Microfracture
J. Richard Steadman
Trauma to the articular cartilage of the knee is a common result of recreational and elite sport injuries. The poor capacity of articular cartilage to heal itself makes the treatment of these lesions challenging.1 The microfracture technique (Fig. 9.1) has been shown to be an effective arthroscopic treatment for full-thickness chondral lesions of the knee.2,3 This technique is cost-effective, not technically complicated, has an extremely low rate of associated patient morbidity, and does not present any hindrances to further treatment. Microfracture does not replace tissue; rather, it should be considered tissue repair. This technique relies on a “marrow-based” strategy to foster tissue repair. In order for tissue to regenerate, cells must be present. The creation of controlled “microfractures” through the subchondral bone promotes the release of marrow-based mesenchymal stem cells and growth factors (Fig. 9.2). A marrow clot is formed at the base of a prepared chondral lesion (Fig. 9.3). These cells proliferate and differentiate into cells with morphologic features of chondrocytes, and produce a cartilaginous repair tissue that fills the chondral defect.
Basic Science of Articular Cartilage Injury
In a single event, the shearing forces of the femur on the tibia may result in trauma to the articular cartilage, causing the cartilage to fracture, lacerate, and separate from the underlying subchondral bone, or separate along with a piece of the subchondral bone (Fig. 9.4).1,4,5 The articular surface may also fatigue and fail as a result of chronic repetitive loading beyond the tolerance of normal physiological levels. Younger patients usually experience a single event that leads to acute cartilage lesions (Fig. 9.5), whereas chronic degenerative lesions (Fig. 9.6) are seen in middle-aged and older members of the population.1,4,5,6 Repetitive impacts can cause cartilage swelling, an increase in collagen
fiber diameter, and an alteration in the relationship between collagen and proteoglycans.1,5 Thus, acute events may not result in full-thickness cartilage loss, but rather start a degenerative cascade that can lead to chronic full-thickness loss (Fig. 9.7A–D). The degenerative cascade typically includes early softening and fibrillation (grade I); fissures and cracks in the surface of the cartilage (grade II); severe fissures and cracks with a “crab meat” appearance (grade III); and, finally, exposure of the subchondral bone (grade IV).1,4,5,6
fiber diameter, and an alteration in the relationship between collagen and proteoglycans.1,5 Thus, acute events may not result in full-thickness cartilage loss, but rather start a degenerative cascade that can lead to chronic full-thickness loss (Fig. 9.7A–D). The degenerative cascade typically includes early softening and fibrillation (grade I); fissures and cracks in the surface of the cartilage (grade II); severe fissures and cracks with a “crab meat” appearance (grade III); and, finally, exposure of the subchondral bone (grade IV).1,4,5,6
![]() Figure 9.1. A microfracture awl creating microfractures in a full-thickness chondral defect of the femoral condyle of the knee. |
Biological and Physiological Backgrounds
Articular cartilage is maintained by the balanced coupling of the anabolic and catabolic processes of chondrocytes. Much research has focused on defining the mechanism that regulates the anabolic/catabolic homeostasis seen in normal cartilage. In a degenerative process, matrix resorption is accelerated, causing degradation that outpaces the anabolic attempt of the chondrocyte to produce new matrix. Correcting this imbalance in cartilage metabolism or promoting the healing of damaged cartilage is necessary to stop the cascade of the degenerative lesion.
