This article describes the physiologic and neural mechanisms that cause neuromuscular fatigue in racquet sports: table tennis, tennis, squash, and badminton. In these intermittent and dual activities, performance may be limited as a match progresses because of a reduced central activation, linked to changes in neurotransmitter concentration or in response to afferent sensory feedback. Alternatively, modulation of spinal loop properties may occur because of changes in metabolic or mechanical properties within the muscle. Finally, increased fatigue manifested by mistimed strokes, lower speed, and altered on-court movements may be caused by ionic disturbances and impairments in excitation-contraction coupling properties. These alterations in neuromuscular function contribute to decrease in racquet sports performance observed under fatigue.
This article describes the physiologic and neural mechanisms that cause neuromuscular fatigue in racquet sports; table tennis, tennis, squash and badminton. In these intermittent and dual activities, performance may be limited as a match progresses because of a reduced central activation, linked to changes in neurotransmitters concentration or in response to afferent sensory feedback. Alternatively, modulation of spinal loop properties may occur because of changes in metabolic or mechanical properties within the muscle. Finally, increased fatigue manifested by mistimed strokes, lower speed, and altered on-court movements may be caused by ionic disturbances and impairments in excitation-contraction coupling properties. These alterations in neuromuscular function contribute to decrease in racquet sports performance observed under fatigue.
Technical characteristics and physiologic demands of racquet sports
Badminton, squash, and table tennis are among the most popular racquet sports, even if tennis is probably the most widely practiced. Before discussing the potential mechanisms that limit performance, the technical characteristics of these sports and the physiologic strain imposed on the players have to be described. In racquet sports, the activity pattern is intermittent; that is, characterized by repetitions of fast starts and stops and alternating brief periods of exercise at maximal or near maximal intensity, and longer periods of lower intensity. Performance arises from complex interaction between technical, tactical, physiologic, and psychologic skills that often have to be sustained in hostile environmental conditions. To successfully endure tournament competition, racquet sports players must accelerate, decelerate, change direction, move quickly, maintain balance, and repeatedly generate optimum stroke production. This physiologic strain is influenced by hydration and nutritional status. The duration of competition in racquet sports can vary from 30 to 60 minutes in squash and badminton to more than 5 hours in tennis, but average durations of 30 to 90 minutes are common in all racquet sports. In most high-level matches, the rallies last on average between 2 and 15 seconds and the work-to-rest ratio varies between 1.1 and 1.5. Nevertheless, match activity varies widely across racquet sports. In tennis, the mean durations of rally and resting periods are approximately 4 to 8 seconds and 15 to 20 seconds, respectively. The average effective playing time ranges usually between 10% and 30% of the game duration. In squash, the point duration is longer (10–20 seconds) and the resting period is shorter (7–8 seconds); so the effective playing time is 50% to 70% of game duration. A summary of the results of several notational analyses of racquet sports is presented in Table 1 .
Activity | PD (s) | RD (s) | W:R ratio | EPT (%) | References |
---|---|---|---|---|---|
Table tennis | 3–4 | 8 | 1:3 | 35 | |
Tennis | 5–12 | 15–20 | 1:4 | 20–30 | |
Badminton | 4–8 | 10–16 | 1:2 | 40–50 | |
Squash | 15–20 | 8–10 | 1:1 | 50–70 |
Research on the physiologic demands of racquet sports indicate that these sports place considerable demands on both aerobic and anaerobic pathways, but their relative contributions are controversial. Estimates of exercise intensity (oxygen uptake, heart rate, or blood lactate concentrations) are described in Table 2 . In racquet sports, the physiologic demand may vary to a large extent, and is influenced by a multitude of factors such as the style of the player, gender, the level and style of the opponent, the surface, the equipment (ie, missile and racquet characteristics), and environmental factors (ie, temperature and humidity).
Activity | %VO 2max | %HR max | [La] (mmol.l −1 ) | References |
---|---|---|---|---|
Table tennis | – | 80–85 | 2 | |
Tennis | 60–80 | 70–85 | 2–4 | |
Badminton | 75–85 | 75–90 | 3–6 | |
Squash | 80–85 | 85–92 | 6–10 |
Cardiorespiratory fitness has been traditionally measured by maximal oxygen uptake. Racquet sports players possess moderate (table tennis: ∼50 mL.min −1 .kg −1 ; tennis: ∼50–55 mL.min −1 .kg −1 ) to high (badminton: ∼55 mL.min −1 .kg −1 ; squash: >60 mL.min −1 .kg −1 ) aerobic capabilities, similar to those of team-sports players but obviously lower than those of endurance athletes. However, an elevated cardiovascular endurance is a prerequisite attribute to compete at the elite level (ie, fast recovery between points) in all racquet sports but table tennis. Flexibility, muscular endurance, strength, and power, as well as more specific factors such as acceleration, agility, balance, and response time have also been described as important physical factors in racquet sports. At the elite level, effective stroke production requires rapid on-court movements, explosive force, and the capacity to generate explosive bursts of power. As a consequence, success in the decisive rallies at the end of a long and demanding match can be determined by the ability to repeatedly perform sprints and generate effective powerful strokes. Therefore an important issue is to describe and understand how fatigue limits performance in racquet sports.
