograft. The disadvantages to the use of autogenous bone grafts include donor site morbidity, limited supply, additional cost due to increased operating room time, and additional surgical dissection and potential risks and complications. There are three main biologic processes necessary for normal bone healing, namely, osteogenesis, osteoinduction, and osteoconduction. Autogenous bone graft provides all three of these crucial elements, which is the primary reason that autograft remains the ideal replacement material, while most orthobiologic materials enable only one or two of these processes, unless the materials are combined with other orthobiologics or autogenous materials. In addition to these biologic processes, recreating the acute healing phase at the defect site, along with ensuring mechanical stability, provides the best possible environment for bone healing. The type of material used to promote these processes will provide one or more of the required qualities needed for healing an osseous defect.
When bone is injured, damage to the local cells, blood vessels, bone matrix, and periosteum at the fracture site and the surrounding soft tissues occurs. Hematoma forms between the fracture edges, and cells in the local osseous environment die due to ischemia in the fracture cleft, and this extends to a level at which there is sufficient blood supply to prevent hypoxia. Dead, dying, and injured cells release inflammatory mediators that cause intact blood vessels to dilate and transude plasma into the fracture gap and this, in turn, causes edema at the fracture site. Ischemia and hypoxia are also associated with an increase in the presence of free radicals, which leads to further cytolysis that, in turn, stimulates migration of leukocytes, macrophages, and lymphocytes to the site of the injury, thereby completing the acute inflammatory phase. After the acute inflammatory phase, necrotic tissue is reabsorbed and neovascularization is stimulated via cytokines, a process that is followed by migration of fibroblasts and chondrocytes to the area to begin formation of new bone matrix. The rate and amount of repair differs from this point, depending on the area in which the bone is injured, its local blood supply, and the structural composition of the injured bone. Specifically, metaphyseal bone tends to heal faster than epiphyseal and diaphyseal bone, and cancellous bone heals faster than does cortical bone. The stability of the fracture fragments, and the supply of blood to the bone,
also plays major roles in whether or not the bone will undergo primary or secondary bone healing from this point forward.
also plays major roles in whether or not the bone will undergo primary or secondary bone healing from this point forward.
OSTEOINDUCTIVE MATERIALS
Osteoinductive materials stimulate bone healing. These materials induce MSCs to proliferate and differentiate into osteoprogenitor cells. Stimulation of these osteoprogenitor cells and substances, including growth factors and other proteins, leads to the formation of bone or cartilage, depending on the qualities of the local tissue environment. The exact concentrations of these osteoinductors that are needed for bone formation remain unknown and are variable between the different agents in this class and the way that they are processed. The most commonly used osteoinductors include platelet gel and BMPs, as well as DBM, which contains a collagen substrate along with BMPs, so it (DBM) is both inductive and conductive.
Platelet Gel
In acute bone injuries, a number of inflammatory mediators are released from platelets as well as injured and dead cells present at the fracture site. Platelets contain different growth factors, including platelet-derived growth factor, transforming growth factor beta (TGF-β), insulin-like growth factor, and vascular endothelial growth factor, all of which are important in fracture healing (4,5 and 6). Since platelets contain these growth factors, adding them in high concentrations to the fracture site has been shown to aid bone (as well as dermal) healing. Along with enhancement of healing, the fibrin clot that is formed from the introduction of excess platelets serves as a lattice for cellular attachment and ingrowth. To obtain autologous platelets, blood is drawn from the patient preoperatively (or during the operation) and centrifuged, thereby separating it into three components: (a) platelet-rich plasma (PRP), (b) platelet-poor plasma (PPP), and (c) red blood cells. It is the PRP component that is procured for use as an osteoinductor, and the concentrations of each of the components differ based on the method of centrifugation and the amount of blood that is used, which typically varies from 20 to 400 mL (7). Some advanced ultrafiltration techniques can be used to obtain superconcentrated plasma containing up to 8 to 10 times the number of platelets present in whole blood (8). Once prepared, PRP is mixed with thrombin and applied to the fracture site or other bone defect, or to soft tissue repair sites (Fig. 88.1), alone or in combination with other stimulating or conducting agents. The PPP component is high in fibrinogen and can be combined with thrombin and is used to augment wound closure.
