Osseointegration is a biologic process vital to modern endoprosthetic fixation in orthopedic surgery. Numerous engineering advancements have improved the utilization of this technology in orthopedics; however, issues such as stress shielding, aseptic loosening, and bone loss remain an ongoing challenge. These host responses are especially problematic in limb salvage surgery. Novel applications of osseointegration have been developed to address some of these fixation issues with massive tumor endoprostheses. This articles reviews osseointegration and emerging novel applications of this technology in limb salvage surgery and future prosthetics for amputees.
Key points
- •
Stress shielding and bone loss are common complications of endoprosthetic arthroplasty implants used in orthopedic surgery.
- •
Deficient bone stock is major challenge in primary and revision limb salvage reconstructions for benign and malignant bone tumors.
- •
Compressive osseointegration is a novel application of osseointegration technology designed to improve bone stock and facilitate short segment bone fixation.
- •
Compressive osseointegration has been shown to provide a viable fixation alternative for primary and revision endoprosthetic limb salvage reconstructions.
Introduction
Endoprosthetic bone and joint reconstructions have revolutionized the orthopedic management of a variety of degenerative, congenital, traumatic, and oncologic musculoskeletal conditions. As a consequence of the success of these procedures, indications for endoprosthetic joint reconstructions are ever increasing, as are societal expectations for improved function and durability of these implants. Numerous aspects of implant design, such as modifications in implant geometry, more durable bearing surfaces, and advances in the bone–implant interface, have substantially contributed to the increasing success and utilization of endoprosthetic bone and joint reconstructions. As the number of these endoprosthetic reconstructions performed yearly increases, especially in younger patients, so are the number of revision arthroplasty procedures, which are frequently complicated by significant bone loss, infection, postoperative complications, and inferior patient outcomes. Consequently, modern implant designs that maximize longevity while limiting the biological and technical morbidities associated with revision surgery are desirable.
Introduction
Endoprosthetic bone and joint reconstructions have revolutionized the orthopedic management of a variety of degenerative, congenital, traumatic, and oncologic musculoskeletal conditions. As a consequence of the success of these procedures, indications for endoprosthetic joint reconstructions are ever increasing, as are societal expectations for improved function and durability of these implants. Numerous aspects of implant design, such as modifications in implant geometry, more durable bearing surfaces, and advances in the bone–implant interface, have substantially contributed to the increasing success and utilization of endoprosthetic bone and joint reconstructions. As the number of these endoprosthetic reconstructions performed yearly increases, especially in younger patients, so are the number of revision arthroplasty procedures, which are frequently complicated by significant bone loss, infection, postoperative complications, and inferior patient outcomes. Consequently, modern implant designs that maximize longevity while limiting the biological and technical morbidities associated with revision surgery are desirable.
Cement fixation of orthopedic implants
Of considerable interest to modern implant design is the bone–implant interface. Historically, orthopedic endoprosthetic fixation was achieved using an acrylic bone cement, polymethylmethacrylate (PMMA), championed by the success of Sir John Charnley’s cemented total hip arthroplasty. Cemented fixation of orthopedic implants remains common practice with excellent longitudinal results. PMMA does not have adhesive or biologic properties, and functions as a grout, filling macroscopic and microscopic voids between host bone and the implanted device. The longevity of cemented implants is largely influenced by the quality of apposition between the implant–cement and bone–cement interfaces. Although beyond the scope of this review, numerous variables, such as cement preparation, implant geometry, implant surface texture, and cement mantle thickness, contribute to the success of cemented implant fixation. In addition to the technical and time requirements associated with cement fixation, a major limitation of cemented fixation is a lack of biological or osteogenic activity at the implant–bone interface. Additionally, when cemented orthopedic implants are revised, cement removal can be onerous and necessitate additional bone loss. Well-fixed cemented implants are also associated with stress shielding of surrounding bone.
Biologic fixation using osseointegration
To circumvent some of the shortcomings associated with cemented fixation, press–fit implants augmented with porous coated surfaces have become the dominant fixation strategy for many orthopedic endoprosthetic implants. By forcibly wedging slightly oversized implants into bone, implants are immediately stabilized by a combination of frictional forces and hoop stresses. However, the natural biologic tendency of the body is to wall off foreign material with an encapsulating layer of inflammatory, fibrous tissue, which can result in implant loosening and failure. To minimize this natural host response, various implant surface modifications have been introduced to promote a spectrum of bone anchorage to the implanted device. Bone–implant adherence ranges from bony ongrowth, where bone simply adheres to a roughened surface, to bony ingrowth, also known as “osseointegration,” in which bone trabeculae form within the metal’s porosities.