Manifestation of fatigue
A close inspection of the literature reveals that the effects of fatigue on performance in squash, badminton, or table tennis players have received little documentation. During the last decades, several studies have provided scientific evidence to support the observations made by coaches that fatigue impairs performance, as shown by mistimed shots (ie, power and precision) and altered on-court movements (ie, speed, positioning to the ball). In tennis, physiologic perturbations during training and simulated matches and their combined effects on a number of performance variables have been reviewed. Fatigue-inducing protocols have been recently developed to determine the effects of fatigue on stroke production in specific conditions close to those of competition. These studies have reported conflicting results regarding change in stroke velocity and accuracy. For example, Davey and colleagues observed a large decrease in the accuracy of shots played (∼69% and 30% for ground stroke and serve, respectively) during an exhausting tennis simulation test, whereas accuracy was only slightly reduced (ground stroke) or unchanged (serve) after a 2-hour, on-court strenuous training session. However, the lack of sensitivity and the large variability in selected variables limit considerably the generalization of these findings. Another shortcoming is that fatigue levels experienced by players failed to reflect those recorded in match play (ie, format of the protocol, using a ball machine to administer pre- and post-fatigue on-court skill assessment; Ref. ). For example, it is questionable how an intermittent test leading to volitional exhaustion in approximately 35 minutes could induce a degree of physiologic strain comparable to that of actual competition. These limitations have guided investigators to evaluate the effects of fatigue on performance during simulated match conditions. For example, Mitchell and colleagues have reported that fatigue after a 3-hour tennis match is manifested by a decreased velocity of the serve and longer time to complete tennis pattern shuttle-runs. Using a similar approach, Girard and colleagues recently reported progressive reductions in maximal voluntary strength (∼10%–13% in quadriceps) and leg stiffness highly correlated with increases in perceived exertion and muscle soreness throughout a 3-hour tennis match, whereas explosive strength was maintained and decreased only after the exercise. As recently outlined by Hornery and colleagues, these studies are also limited by a lack of knowledge regarding mechanisms underlying potential fatigue. Finally, it is important to keep in mind that several factors, including the environmental conditions (hot/humid), dehydration status, and intake of carbohydrate and caffeine, have been shown to affect racquet sports performance and therefore the extent of muscle fatigue experienced by players.
Manifestation of fatigue
A close inspection of the literature reveals that the effects of fatigue on performance in squash, badminton, or table tennis players have received little documentation. During the last decades, several studies have provided scientific evidence to support the observations made by coaches that fatigue impairs performance, as shown by mistimed shots (ie, power and precision) and altered on-court movements (ie, speed, positioning to the ball). In tennis, physiologic perturbations during training and simulated matches and their combined effects on a number of performance variables have been reviewed. Fatigue-inducing protocols have been recently developed to determine the effects of fatigue on stroke production in specific conditions close to those of competition. These studies have reported conflicting results regarding change in stroke velocity and accuracy. For example, Davey and colleagues observed a large decrease in the accuracy of shots played (∼69% and 30% for ground stroke and serve, respectively) during an exhausting tennis simulation test, whereas accuracy was only slightly reduced (ground stroke) or unchanged (serve) after a 2-hour, on-court strenuous training session. However, the lack of sensitivity and the large variability in selected variables limit considerably the generalization of these findings. Another shortcoming is that fatigue levels experienced by players failed to reflect those recorded in match play (ie, format of the protocol, using a ball machine to administer pre- and post-fatigue on-court skill assessment; Ref. ). For example, it is questionable how an intermittent test leading to volitional exhaustion in approximately 35 minutes could induce a degree of physiologic strain comparable to that of actual competition. These limitations have guided investigators to evaluate the effects of fatigue on performance during simulated match conditions. For example, Mitchell and colleagues have reported that fatigue after a 3-hour tennis match is manifested by a decreased velocity of the serve and longer time to complete tennis pattern shuttle-runs. Using a similar approach, Girard and colleagues recently reported progressive reductions in maximal voluntary strength (∼10%–13% in quadriceps) and leg stiffness highly correlated with increases in perceived exertion and muscle soreness throughout a 3-hour tennis match, whereas explosive strength was maintained and decreased only after the exercise. As recently outlined by Hornery and colleagues, these studies are also limited by a lack of knowledge regarding mechanisms underlying potential fatigue. Finally, it is important to keep in mind that several factors, including the environmental conditions (hot/humid), dehydration status, and intake of carbohydrate and caffeine, have been shown to affect racquet sports performance and therefore the extent of muscle fatigue experienced by players.