![]() Figure 9.2. Marrow elements, which may contain stem cells and growth factors, are released from the marrow cavities formed by the microfractures. |
![]() Figure 9.3. A marrow clot, or “super clot” as we refer to it, is formed at the base of the lesion following microfracture. |
Various polypeptide mediators, such as transforming growth factor-β, insulinlike growth factor-1 (IGF-1), and basic fibroblast growth factor regulate the metabolic balance between anabolism and catabolism of articular cartilage.7 Transforming growth factor-β has been shown to
stimulate the synthesis rate of matrix macromolecules, especially small glycosaminoglycans, and promote the repair of damaged cartilage.8,9 IGF-1 can stimulate chondrocyte proliferation and the synthesis of aggrecan and hyaluronic acid.10,11,12 IGF-1 can also decrease cartilage degradation by antagonizing interleukin-1 action.13 Basic fibroblast growth factor is a potent mitogenic agent for chondrocytes and can stimulate chondrocytes to synthesize a cartilaginous matrix.14 These results indicate a potential therapeutic application of exogenetic growth factors to enhance the healing of damaged cartilage.15
stimulate the synthesis rate of matrix macromolecules, especially small glycosaminoglycans, and promote the repair of damaged cartilage.8,9 IGF-1 can stimulate chondrocyte proliferation and the synthesis of aggrecan and hyaluronic acid.10,11,12 IGF-1 can also decrease cartilage degradation by antagonizing interleukin-1 action.13 Basic fibroblast growth factor is a potent mitogenic agent for chondrocytes and can stimulate chondrocytes to synthesize a cartilaginous matrix.14 These results indicate a potential therapeutic application of exogenetic growth factors to enhance the healing of damaged cartilage.15
![]() Figure 9.4. Illustration demonstrating fracture, laceration, and separation of articular cartilage following trauma. |
Chondrocytes may also respond to physical stimulation. Studies have shown that mechanical force can regulate the metabolic response of articular cartilage.16,17,18,19 Immobilization or reduced loading of the joint was shown to result in a decrease in proteoglycan synthesis, whereas moderate exercise led to an increase in proteoglycan synthesis16 and thickening of the cartilage matrix.17 Severe mechanical loading resulted in a thinning of cartilage matrix and led to degenerative changes.19 Dynamic loading has also been shown to affect glycosaminoglycan and DNA synthesis.18
Basic Science of Microfracture
Several animal studies have been done to assess the microfracture technique. In our experience, the equine model is the best model to use for cartilage research. The horse-human relationship research is described in Chapter 16.
Equine articular cartilage is similar in thickness to that of humans. In the horse, procedures (including relooks) can be done arthroscopically. The horse joint undergoes realistic biomechanical forces during gait, and its rehabilitation can also be controlled. Swimming allows horses to avoid full weightbearing exercise, and the treadmill can be used to control the intensity and duration of activity. The fact that horses must be immediately weightbearing following surgery is a disadvantage. Although partial weightbearing is impossible for the horse, this limitation provides an even more challenging environment in which to test cartilage repair. After surgery, the
antalgic quadruped gait provides some protection for the area of microfracture.
antalgic quadruped gait provides some protection for the area of microfracture.
The first study on microfracture in the horse was to determine if microfracture produced more repair tissue than an untreated lesion.20 Large chondral defects were created in the radial carpal bones and in both medial femoral condyles of the horses. One carpal bone and one femoral condyle of each horse were treated with microfracture and the others were left untreated. In five horses at 4 months, and five horses at 12 months, gross, histologic, and histomorphometric examinations of defect sites and repair tissues were performed. The repair tissues were also evaluated for collagen typing. The results showed a significant amount of repair tissue in the defects that were treated with microfracture (Fig. 9.8). An increase in Type II collagen (Fig. 9.9) and earlier bone remodeling, as documented by changes in porosity, was also seen in the defects treated with microfracture.
On histologic evaluation of these samples, it was noted that the presence of calcified cartilage impeded the growth of repair tissue (Fig. 9.10). This finding led to further analysis of defects treated by removing calcified cartilage and those with calcified cartilage intact. In this study, defects were made in mature horses on the axial weightbearing portion of both medial femoral condyles.21 The calcified cartilage layer was removed from one defect in each horse. At 4 months and 12 months, removal of calcified cartilage resulted in improved grade of overall repair tissue and increased histological filling of the defect. The study concluded that removal of calcified cartilage layers provided optimal amount and attachment of repair tissue. The results of this study led to changes in the technique. Removal of the calcified cartilage was determined to be an important step in the microfracture procedure (Fig. 9.11).
