Fig. 6.1
The axial skeleton is hypoxic and expresses HIF-1α. (a) Characterization of hypoxia- inducible reporter mice (5XHRE-LacZ reporter). Note activation of the reporter construct in mesenchymal condensations by β-gal staining (black arrows). (b) Staining with hypoxia marker EF5 confirms that the nucleus pulposus (NP) of the presumptive intervertebral disc is hypoxic (Images kindly provided by Dr. Ernestina Schipani, Indiana University)
Another important environmental factor that characterizes the disc niche is the elevated osmolarity. Although sparse, cells in the nucleus pulposus secrete a complex extracellular matrix that contains collagens and the proteoglycan aggrecan as well as versican. Glycosaminoglycan (GAG) chains of the aggrecan molecule provide a robust hydrodynamic system that serves to accommodate applied biomechanical forces (Feng et al. 2006; Setton and Chen 2006). In the nucleus pulposus, the principle GAG is chondroitin sulfate. Bound to the aggrecan core protein and associated with hyaluronic acid, the chondroitin sulfate chains form a giant polydispersed supramolecular structure with a net negative charge; this serves as a driving force for binding cations especially Na+, thereby elevating tissue osmolarity (this topic is discussed further in Chaps. 2 and 4). The high osmotic pressure of the aggregate contains the forces applied to the spine (Ng et al. 2003). We have shown that nucleus pulposus cells responded to changes in osmotic pressure by upregulating the transcription factor, TonEBP (tonicity enhancer-binding protein) (Tsai et al. 2006), the only known mammalian transcription factor that responds to changes in osmolarity. In the following sections, we will describe in detail the activities of this interesting protein and its importance in maintenance of disc cell function. In addition to osmolarity and hypoxia, several other morphogenic proteins including those of the TGF-β superfamily play an important role in niche maintenance. Towards the end of the chapter, the activities of TGF-β in the postnatal nucleus pulposus are briefly discussed.
6.2 Role of HIF Proteins in the Intervertebral Disc Niche
If the concept of a regulatory niche, composed of a number of cell types responsive to local microenvironmental conditions, is valid, then this begs the question: is cell survival in the hypoxic niche hypoxia-inducible factor (HIF) dependent? Thanks to the brilliant studies of Semenza and colleagues, it is now recognized that the critical molecule regulating energy metabolism and survival activity under hypoxia is HIF-1 (Semenza et al. 1994). HIF is a member of the basic helix–loop–helix (bHLH)–PER–ARNT–SIM (PAS) family of proteins and composed of a constitutively expressed β-subunit and an α-subunit. In most cells, the latter subunit is stable under hypoxic conditions but is rapidly degraded in normoxia (Wang et al. 1995). Transactivation of HIF-1 target genes involves dimerization of the two subunits and binding to an enhancer, the hypoxia-response element in target genes. HIF-1 serves as a key transcription factor that regulates the expression of enzymes concerned with glycolysis, the activity of the TCA cycle, and oxidative phosphorylation (Semenza et al. 1994; Papandreou et al. 2006; Fukuda et al. 2007). Additional target genes include those required for survival, apoptosis, autophagy, and matrix synthesis (Schipani et al. 2001; Zhang et al. 2008; Hofbauer et al. 2003). Details of these relationships are shown schematically in Fig. 6.2. It should be added that other isoforms exist, the most important being HIF-2α. Recent evidence suggests that HIF-1α and HIF-2α are not redundant and that the relative importance of each of the homologues, in response to hypoxia, varies among different cell types (Sowter et al. 2003). For example, unlike HIF-1, HIF-2 regulates expression of a number of unique genes including superoxide dismutase 2 (SOD2), catalase, frataxin, and cited2 (Scortegagna et al. 2003; Oktay et al. 2007; Aprelikova et al. 2006).
