Injuries to the flexor tendons remain among the most difficult problems in hand surgery. Historically, lacerations to the intrasynovial portion of the flexor tendons were thought to be unsuitable for primary repair. Despite continuing advances in our knowledge of flexor tendon biology, repair, and rehabilitation, good results following primary repair of flexor tendons remain challenging to achieve.
Key points
- •
Flexor tendon injuries can be challenging, especially in zone II.
- •
A strong repair using at least a 4-strand core suture and an associated epitendinous suture will allow for early rehabilitation, which can minimize the risk of adhesion formation. Core sutures should have a minimum of 7-mm to 10-mm depth of purchase, whereas epitendinous sutures should have a 2-mm rim for repair.
- •
Bulky repairs should be avoided to allow for smooth tendon function. On occasion, the A2 or A4 pulleys may be vented up to 50% to avoid impingement.
- •
Wide-awake local anesthesia allows for intraoperative motion testing and appropriate adjustment of the repair to prevent postoperative rupture.
- •
Early active motion protocols can result in better outcomes, especially when combined with the guidance of certified hand therapists.
Introduction
Despite advancements in our understanding of flexor tendon biology, repair, and rehabilitation, a successful outcome after intrasynovial flexor tendon injury can be difficult to achieve. This requires a thorough understanding of the biologic principles of tendon injury and healing, a detailed knowledge of normal and pathologic flexor tendon anatomy, an attention to meticulous surgical technique, a careful postoperative rehabilitation protocol, and a surgeon, therapist, and patient who are motivated to enact that protocol.
Introduction
Despite advancements in our understanding of flexor tendon biology, repair, and rehabilitation, a successful outcome after intrasynovial flexor tendon injury can be difficult to achieve. This requires a thorough understanding of the biologic principles of tendon injury and healing, a detailed knowledge of normal and pathologic flexor tendon anatomy, an attention to meticulous surgical technique, a careful postoperative rehabilitation protocol, and a surgeon, therapist, and patient who are motivated to enact that protocol.
Anatomy
The flexor digitorum superficialis (FDS) muscle originates proximally in the forearm, and is innervated by the median nerve. The flexor digitorum profundus (FDP) lies deep to the FDS and has a dual innervation: the FDP to the ring and small fingers by the ulnar nerve and the FDP to the index and long fingers by the median nerve. The FDP tendons all arise from a common muscle belly, although the tendon to the index finger may arise separately. Distally, at the level of the carpal tunnel, the FDS to the long and ring fingers lies superficial to the FDS of the index and small fingers. Each digit has both an FDS and an FDP tendon. At the level of the metacarpal neck, the tendons enter the synovial sheath, at which point the FDS splits into halves that course dorsally around the FDP tendon. These halves then rejoin at the Camper chiasm, then split again to insert independently on the middle phalanx.
The flexor tendon sheath begins at the level of the metacarpal neck, and consists of 5 annular and 3 cruciate pulleys. The A1, A3, and A5 pulleys arise from the volar plates of the metacarpophalangeal (MCP), proximal interphalangeal (PIP), and distal interphalangeal (DIP) joints, respectively. The A2 and A4 pulleys arise from the volar portion of the proximal and middle phalanges. The first cruciate pulley (C1) is between A2 and A3, C2 is between A3 and A4, and C3 is between A4 and A5.
The flexor pollicis longus (FPL) has a unique anatomy. The muscle originates from the middle and proximal aspect of the distal radius and is innervated by the anterior interosseous nerve. The FPL tendon courses through the carpal tunnel in a dorsal and radial position. The pulley system of the FPL consists of 2 annular pulleys, the A1 and A2 pulleys, which originate from the volar plates of the MCP and interphalangeal (IP) joints, respectively. The diagonally oriented oblique pulley is critical to maintaining normal FPL function and is located over the proximal phalanx between the 2 annular pulleys.