Another study in the horse was performed to assess key matrix component expression in early cartilage healing
with microfracture.22 Microfracture and control samples were collected at 2, 4, 6, and 8 weeks. Analyses included determining qualitative impression of cellular and molecular changes. Comparisons of histomorphometric data and molecular and protein expression of critical cartilage components were performed at 8 weeks. The results demonstrated a gradual and significant increase in mRNA content for both Type II collagen and aggrecan over the 8-week period (Fig. 9.12). The Type II collagen expression was enhanced with microfracture. This enhancement of Type II collagen protein after microfracture was supported by the previous long-term study.20 In this study, aggrecan expression did not appear to be influenced by microfracture treatment, whereas another critical matrix component was enhanced (Type II collagen).
with microfracture.22 Microfracture and control samples were collected at 2, 4, 6, and 8 weeks. Analyses included determining qualitative impression of cellular and molecular changes. Comparisons of histomorphometric data and molecular and protein expression of critical cartilage components were performed at 8 weeks. The results demonstrated a gradual and significant increase in mRNA content for both Type II collagen and aggrecan over the 8-week period (Fig. 9.12). The Type II collagen expression was enhanced with microfracture. This enhancement of Type II collagen protein after microfracture was supported by the previous long-term study.20 In this study, aggrecan expression did not appear to be influenced by microfracture treatment, whereas another critical matrix component was enhanced (Type II collagen).
The study by Frisbie et al.22 confirmed that microfracture significantly increases Type II collagen expression as early as 8 weeks after treatment (Fig. 9.13). However, microfracture did not alter other key components of the matrix (aggrecan and Type I collagen). The quest for additional improvement in repair tissue character after the use of this technique continues.
The Acute Chondral Injury
Indications and Contraindications
General indications for microfracture include full-thickness defect, unstable cartilage that overlies the subchondral bone, and a partial-thickness lesion in which cartilage simply scrapes off the bone when probed. Other considerations for use of the microfracture procedure include the patient’s age, acceptable biomechanical alignment of the knee, activity level, his or her willingness to accept rehabilitation protocol, and the individual’s expectations (Fig. 9.14).3,23,24,25 If all of these criteria are met, microfracture should be considered as a treatment option.
Patient age is not a specific contraindication. Our studies have shown that patients younger than 35 years of age with acute lesions have greater improvement, while older patients show some improvement. The size of the lesion is also not a contraindication for microfracture.2,3 In previous studies, we have shown that large acute lesions respond well to microfracture; however, lesions less than 400 mm2 (Fig. 9.15) tend to respond better to microfracture than those lesions greater than 400 mm2, but we have not observed this difference to be statistically significant.2,3
Specific contraindications for microfracture include axial malalignment (Fig. 9.16; as described in Chapter 13), patients unwilling or unable to follow the required strict and rigorous rehabilitation protocol, partial-thickness
defects, and inability to use the opposite leg for weightbearing during the minimal or nonweightbearing period.2,26
defects, and inability to use the opposite leg for weightbearing during the minimal or nonweightbearing period.2,26
Preoperative Planning
Initial evaluations of patients who present with knee joint pain include a thorough physical and orthopaedic examination, as well as an evaluation of their symptoms. These symptoms may include pain, swelling, stiffness, and mechanical symptoms. It is important on the initial evaluation to determine the patient’s activity level and expectations. Identification of point tenderness over a femoral condyle or tibial plateau is a useful finding, but in itself is not diagnostic. If compression of the patella (Fig. 9.17) elicits pain, this finding may be indicative of a patellar or trochlear lesion. At times, physical diagnosis can be difficult and elusive, especially if only an isolated chondral defect is present.
Imaging
We use long standing radiographs to determine angular deformity and joint-space narrowing, which is often indicative of articular cartilage loss (Fig. 9.18). We also obtain standard anteroposterior and lateral radiographs of both knees, as well as weightbearing views with the knees flexed to 30 or 45 degrees (Fig. 9.19). Patellar views are also useful to evaluate the patellofemoral joint. Magnetic resonance imaging, which employs newer diagnostic
sequences specific for articular cartilage, has become a mainstay of our diagnostic workup of patients with suspected chondral lesions (Fig. 9.20). Imaging is described in detail in Chapter 7.
sequences specific for articular cartilage, has become a mainstay of our diagnostic workup of patients with suspected chondral lesions (Fig. 9.20). Imaging is described in detail in Chapter 7.