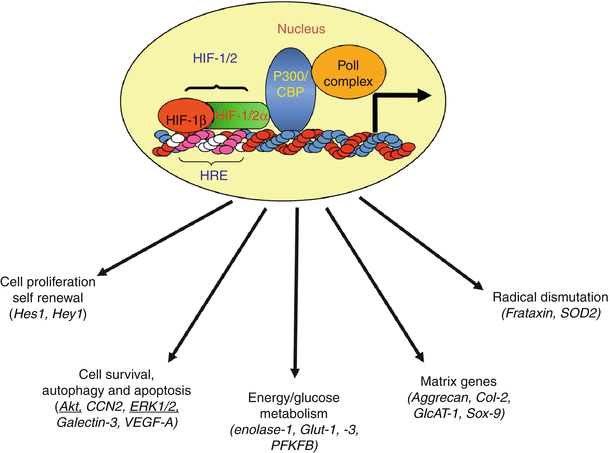
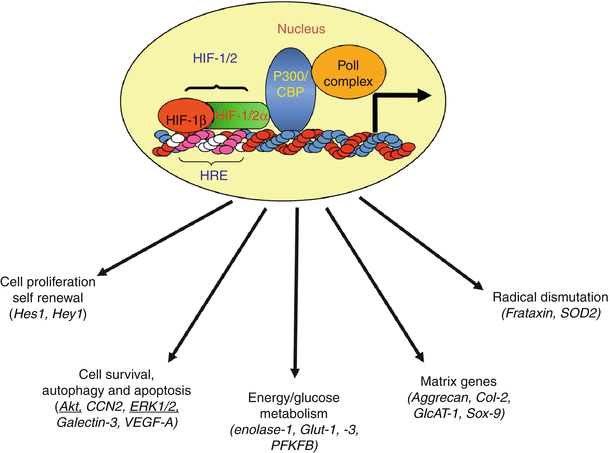
Fig. 6.2
Functional activity of HIF target genes. Critical functions include energy metabolism, angiogenesis, cell survival, autophagy and apoptosis, matrix synthesis, proliferation, self-renewal and differentiation, radical dismutation, and pH regulation. Many of these functions are critical for survival and functioning of the nucleus pulposus cells in the avascular niche of the intervertebral disc. Hypoxia-/HIF-sensitive proteins that are identified in the nucleus pulposus cells are shown in parentheses (Reproduced from Risbud et al. (2010). With permission from Elsevier)
In addition to the genes mentioned above, the Sox family of transcription factors that are essential for the development and function of the nucleus pulposus are hypoxia and HIF sensitive (Smits and Lefebvre 2003; Lafont et al. 2007; Khan et al. 2007; Kanichai et al. 2008). Lafont et al. (2007) showed that HIF-2, but not HIF-1, regulated the expression of Sox9 and the phenotype of primary human chondrocytes. Similarly, expression of Sox9, Sox5, and Sox6 is hypoxia- (5 % O2) and HIF-2-sensitive during chondrogenic differentiation of stem cells derived from the infrapatellar fat pads of osteoarthritic patients (Khan et al. 2007). In contrast, using marrow mesenchymal stem cells, Kanichai et al. (2008) showed involvement of HIF-1α in regulating Sox9 expression during chondrogenesis under hypoxia. However, the relationship between Sox proteins and HIF in the hypoxic niche of the disc is not as yet known (Box6.1).
6.2.1 Box 6.1: Oxygen, Hypoxia and HIF
Over a billion years ago, the primitive atmosphere of the earth held very small amounts of dioxygen. Through the extraordinary activities of photosynthetic plants and organisms, over an enormous time period, the level of oxygen increased rapidly: a mere 200 million years ago, the oxygen content increased to about 16 %. Today, despite a huge elevation in manmade and natural oxidative activities, the gas level has held constant around 21 %.
The life-supporting activities of the gas was recognized by the Greeks who postulated that there were only four natural elements, air – along with earth, water, and fire. At the time of the American Revolution, there was a convergence of intellectual thought on the notion that air was a mixture of gases, especially the vital gas, oxygen. Among those charged with the discovery of oxygen were Joseph Priestley, Carl Wilhelm Scheele, and Antoine Lavoisier. Priestley, an Englishman, who for religious and political reasons settled in Pennsylvania, and was a good friend of Benjamin Franklin, found that a gas generated from metal oxides could keep a mouse alive longer than a similar volume of air. Priestley called his discovery “dephlogisticated air.” Almost at the same time, Scheele isolated the same gas which he termed “fire air.” A Frenchman, Antoine Lavoisier, labeled the gas “acid-maker” or “oxygen,” viewing it as part of the air that was free to combine with other elements. Of note, Priestly found that the “dephlogisticated air” had the property of changing dark venous blood to bright red arterial blood.