The nutritional supply of the flexor tendons has important clinical implications. The tendon sheath provides nutrition via passive diffusion through its synovial fluid, and it contains visceral and parietal layers, which together create a smooth gliding surface for the tendons. In addition, the tendons receive a direct blood supply through connections from the digital arteries known as vinculae. Each tendon has long and short vinculum, which are supplied by a transverse “ladder branch” of the digital arteries. The tendons also receive blood supply from intrinsic longitudinal vessels proximally, from the proximal synovial reflection, and from a direct blood supply from the distal phalanx.
Each vinculum enters the tendon on its dorsal surface, resulting in the dorsal aspect of the tendon being the most well vascularized. The dorsal aspect of the tendon is also the optimal biomechanical location for suture placement during tendon repair. Sutures placed in this location theoretically may interfere with the blood supply. In addition, between the insertion of the vinculae, each tendon has a hypovascular watershed zone. In this area, the tendons rely on passive diffusion through the sheath. The hypovascular zone of the FDS tendon is located in the region of the Camper chiasm, between the vinculum breve superficialis (VBS) and vinculum longum superficialis (VLS). The FDP has 2 hypovascular zones: proximally in the region of A2 between the intrinsic longitudinal vessels and the vinculum longum profundus (VLP), and distally between the VLP and vinculum breve profundus (VBP). In addition, there is a distal dorsal hypovascular zone of Ditsios between the vessels supplying the FDP at its insertion and the VBP.
The course of the flexor tendons of the fingers has been divided into 5 zones. Zone I is distal to the insertion of the FDS tendon and contains only the FDP tendon until its insertion into the base of the distal phalanx. Zone II extends the length of the synovial sheath, and contains both the FDS and FDP tendons. Tang has subdivided zone II into 4 parts: IIa to d. The area beneath the A2 pulley, zone II-c, is the area in which satisfactory functional recovery is most difficult to achieve after primary repair of both the FDP and FDS tendons. Zone III includes the region extending from the proximal aspect of the tendon sheath to the distal aspect of the transverse carpal ligament. This zone contains the lumbrical muscles. Zone IV is beneath the transverse carpal ligament within the carpal tunnel, and zone V is the forearm proximal to the carpal tunnel. In the thumb, zone I is located distal to the IP joint. Zone II is between the MP and IP joints, and zone III lies beneath the thenar muscles.
Patient evaluation
The initial evaluation of a patient with a flexor tendon injury is important in formulating a diagnosis, establishing a treatment plan, and counseling the patient regarding expected outcomes. The mechanism of injury has implications for the quality of the tendon and the status of the surrounding soft tissues. A sharp laceration is more likely to have a cleanly cut tendon end and less soft tissue damage than a crushing or avulsion-type mechanism. The position of the finger at the time of injury can help predict the location and lengths of the underlying tendon stumps. A finger that was held in flexion at the time of laceration can be expected to have a shorter, more distally located stump and may require more substantial distal wound extension.
Observation can provide many useful details about the nature of the injury. Any soft tissue defects or deformities suggesting underlying bony injury should be noted. In the absence of any concomitant bone or joint injuries, a digit with lacerated FDP and FDS tendons will assume an extended position ( Fig. 1 ). In this scenario, the tenodesis effect, which would typically cause flexion of the digit, will not occur.
Each flexor tendon must be examined independently. Stabilizing the middle phalanx of the injured digit and asking the patient to actively flex the DIP joint isolates the FDP. The FDS is examined by holding the adjacent digits in extension and asking the patient to flex the injured digit at the PIP joint. This maneuver tethers the FDP and ensures that digital flexion is occurring through the FDS alone.
A neurovascular examination is performed before administration of local anesthesia or sedation. The digit is examined for color, turgor, and capillary refill time. Both ulnar and radial digital neurovascular bundles are examined and assessed for digital pulses (using a Doppler probe if necessary) or abnormalities in static 2-point discrimination. If necessary, microvascular repair or revascularization can be performed.
Radiographs of the injured digit are routinely obtained to ensure there are no foreign bodies or underlying bony abnormalities. Advanced imaging studies, such as MRI or ultrasound, are typically not needed in the acute situation but may be helpful in delayed presentation when the location of the proximal stump is in question. Antibiotics and tetanus are administered as warranted by the clinical situation.