Initially coined by P-I Brånemark, osseointegration refers to direct structural and functional connection between ordered, living bone and the surface of a load-carrying implant. Host bone is anchored into the implanted device, resulting in an intimately coupled bone–implant interface, which is stable under physiologic loading conditions. Although the concept of osseointegration was first put forth to define the connection between bone and titanium, bone ingrowth can also be achieved with the use of other metals, metal alloys, and ceramics. Osseointegration has thus become a general term used to describe any type of intimate bone–implant surface contact.
Factors influencing osseointegration
Osseointegration is a complex biological process and depends on numerous implant factors, such as the implant’s material properties, surface characteristics, and biocompatibility. Host factors, such as the mechanical loading environment, inherent host biology, location and local quality of the host bone, are also important. The biology at the host bone–implant interface is strongly characterized by the composition of the implanted material. Titanium is the prototypical metal used for osseointegration, because it is biologically inert, corrosion resistant (unlike stainless steel), and has a modulus of elasticity more biologically comparable to bone. The bioinert properties of titanium are largely attributable to the naturally occurring surface oxide layers. Alloys such as cobalt–chrome–molybdenum are less bioinert than titanium. However, these are still compatible with osseointegration when augmented with surface modifications as described herein. In general, more bioreactive implant materials are more likely to be encapsulated with fibrous tissue, thus impeding uniform osseointegration. Titanium implants are less bioreactive and are associated with more rapid and superior osseointegration compared with stainless steel and cobalt chrome alloys. Additionally, the compatible modulus of elasticity between bone and titanium permits a more favorable load sharing relationship in bone; this provides an advantageous biomechanical environment when bone is stimulated with physiologic forces.
Implant surface characteristics are also extremely important for osseointegration. Bony ingrowth requires a pore size between 50 and 400 μm. This can be obtained by coating substrate implant metals with sintered beads, fiber mesh, and porous elements, such as tantalum metal, titanium, and hydroxyapetite. Porous metals and ceramics can be added to the implant by plasma spraying, a technique that involves mixing metal powders with a pressurized and ionized inert gas, forming a high-energy flame. The molten material is sprayed onto the implant, creating a textured surface. Hydroxyapatite is a porous calcium phosphate ceramic used in many orthopedic implants for its appealing osteoconductive properties and relatively inert bioreactivity.
Osseointegration is a dynamic biologic process that evolves and matures over a 4- to 12-week time frame, and likely beyond. Successful osseointegration requires stable fixation, limited micromotion, and intimate contact. Micromotion of greater than 150 μm leads to fibrous tissue formation at the bone–implant interface, whereas less than 20 μm of motion results predominantly in bone formation. Motion amplitudes between 40 and 150 μm leads to a combination of bone and fibrous tissue formation. In most osseointegrating orthopedic endoprostheses, immediate stability is achieved by an interference fit, which is largely a product of frictional and hoop forces. In theory, once osseointegration has occurred, definitive stability is obtained via anchoring of the host bone into the porous spaces of the implant.
Despite an increasing understanding of the biological complexities of the bone–implant interface and numerous advances in implant design, osseointegration has a variety of limitations. Because osseointegration is a biologic process, host bone quality and physiology may negatively impact bony ingrowth. For example, preclinical models have demonstrated that osseointegration is delayed and quantitatively decreased in osteopenic bone. Diabetes, smoking, and peripheral vascular disease have also been associated with impaired osseointegration. Pertinent to orthopedic applications, even in the setting of optimal osseointegration, deleterious factors such as stress shielding, particle-induced osteolysis, bone loss, and infection may disrupt the osseointegration of the bone–implant interface. Stress shielding of well-fixed porous ingrowth implants is an ongoing challenge in orthopedics. This can be reduced using implants intended for proximal, metaphyseal ingrowth and by using implants with a lesser modulus of elasticity. Minimization of these common orthopedic endoprosthetic complications is a focus of ongoing investigations and collaboration with industry partners.