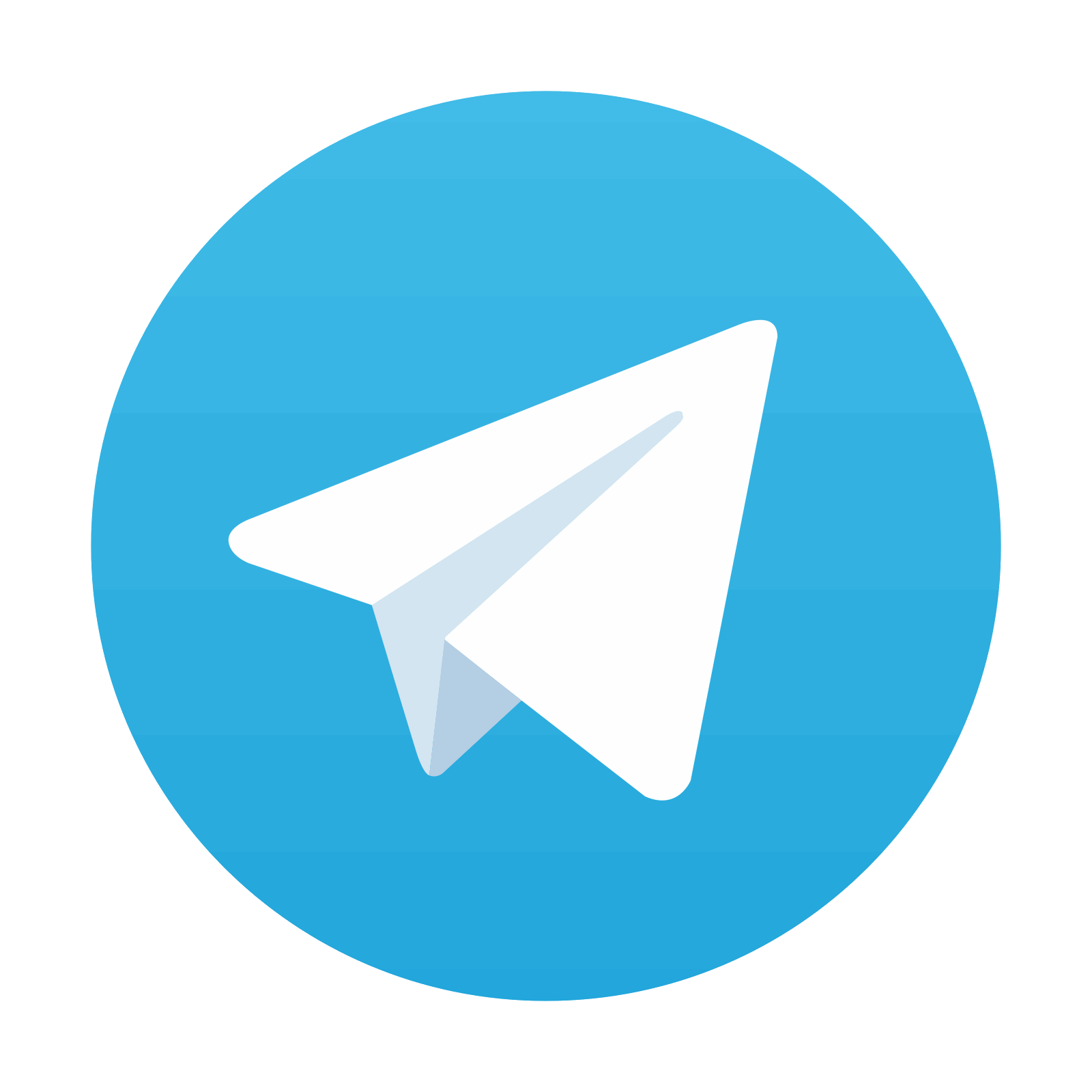
Stay updated, free articles. Join our Telegram channel

Full access? Get Clinical Tree
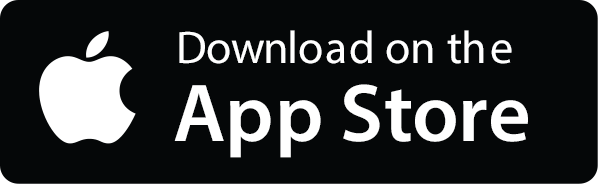
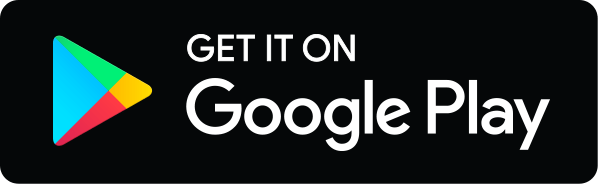