Although numerous studies have reported the efficacy of platelet gel in regard to bone healing, its overall effectiveness remains controversial. In 2003, Weiner and Walker (8) assessed, at 1 and 2 years, lumbar fusion using iliac crest bone graft alone compared with iliac crest bone graft augmented with autologous growth factors (AGFs) and noted that the fusion rate in the autogenous graft alone group was 91% and in the autogenous graft augmented with AGF group, the fusion rate was 62%. Based on this observation, they concluded that the use of AGF resulted in inferior rates of arthrodesis in comparison to autogenous bone graft alone, and they attributed the finding to technical factors related to procuring the platelet gel (8). However, in 2005, Sammartino et al (9) analyzed the effects of autologous PRP on periodontal tissues after extraction of the third molar, and their results showed that the use of PRP accelerated bone regeneration. In 2006, Jenis et al (10) compared AP interbody fusion using iliac crest bone graft with allograft combined with AGFs, in an effort to determine differences in clinical and radiographic outcomes between the treatment groups. To assess clinical outcomes, they used preoperative and postoperative visual analog scale scores for pain, the 36-item Short-Form Health Survey, and the Oswestry Disability Index. Their average follow-up duration exceeded 24 months in both treatment groups, and they concluded that AGF combined with an allograft carrier was equivalent to autograft in terms of clinical and radiographic outcomes, and they noted no inhibitory effect of AGF in regard to graft incorporation. They also suggested that AGF combined with an appropriate carrier is a reasonable alternative to autograft and other more expensive bone induction technologies (10). To date, there remains a lack of well-designed randomized controlled trials involving the use of platelet gels in the field of foot and ankle surgery, so the utility of this enhancer in bone healing is not clearly known.
Bone Morphogenic Proteins
Bone morphogenic proteins play a key role in the migration and proliferation of MSCs, their differentiation into osteogenic and chondrogenic cells, vascular ingrowth, and bone remodeling (11). BMPs are low-molecular-weight, noncollagenous glycoproteins that belong to the TGF-β superfamily (12). BMPs are acquired by means of purifying DBM (Fig. 88.2); however, naturally occurring BMPs are only available in small amounts (micrograms per kilogram of bone matrix). There have been over 20 different BMPs discovered to date, and only BMPs 2 to 7 and BMP-9 have been shown to induce new endochondral bone formation on their own (13). During the migration of cells into the hematoma that has formed at the fracture gap, BMPs 2, 6, and 9 are essential in early differentiation of MSCs into preosteoblasts, and BMPs 4 and 7 stimulate differentiation of preosteoblasts to osteoblasts (14). These BMPs are present in different concentrations at various points in time throughout the healing process, depending upon local biologic and biomechanical stimuli that promote maintenance of an organized fracture callus, resulting in new bone formation at the fracture
site. Due to the large ratio of bone required to produce small amounts of BMP, recombinant gene technology has become a mainstay for the production of these proteins. Gene therapy has been used to reproduce these naturally occurring substances, in the form of recombinant human bone morphogenic protein (rhBMP). Currently, there are only two rhBMPs available for use, namely rhBMP-2 and rhBMP-7, which is also known as osteogenic protein-1 (OP-1).
site. Due to the large ratio of bone required to produce small amounts of BMP, recombinant gene technology has become a mainstay for the production of these proteins. Gene therapy has been used to reproduce these naturally occurring substances, in the form of recombinant human bone morphogenic protein (rhBMP). Currently, there are only two rhBMPs available for use, namely rhBMP-2 and rhBMP-7, which is also known as osteogenic protein-1 (OP-1).
In 2002, Govender et al (15) performed a prospective, randomized controlled study of 450 open tibial fractures using rhBMP-2 and compared the rates of secondary intervention needed to achieve successful healing. The patients were divided into three treatment groups: one received the standard treatment for the open fracture, the second received 0.75 mg/mL of rhBMP-2 at the fracture site in addition to standard treatment, and the third group received 1.50 mg/mL of rhBMP-2 in addition to standard therapy. The prevalences of secondary interventions were 46%, 37%, and 26%, respectively. The treatment group with the highest concentration of rhBMP-2 showed highest proportion of wound healing, the highest and most rapid union rate, and the lowest rate of infection (15). Friedlaender et al (16) compared the effectiveness of BMP-7 (OP-1) in a collagen carrier versus the use of autograft for the treatment of tibial nonunions with intramedullary nail fixation. At 9 months, clinical and radiographic assessments were performed and showed no significant difference between the two groups. However, they found a statistically higher prevalence of atrophic nonunions (41% vs. 25%) and more smokers (74% vs. 57%) in the OP-1 group, which could have affected their outcomes. They also found a significantly lower risk of infection (3% vs. 21%) at the implantation site, in the OP-1 group. They concluded that OP-1 combined with a collagen carrier offered the advantages of a highly inductive molecule with an excellent safety profile, along with the lack of donor site morbidity, and, as a result of their observations, they recommended the use of OP-1 for the management of tibial nonunions (16).