We now know that red blood cells carry dioxygen to all of the tissues of the body so as to power metabolic reactions, particularly those concerned with maintenance of redox and high-energy intermediates such as ATP. Oxygen sensor systems exist within tissues: for example, in the carotid body and in mitochondria. A family of enzymes, prolyl hydroxylases, monitors the oxemic state of the cell and enhances adaptation to hyperoxic and hypoxic conditions.
The term hypoxia is a generalized term used to describe the fall in oxygen to levels that are necessary to sustain most animal life. In some tissues, the blood oxygen concentration can be normoxic, but the cells experience hypoxia due to a high cell density, elevated metabolic activity, or poor vascularization. If hypoxia develops, a family of transcription factors is activated. Hypoxia-inducible factors or HIFs serve to change the metabolic activities of the cell so as to accommodate the available oxygen concentration.
6.2.2 Control of HIF-α Stability in the Disc
To return to the question raised above concerning the importance of the HIF system, a considerable number of reports now clearly show that there is a robust HIF response by cells of the nucleus pulposus. The response is evident across species; it is seen in vivo and in vitro, and more importantly, HIF-1α activity is unresponsive to the oxemic state of the tissue (Rajpurohit et al. 2002; Risbud et al. 2006a, b; Agrawal et al. 2007). Accordingly, when compared with most other tissues, there are substantive underlying differences in the HIF status and reactivity of disc cells: HIF-1α expression and activity is always “on.” This unusual response suggests that stabilization of HIF-1α in cells of the nucleus pulposus ensures that transcriptional activity is a major determinant of cell function. The second HIF homologue, HIF-2α, is robustly expressed by nucleus pulposus cells. Like HIF-1α, steady-state protein levels are similar in both hypoxia and normoxia, suggesting that it too is constitutively expressed (Agrawal et al. 2008).
Before leaving this topic, it is important to comment on mechanisms of stabilization and turnover of HIF-1a and HIF-2a in nucleus pulposus cells. HIF-1a can be stabilized in a number of different ways. For example, von Hippel-Lindau protein activity can be suppressed, or O2 sensing by one or more of the prolyl hydroxylase (PHD) enzymes, members of the 2-oxoglutarate/Fe2+-dependent dioxygenase superfamily, could be low (Appelhoff et al. 2004). Importantly, since the activity of PHDs depends on the tissue oxygen tension, these molecules serve as sensors that control the cellular abundance of HIF-a proteins. It is known that PHD proteins hydroxylate specific prolyl residues in the oxygen-dependent degradation domain of HIF-a subunits. The hydroxylated proteins are bound by the ubiquitin ligase von Hippel–Lindau tumor suppressor protein (pVHL), which targets them for rapid ubiquitination and 26S proteasomal degradation (Maxwell et al. 1999). We reported recently that expression of PHD1-3 is higher in cells of the nucleus pulposus than in cells of the annulus fibrosus (Fujita et al. 2012a). Noteworthy, unlike other cells, our studies clearly showed that in nucleus pulposus cells, stability of HIF-a-oxygen-dependent degradation domain (ODD) is independent of oxemic tension. In addition, mutagenesis studies suggest that hydroxylation reaction may not control HIF-2a degradation in these cells (Köditz et al. 2007; Fujita et al. 2012a). Again these findings are different from articular chondrocytes that exhibit responsiveness of HIF-2a degradation to PHD function in vitro suggesting a cell type-specific response (Thoms and Murphy 2010).
Further investigations found that both HIF-1α and HIF-2α were degraded through the 26S proteasome pathway. Since all PHDs mediate proteasomal HIF-α degradation, but differ in their ability to hydroxylate HIF-1α in vivo, we investigated their individual role in HIF-α turnover in nucleus pulposus cells (Minamishima et al. 2008; Takeda et al. 2006). The high relative expression of PHD2 in nucleus pulposus tissue suggests that this isoform may play an important role in HIF-α turnover. These studies indicate that PHD2 controls to a limited extent HIF-1α degradation even under hypoxic conditions, indicating preservation of PHD2 enzymatic activity at low O2 tension. This finding is in marked contrast to previous reports demonstrating HIF-1α stabilization at low oxygen tensions because of inhibition of PHD enzymatic activity (Epstein et al. 2001). Moreover, this observation highlights the unique physiology of the nucleus pulposus cells and suggests that there is very low cellular utilization of O2, an adaptive response to the hypoxic niche (Bibby et al. 2005; Lee et al. 2007). The limited involvement of PHD2 in HIF-1α degradation implies that it is not a major regulator of HIF-1α turnover. Furthermore, it lends support to the hypothesis that HIF-1α levels are regulated primarily by oxygen-independent pathways.