Principles of tendon repair
There are several variables that can affect the quality of flexor tendon repair. The resistance to gap formation at the repair site and the ultimate strength of the repair are interrelated. They are primarily influenced by the number of core suture strands, the core suture configuration, the core suture size, and the addition of a peripheral epitendinous suture. However, additional factors influence the strength of a repair including material properties of the suture, presence of locking sutures, number of knots, tension of the core sutures, and depth of suture purchase.
The number of core suture strands across the repair is the single most important factor in the strength of repair. It has been demonstrated that repairs in which there are multiple strands crossing the repair site have greater tensile strength than those with fewer strands. Four-strand repairs have been shown to be superior to 2-strand repairs both at time zero and during the first 6 weeks after repair. Six-strand and 8-strand repairs have been shown to be even stronger in vivo. Although these multistrand repairs do have increased bulk, this has not been shown to have an adverse impact on tendon gliding. The primary limitation to these repairs is the surgeon’s ability to effectively place the core suture while minimizing trauma to the tendon and surrounding sheath, which can cause adhesions. Use of looped sutures can decrease the need for handling the tendon, while facilitating the placement of multistrand core sutures. Pretensioning of the core suture to approximately 10% tension does not cause tendon bulkiness but can increase the resistance to initial gapping with early motion.
Besides the number of strands across the repair, the configuration of the repair is important. Many suture repair configurations have been developed in both animal and human cadaveric models. The basis behind different core suture configuration can be simplified into 3 components: the longitudinal component, the transverse component, and the link connecting them. The Kessler 2-strand suture technique has been known as the workhorse for flexor tendon repair and has been modified many times since its introduction. The original Kessler 2-strand suture technique consists of a continuous loop anchored at 4 corners; however, this technique has encountered problems with tendon deformation under load due to load transmission from the longitudinal components to the transverse components. When an axial load is placed across the repair site, the longitudinal components can lengthen while the transverse components narrow. To avoid this, a double-Kessler technique results in a 4-strand repair with significantly smaller gap formation. The cruciate repair technique eliminates the transverse component and therefore can better withstand axial forces ( Fig. 2 ). Specifically, it has demonstrated less elongation at 15 N and 30 N compared with a double Kessler and a continuous Kessler (4-strand repair with 1 knot). Without a transverse component, the cruciate technique tends to fail via suture pullout at higher ultimate tensile strengths. Finally, other strong repairs, such as the augmented Becker repair (Massachusetts General Hospital [MGH] repair) and its modifications, have been developed and appear to not weaken during dynamic, nonlinear testing. This is likely attributed to its cross-locking stitches that help maintain strength in the initial softening period after a repair. In cyclical testing, the MGH repair technique had significantly higher force for 2-mm gap, higher ultimate load to failure, and increased stiffness compared with the modified Kessler suture.
No matter what configuration is used, the size of suture purchase has been shown to effect repair strength. The depth of purchase of the core suture in the tendon should be at least 7 mm, and ideally greater than 10 mm, to avoid significant weakening of the repair. Recently, in an in vivo canine model, an 8-strand core stitch using 4-0 suture with a purchase of 1.2 cm along with a 5-0 epitendinous suture repair with 2-mm purchase length and depth demonstrated significant improvements in gap formation and rupture compared with a configuration with purchase of 0.75 cm and a 6-0 epitendinous suture repair with 1-mm purchase length and depth. Clearly, the size and depth of both core and epitendinous sutures is important.
Another method of increasing the strength of repair is by locking of the suture loops. This occurs when the suture loop tightens around bundles of fibers as longitudinal tension is applied. Locking loops have been shown to have greater time-zero tensile strength than grasping loops where this tightening does not occur. Pennington’s modification of the Kessler 2-strand repair demonstrates the spatial relationship of the transverse and longitudinal limbs and its contribution to the ultimate strength of a repair whereby locking occurs when the transverse component passes volar to the longitudinal component. The locking loop can be ensured by placing the vertical component of the suture deep to the transverse component of the suture, relative to the cut end of the tendon. Both a diameter of 2 mm and the perpendicular placement of locks to the long axis of the tendon are vital to the strength of the lock. The ideal size of a locking loop should involve 25% of the tendon’s width to maximize repair strength.