Osteoconductive materials.
Osteoconductive substitutes are materials that have a structural composition similar to cancellous bone and therefore supply a scaffold-like matrix that allows the proliferation, attachment, and eventual passage of MSCs throughout its substance.
Demineralized Bone Matrix
Demineralized bone matrix is human allograft bone that has been made void of its mineral content, yet retains the collagenous and noncollagenous proteins and growth factors that serve both as an osteoconductive medium and an osteoinductive substrate. There are many different DBM products available in the market place, and they are readily available to the operating surgeon. The specific demineralization process varies from product to product, while the basic principle of the process is generally the same. In essence, allograft cadaveric donors are initially screened for inclusion and exclusion criteria based on medical and social history as well as microbiologic and serologic testing that is undertaken to ensure that transmission of disease does not occur. The bone is then harvested in a sterile manner, washed, treated with antibiotics, demineralized with acid, and then sterilized in one fashion or another. The main methods of sterilization include sonication, electron beam, or gamma irradiation. It is critical for surgeons to realize that some of the inductive capacity of the DBM is threatened as a result of the demineralization and sterilization processes, and manufacturers will tout one product over another based on this fact. Some manufacturers, moreover, will randomly test batches of their DBM for inductive capacity, and this information can be useful to surgeons and may bias the decision as to which DBM will be used.
Once sterilization has been achieved, the remaining organic material in the DBM is combined with a carrier, such as glycerol, gelatin, lecithin, sodium hyaluronate, hydrogel, or calcium sulfate, and this also affects the handling properties of the DBM. Some DBM preparations, such as those containing glycerol, are very soft and readily wash away when the wound is lavaged while being very easy to pack and place about a comminuted fracture or complex bone-to-bone interface, whereas other preparations, such as putty or sheets, are more firm and dense, and resist washout while retaining the configuration in which they were placed. Although the particular carrier affects the handling and application characteristics of the DBM, it has no effect on sterilization, preservation, or storage, and it does not affect the chemical or metabolic action of the DBM (7,17). Again, the amounts of active induction factors present in DBM after demineralization vary with the processing technique used to manufacture the product, and this fact should be taken into consideration when choosing an application (18,19). DBM is available in several different forms, ranging from injectable gel to paste, putty, blocks, and combinations with freeze-dried allogeneic cancellous bone chips (Fig. 88.3). The different compositions determine washout strength and the product’s ability to be molded and to fill voids in bone. Peterson et al (17) compared three different DBMs for use in spinal fusion in athymic rats and showed that fusion rates were highest in the electron beam sterilized glycerol carrier product, as determined by radiographic, histologic, and manual testing of the arthrodesis sites. Bae et al (20) showed, by means of ELISA assay for detection of BMPs 2, 4, 7, and 9, variability in retention of inductive proteins between different manufacturers’ DBMs and also variation between different lots that were prepared in the same fashion. Their results brought to question the reliability, in regard to inductive capacity and
possibly efficacy, of DBMs, particularly as it relates to providing consistent osteoinduction.
possibly efficacy, of DBMs, particularly as it relates to providing consistent osteoinduction.