In contrast to HIF-1α, the turnover of HIF-2α through 26S proteasome is largely independent of PHD function; there is also a limited involvement of the lysosomal pathway. Relevant to this discussion, recent studies by Gogate et al. (2012) indicate that Hsp70 modulates HIF-1α protein stability and transcriptional activity. In nucleus pulposus, Hsp70 was shown to interact with HIF-1α under hypoxic conditions and promoted degradation through the proteasomal pathway (Gogate et al. 2012). These findings strongly suggest that nucleus pulposus cells are functionally adapted to their avascular, hypoxic microenvironment and rely mostly on oxygen-independent pathways for controlling HIF-1α and HIF-2α levels. With respect to regulation, in hypoxia, PHD3 promotes HIF-1α transcriptional activity (Fujita et al. 2012b); this observation is in line with a recent report that describes a crucial role of the PHD3–PKM2 complex in enhancing HIF-1α interaction with p300, an important transcriptional coactivator (Luo et al. 2011). The possible pathways involved in HIF-α turnover in nucleus pulposus cells are demarcated in Fig. 6.3.
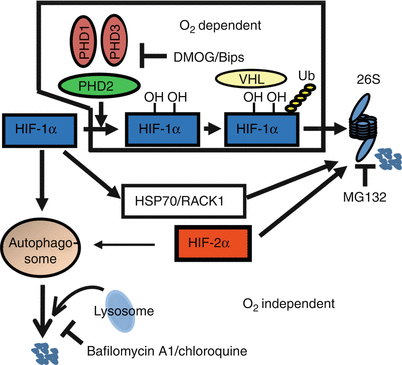
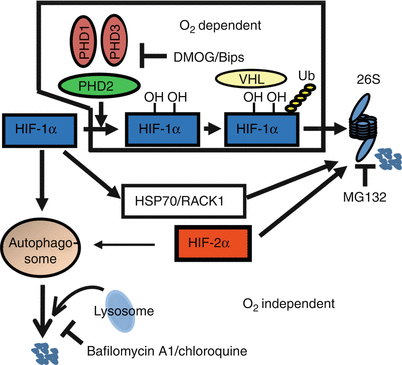
Fig. 6.3
A schematic model of the unique regulation of HIF-1α and HIF-2α degradation in nucleus pulposus cells. PHD2 controls a limited oxygen-dependent degradation of HIF-1α through 26S proteasome pathway. Oxygen-independent mechanisms through 26S proteasome as well as lysosomal pathway are active in HIF-1α turnover. In contrast, HIF-2α is unresponsive to oxidative degradation and is also turned over though 26S and lysosomal pathway (Reproduced from Fujita et al. (2012a). With permission of the American Society for Bone and Mineral Research)
It is important to note that, in nucleus pulposus cells, the expression of PHD2 and PHD3 is also induced by hypoxia in an isoform-specific manner (Fujita et al. 2012b). While PHD2 is selectively regulated by HIF-1α, PHD3 expression is controlled by both HIF-1α and HIF-2α at the transcript level. Significantly when there is inflammatory disc disease, expression of PHD2 and PHD3 is primarily responsive to TNF-α and IL-1β in HIF independent fashion (Fujita et al. 2012c). Moreover, unlike other tissues (D’Angelo et al. 2003; Marxsen et al. 2004; Henze et al. 2010), hypoxic expression of PHD1 is also dependent on HIF-1α activity in nucleus pulposus cells (Fujita et al. 2012b). Taken together, these studies clearly indicate the existence of a regulatory feedback loop between PHD2, PHD3, and HIF-1α in the hypoxic nucleus pulposus cells. This scenario is distinct from the articular cartilage, a tissue functionally similar to the nucleus pulposus, where PHD2 also regulates HIF-2α degradation (Thoms and Murphy 2010). These results highlight the unique nature and control of the HIF-PHD system in nucleus pulposus cells and for the first time provide a biochemical rationale for normoxic stabilization and maintenance of steady-state levels of these proteins in the nucleus pulposus. Whether stabilization of HIF-α proteins is related to the unique embryonic origins of the tissue is currently unknown. However, it is important to note that because discs are hypoxic in vivo, stabilization of HIF-α expression would serve to maintain cell metabolism and functional activities when disc integrity is breached during disc herniation (Ha et al. 2006) or at an early stage of degeneration (Roberts et al. 2006).