Furthermore, the caliber of the core suture is an important determinant of repair strength. Sutures of increased caliber have greater strength to failure than sutures of lesser caliber. Larger suture is more likely to fail by pullout than smaller-caliber suture, which is more likely to fail by rupture. Although larger suture is favorable, sutures thicker than 3-0 in size should be avoided as they can increase the bulk of the repair. Moreover, specific suture material has an effect on the strength of repair. Fiberwire (Arthrex, Naples, FL) is a long-chain polyethylene with braided polyester jacket that has become increasingly popular in tendon repair because of its superior tensile strength over similar-caliber ethibond suture. It is primarily limited by its poor knot-holding characteristics, which require a minimum of 6 knots to be thrown to decrease the risk of unraveling. To limit knot failure, other materials have been studied. In cadavers, a monofilament stainless steel cable-crimp system has demonstrated good biomechanical strength of repair. In addition, barbed suture technology has demonstrated some promise. In an adult pig FDP model, Sato and colleagues found that barbed suture possessed greater tensile strength before gap formation than monofilament suture of the same material.
Following core suture placement, the addition of a peripheral epitendinous suture is recommended, as it has several advantages. First, it helps to decrease bulk by smoothing out the repair site. Second, the addition of the peripheral suture itself contributes up to 50% of the ultimate load to failure and potentially up to 50 N of strength. Several epitendinous configurations have been suggested, including simple running, cross-stitch, interlocking cross-stitch, and interlocking horizontal mattress. A simple running peripheral suture should be placed 2 mm from the cut end of the tendon.
The current recommendation for repair is to use a 4-strand or greater core suture combined with a running epitendinous suture. Most surgeons will use a synthetic, braided suture of 4-0 caliber or greater. This should achieve a repair site tensile strength sufficient to perform a postoperative motion protocol without significant risk of gap formation. If an epitendinous suture is not used, a 6-strand core repair is recommended with slight tensioning of the core sutures to prevent gapping. It should be noted that the repair strength of the core stitch and epitendinous suture are not summative and can fail independently.
Biology of tendon repair
Several growth factors have been recognized to increase after tendon injury, which may contribute to both tendon healing and scar formation. These include vascular endothelial growth factor, insulinlike growth factor, platelet-derived growth factor (PDGF), basic fibroblast growth factor (bFGF), and transforming growth factor-ß. The transcription factor NF-κB has been shown to increase for up to 8 weeks after tendon injury. Fibronectin and integrins are upregulated during the early postoperative period in tendons undergoing early passive mobilization.
To increase healing potential and to reduce adhesion formation, attempts have been made to manipulate the healing environment for intrasynovial flexor tendon repairs. In vitro studies on canine fibroblasts have revealed a dose-dependent effect on flexor tendon cell proliferation from the synergy of PDGF-BB and bFGF. The sustained release of PDGF-BB from fibrin matrices by the manipulation of heparin concentrations in heparin-binding delivery systems has been shown to increase cell proliferation and matrix production. Advances in gene therapy have allowed experimentation with transfection of various gene sequences. Tang and colleagues used leghorn chicken FDP tendons and an adeno-associated viral vector to transport bFGF genes directly onto the cut tendon ends, resulting in significantly increased strength at 2 and 4 weeks and also significantly fewer adhesions at 12 weeks compared with controls. Finally, tendons treated with hyaluronic acid (HA) have showed improved gliding mechanics, and those modified through carbodiimide derivatization (cd-HA) may even have improved HA retention.