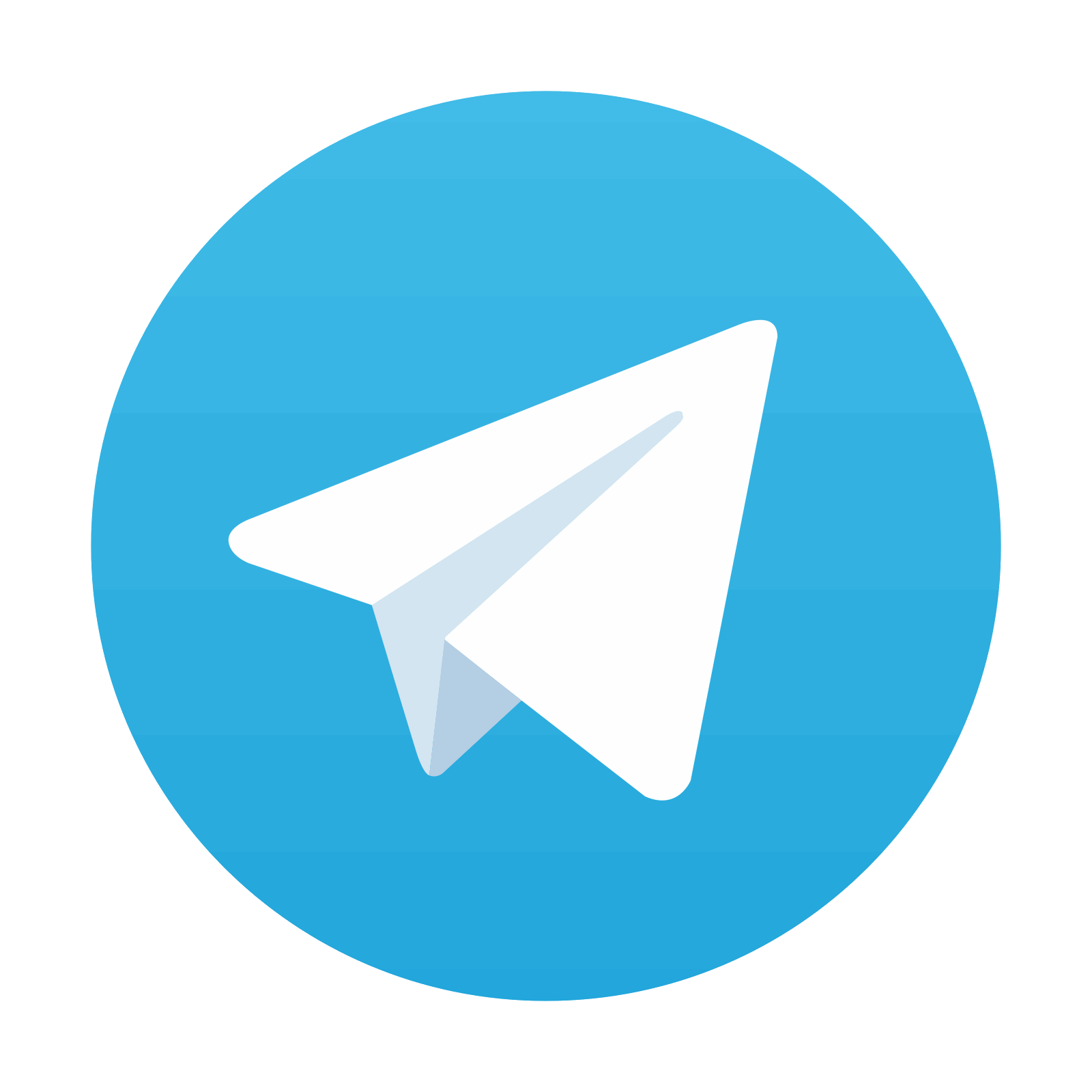
Stay updated, free articles. Join our Telegram channel

Full access? Get Clinical Tree
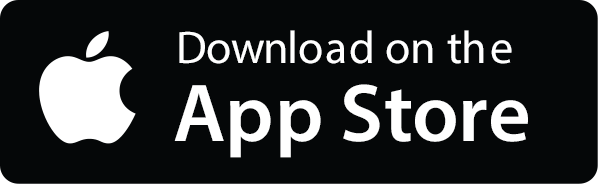
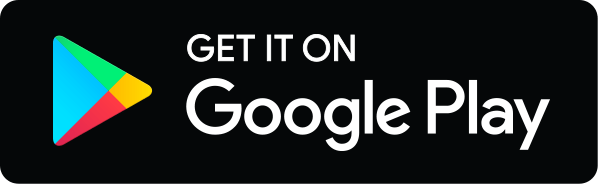