Defining and quantifying neuromuscular fatigue
Fatigue is a complex phenomenon and has been a major research topic for exercise scientists for the last half century. Neuromuscular fatigue refers to a transient reduction in the maximal force capacity of the muscle, and is measured objectively by an acute reduction of performance during exercise. The inability to produce and maintain the required force can be attributed to several potential mechanisms occurring within cortical regions to muscular contractile elements, with each of these stages as a possible limiting factor. Traditionally, studies of neuromuscular fatigue during exercise have focused on alteration in the recruitment of motor units or muscles themselves. In reality, it is best to use the concepts of central fatigue (ie, an exercise-induced decrease of muscle force caused by a reduction in recruitment) and peripheral fatigue (ie, decrease in force caused by a decrease in muscle fiber contractility induced predominantly by metabolic events within the muscle). However, it is not within the scope of this article to describe in detail the central and peripheral aspects of fatigue. The reader is referred to the excellent reviews of Gandevia and Fitts.
Reliable assessment of neuromuscular fatigue can be studied in humans using percutaneous stimulation of nerve or muscle, although this method sometimes causes discomfort. This method usually associates electromyography (EMG) and measurements of voluntary and evoked forces. It is possible to superimpose an electrical or magnetic stimulation on the motor nerve (ie, tibial nerve for the soleus muscle) or to perform transcranial magnetic stimulations to evaluate the level of central fatigue of a subject. In short, the twitch superimposed to a maximal voluntary contraction (MVC) is compared with the twitch evoked on the relaxed muscle in order to calculate the level of voluntary activation (twitch interpolation technique Ref. ). If the electrical stimulus elicits an increase in force greater than MVC, then there is reduced motor drive and central fatigue has occurred. Electrical or magnetic stimulation can also be evoked on the relaxed muscle to explore peripheral fatigue (ie, amplitude of the mechanical response). By analyzing the changes in the integrated electromyographic signal (iEMG), or root mean square (RMS), and the compound action potential (M-wave) during voluntary and evoked contractions, respectively, EMG can also be considered as an acceptable noninvasive method of measuring neuromuscular fatigue. With such indices it is possible to clarify whether a decrease in MVC force is completely attributable to the loss of muscle contractile properties, or whether central drive may contribute to this decrease. This method has been applied to isometric contractions more frequently than to dynamic ones of muscles groups of both upper and lower limbs. In addition, peak MVC force is commonly assessed in humans to characterize fatigue, probably because it is an easily measurable variable under relatively standardized conditions, which ultimately allow valuable comparisons between studies. In racquet sports, one issue to be resolved when assessing functional impairment of fatigued muscles is that the dynamic parameters such as the rate of force development, usually analyzed in other sports, does not reflect the specific fatigue that is induced by repeated accelerations-decelerations of brief durations in order to reach maximum velocity. Therefore one may underestimate the functional muscle impairment (ie, ability to generate fast movements) by analyzing force parameters only. Furthermore, the large variability in the contraction types, the muscle groups tested, and the testing protocols (ie, nature of the exercise, ergometers, fatigue variables) complicate the interpretation of mechanisms underlying neuromuscular fatigue for a given task. However, a good reliability of measurements used for characterizing central (ie, voluntary activation level or EMG) and peripheral (ie, M-wave properties or peak twitch and peak doublet torques) fatigue was recently described by Place and colleagues.
It is well-established that the magnitude and the etiology of fatigue depend on the exercise (task-dependency). During racquet play, fatigue develops as the duration and intensity of physical exertion increase. Transient episodes of muscular fatigue generally occur after several consecutive intense rallies. As a result of this combination of repeated sprints and the prolonged pattern of exercise, the ability to maintain exercise intensity in racquet sports represents a unique challenge. During real competitions the changes in the sequence of movement are complex and unpredictable, and are widely affected by the level and game of the opponent, so this variability imposes unique physiologic demands. Therefore, it is likely that the mechanisms underlying fatigue are different among the different racquet games.
The neuromuscular adaptation to fatigue has been widely studied in conditions of voluntary isometric muscle actions (ie, Duchateau and colleagues ) and electrical stimulation (ie, Boerio and colleagues ). Much less is known, however, about the effects of real exercise such as racquet games on the neuromuscular function. This lack of direct scientific information on racquet games-specific fatigue is only partly compensated for by interpretation of data from laboratory studies or of other intermittent activities (ie, team sports). The objective of the next two sections is to carry out an integrative review of the available literature on possible causes (peripheral and central) limiting muscular performance during racquet sports competitions.