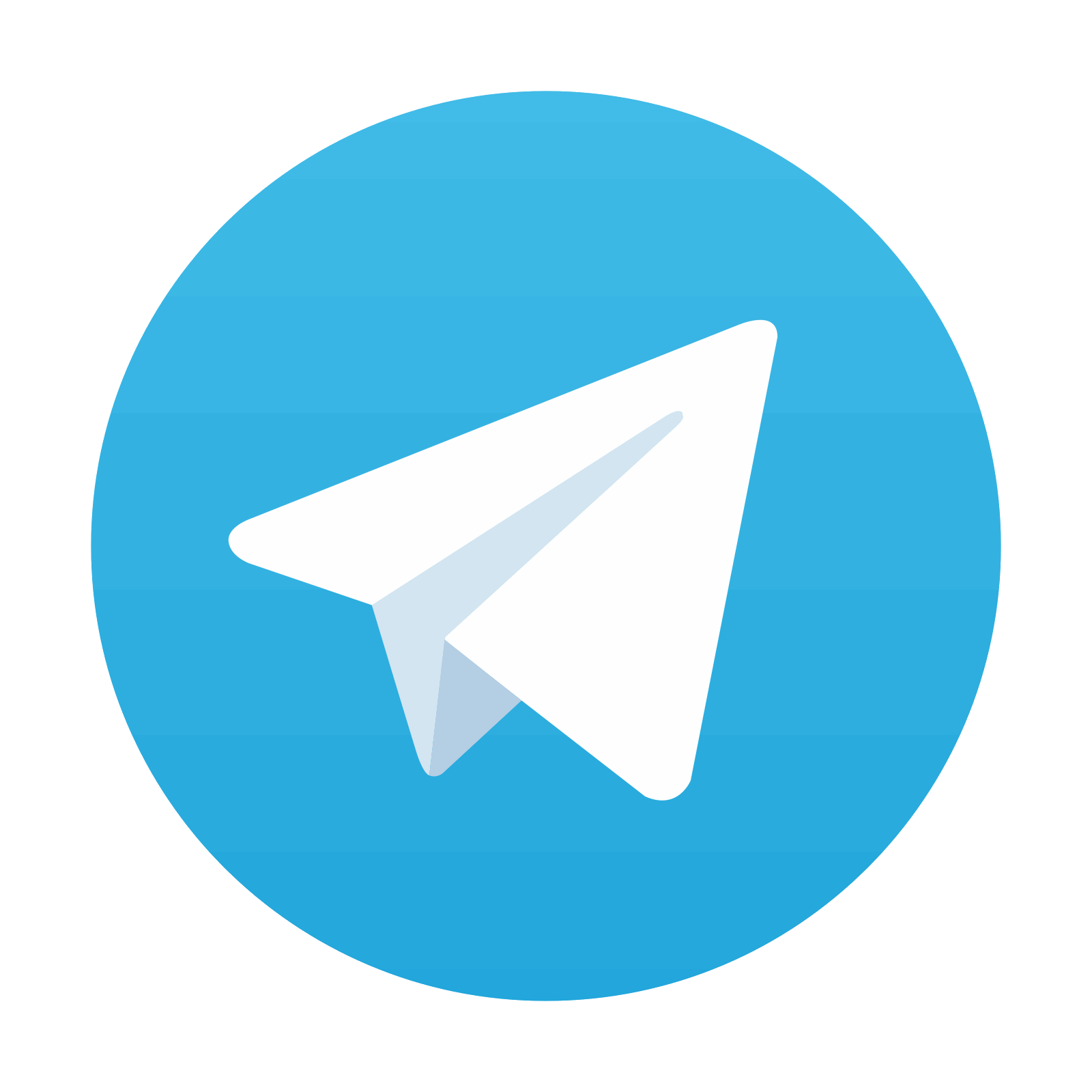
Stay updated, free articles. Join our Telegram channel

Full access? Get Clinical Tree
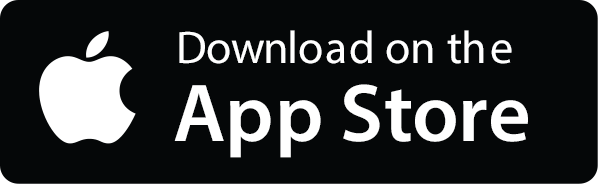
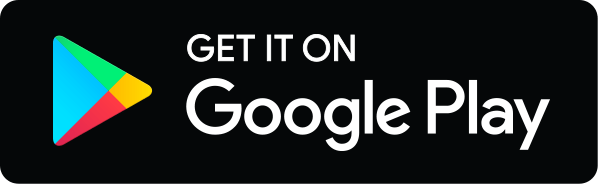