6.2.3 Role of HIF-1 in Energy Conservation by Cells of the Nucleus Pulposus
Earlier classical biochemical studies have shown that when the pO2 is low, there is almost complete reliance on glycolysis to generate ATP and reducing equivalents. As indicated in the previous section, one of the consequences of low oxygen tension in the nucleus pulposus is the reliance on glycolysis for energy generation (Agrawal et al. 2007; Holm et al. 1981). Glycolysis may be viewed as a relatively inefficient process: it generates 2 mol of ATP/mol glucose; in contrast, mitochondrial metabolism is slow, but it creates about 30 mol of ATP/mol glucose. In the trade-off between rate and yield, the glycolytic pathway generates a small number of ATP molecules at a very fast rate and maintains the reducing status of the cell. In this way, glycolysis provides sufficient energy for both housekeeping functions and for protein synthesis.
One of the logical outcomes of stabilization of HIF-1α is the robust expression of glucose transporters and enzymes required for anaerobic glycolysis. When the expression of three target genes (glucose transporter [GLUT]-1 and −3 and GAPDH) at 2–21 % O2 were evaluated, it was found that the activities are comparable and remain constant over time (Agrawal et al. 2007). Although these genes were not responsive to the oxemic state of the culture, we have observed a small induction in enolase-1 and phosphofructokinase 2 (PFKFB) promoter activities. This result is surprising as the later protein is regarded as the glycolytic “pacemaker”; however, because this intermediary step is sensitive to a number of hormones, and metabolic intermediates, it is more than likely that induction is in response to other regulatory factors. Nevertheless, the muted response does not detract from the conclusion that the glycolytic flux in disc cells, even in normoxia, is high.
In normoxia, the basal concentration of ATP in nucleus pulposus cells is between 20 and 25 nmol/L/mg protein (Agrawal et al. 2007). These values are comparable with levels reported for articular chondrocytes, another cell type that uses glycolysis to generate energy (Pfander et al. 2003). In the presence of 2-deoxyglucose, a potent inhibitor of glycolysis, ATP generation is suppressed by almost 80 % (Agrawal et al. 2007). The sensitivity of the cells to this inhibitor emphasizes the reliance on glycolysis for energy generation. Based on this observation, it is likely that the oxemic stability of HIF-1α in nucleus pulposus cells is optimal for survival in an environment where there are frequent shifts in vascular supply and O2 delivery; in the intervertebral disc, these shifts may reflect minute to minute or day/night variations in biomechanical forces applied to the spinal units.
Although glycolysis is clearly the major ATP-generating pathway, the possibility exists that some high-energy intermediates may be produced through mitochondrial oxidative phosphorylation. However, current studies indicate that inhibitors of mitochondrial function do not influence ATP production nor nucleus pulposus cell viability (Agrawal et al. 2007). As for a role, if any, for mitochondria, little is known. Gan et al. (2003) have reported that although nucleus pulposus cells contain mitochondria with normal architecture, the total number of organelles per cell is low. Nevertheless, nucleus pulposus cells can perform mitochondrial oxidative metabolism: thus, they oxidize fatty acid and generate ATP (Agrawal et al. 2007). Based on all of these studies, there is strong support for the notion that although glucose and anaerobic glycolysis represent the major fuel and pathway for energy generation, respectively, mitochondria in the nucleus pulposus are functional; they retain the capacity to metabolize fatty acids through mitochondrial oxidative metabolism.