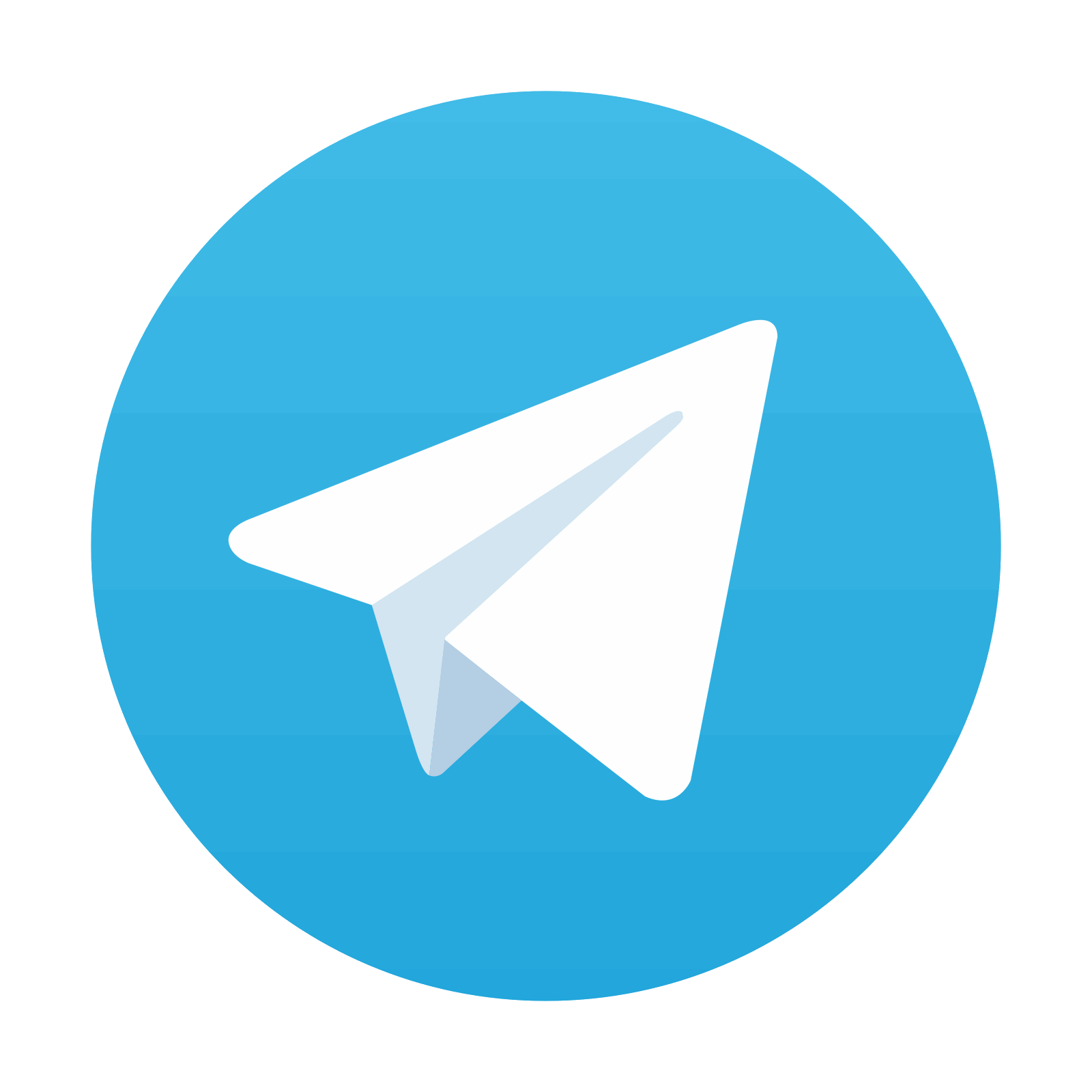
Stay updated, free articles. Join our Telegram channel

Full access? Get Clinical Tree
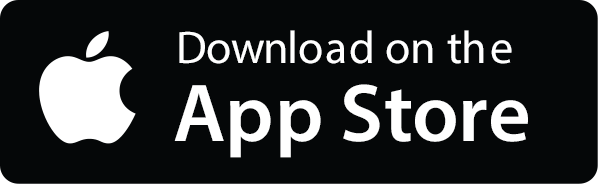
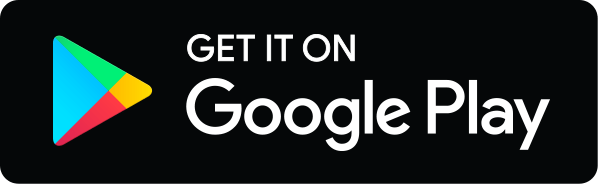