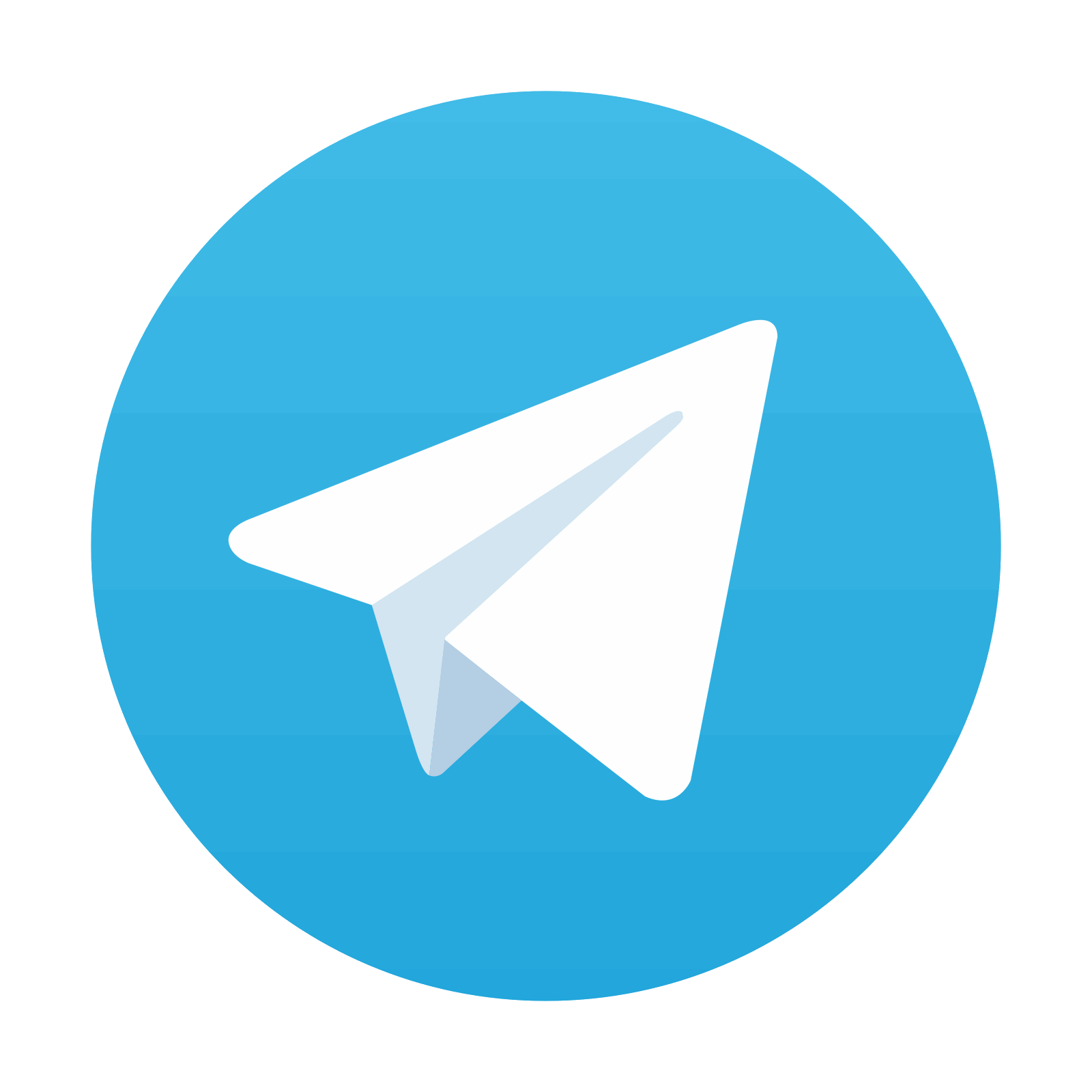
Stay updated, free articles. Join our Telegram channel

Full access? Get Clinical Tree
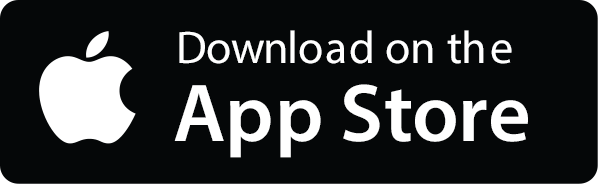
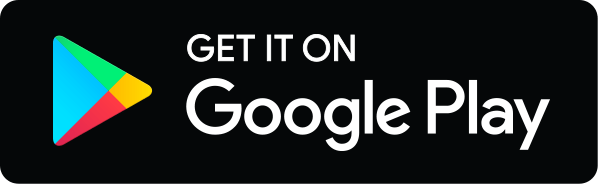