The conclusion that disc cell energy metabolism is dependent on glycolysis fits well with current observations concerning the regulatory functions of HIF-1. It is known that HIF-1 plays a major role in directing the interplay between glycolysis and oxidative phosphorylation. HIF-1 inhibits mitochondrial function by trans-activating the gene encoding pyruvate dehydrogenase kinase 1. Because this protein suppresses pyruvate dehydrogenase, pyruvate cannot be converted into acetyl-CoA, and as a result the TCA cycle is blocked (Papandreou et al. 2006). Moreover Fukuda et al. (2007) showed that HIF-1 reciprocally regulates mitochondrial cytochrome c oxidase (COX)-4 subunit expression by activating transcription of the genes encoding COX4-2 and a protease that is required for COX4-1 degradation. Thus, HIF regulates not just the entry of reducing equivalents into the mitochondria but also oxidative phosphorylation. Based on these observations, it is concluded that although mitochondrial function is retained by cells of the intervertebral disc, it is reasonable to assume that normoxic expression of HIF-1α by nucleus pulposus cells serves to suppress oxidative phosphorylation and promotes glycolytic ATP generation. More than likely, nucleus pulposus mitochondria are required for non-energy-related metabolic functions, while oxidative phosphorylation is used to a very minor degree.
6.2.4 Role of Hypoxia and HIF in Promoting Cell Survival and Function in the Disc
If the premise is correct that the HIF signaling network serves to promote nucleus pulposus function, then the cells should be adapted to survive and grow in a hypoxic environment. Studies from our lab (Risbud et al. 2005a, b; Agrawal et al. 2007; Zeng et al. 2007) and other groups (Mwale et al. 2011; Feng et al. 2013) show that hypoxia, possibly through HIF-1, enhances the expression of important matrix genes and the phenotype of nucleus pulposus cells. Experiments have also been performed in which nucleus pulposus cells were treated with low levels of common apoptogens and survival measured (Risbud et al. 2005a, b). Notably, when the pO2 was below 5 %, there was maximum disc cell survival. Studies of nucleus pulposus and chondrocytes showed that when HIF-1α is partially silenced, viability is maintained in the face of an O2 challenge (Fujita et al. 2012b; Bohensky et al. 2007). On the other hand, complete deletion of HIF-1 in growth plate chondrocytes results in massive cell death highlighting the requirement of HIF-1 for cell survival in the hypoxic niche (Schipani et al. 2001). However, it is possible that some effects of hypoxia on nucleus pulposus survival are probably mediated by other signaling molecules in an HIF-1-independent fashion. The latter observation raises the question: which signaling pathways are upregulated in hypoxia? Work from a number of labs suggests that a variety of hypoxia-responsive proteins exist, including vascular endothelial growth factor (VEGF) (Fujita et al. 2008; Agrawal et al. 2008), galectin-3 (Zeng et al. 2007), and Akt/PI3K (Risbud et al. 2005a, b). Our work has shown that expression levels of phospho-Akt in nucleus pulposus cells are high in hypoxia and when serum-starved confers resistance to apoptosis (Risbud et al. 2005a, b). Activation of this protein is of considerable interest as it has been shown to modulate apoptosis by inactivating Bad and caspase-9 and modulating the transcription of proapoptotic transcription factors (Duronio 2008). Relevant to nucleus pulposus cells, activation of PI3K/Akt signaling has been shown to regulate HIF-1α protein levels in other cell types (Kanichai et al. 2008). Like Akt, extracellular signal-regulated kinase (ERK)1/2 is induced in hypoxic nucleus pulposus cells. Because activation of ERK has been linked to survival, possibly by regulating nitric oxide synthase and caspase activities, the it is probable that activation of ERK in concert with Akt serves to maintain the viability of the disc cells at a low pO2 (Risbud et al. 2005a, b).
Like HIF-1, one of the critical functions of Akt is regulation of glucose metabolism. It is thought that Akt may promote cell survival by maintaining GLUT-1 transcription under conditions of growth factor withdrawal (serum starvation) (Rathmell et al. 2003). Indeed, the high level of expression of GLUT-1 protein by nucleus pulposus cells in vivo (Rajpurohit et al. 2002; Richardson et al. 2008) indicates that this tissue may adapt to its hypoxic environment by increasing glucose uptake. This activity would serve to promote and enhance glycolysis, thereby preventing ischemia-induced injury. Taken together, these studies suggest that the PI3K-Akt and ERK signaling pathways in conjunction with HIF-1 provide a mechanism by which nucleus pulposus cells remain viable and maintain their specialized physiological function, despite environmental limitations in O2 and possibly changes in nutrient availability.
Two proteins that have been linked to nucleus pulposus survival in hypoxia are VEGF-A and galectin-3. In disc cells, it has been reported that HIF-1 regulates galectin-3 expression (Zeng et al. 2007). From a functional perspective, by forming complexes with integrins, externalized galectin-3 influences cell adhesion and spreading (Sasaki et al. 1998). Accordingly, galectin-3 is most likely involved with matrix stability and in concert with HIF-1 provides the discal cells with both a mechano-transduction and a survival function. Other studies have shown that galectin-3 regulates survival by suppressing signaling through the TNF family of proteins (Oka et al. 2005). This finding is particularly pertinent to disc disease, as TNF-α together with other cytokines is known to play a major role in the etiology, as well as progression, of the degenerative state. Based on these findings, it is possible that in the hypoxic intervertebral disc, the robust expression of HIF-1α serves to maintain galectin-3 levels, which then serve to promote cell survival and disc function (Zeng et al. 2007).
With respect to VEGF-A, not surprisingly, levels of this protein are high in herniated discs or in degenerative discs where there is evidence of neovascularization (Kokubo et al. 2008). In the normative state, because the disc environment is avascular, it would be reasonable to assume that VEGF expression is low. However, this is not the case as there is robust expression of this protein and its receptor in the nucleus pulposus (Fujita et al. 2008; Agrawal et al. 2008). Most likely, the level of expression is related to both HIF-1 and HIF-2, as both isoforms upregulate VEGF-A expression and promoter activity (Agrawal et al. 2008). This observation begs the question: in the disc, what is the function of VEGF? Clearly, it cannot serve to promote angiogenesis as this activity would promote vascularization and compromise disc function. There is some information to indicate that VEGF supports cell survival (Zelzer et al. 2004). Indeed, Fujita et al. (2008) confirmed that VEGF and its receptors are expressed by nucleus cells in hypoxia and showed that this protein promoted nucleus pulposus survival. Thus, from a functional viewpoint, VEGF could serve as to maintain nucleus pulposus viability in the face of shifts in environmental pO2.
As indicated above, there is growing interest in the second HIF homologue, HIF-2α. This protein is robustly expressed by nucleus pulposus cells. With respect to functional activities, unlike most other tissues, hypoxia failed to increase the transcriptional activities of SOD2 and frataxin, two common HIF-2 target genes concerned with radical dismutation (Scortegagna et al. 2003; Oktay et al. 2007). This finding could explain why this tissue is susceptible to radical attack associated with annular lesions or nucleus herniation. Notably, there is evidence to indicate that kyphosis, scoliosis, and radiculopathies are linked to defective radical dismutation (Murakami and Kameyama 1963; Sparrow et al. 2012), whereas Friedreich’s ataxia is now known to be attributable to low frataxin levels and loss of antioxidant defenses (Gakh et al. 2006). It would be important to know whether these conditions are also linked to the inability of vertebral tissues to mount a robust HIF-2-dependent scavenging response.
Notably, both HIF-1 and HIF-2 are involved in survival of endplate chondrocytes by activation of the autophagic pathway (Bohensky et al. 2009; Srinivas et al. 2009). Recent studies suggest that autophagy is active in the nucleus pulposus (Ye et al. 2011; Jiang et al. 2012). The importance of this system for removal of misfolded proteins and damaged organelles has been emphasized by a number of workers, and its role in directing the maturation of connective tissue cells has been discussed by Srinivas and his colleagues (Srinivas et al. 2009). Noteworthy, although autophagy is viewed as a survival pathway, there is little doubt that continued macromolecular breakdown, while serving as a source of nutrients and energy for the stressed cell, inevitably leads to increased susceptibility to apoptosis (type II apoptosis). Hence, HIF activity and ultimately HIF targets serve as key proteins that straddle both the apoptotic and survival pathways.
Bohensky et al. (2009) pointed out that HIF-2 was involved in regulating survival by modulating autophagy. HIF-2 was expressed abundantly by cells in human and murine articular cartilage, hypertrophic cartilage, and in the endplate cartilage. When HIF-2α was suppressed, ROS generation was elevated, and there was a decrease in the activity of the ROS dismutating enzymes catalase and superoxide dismutase. Suppression of HIF-2α was associated with decreased Akt-1, reduced Bcl-x(L) expression, and a robust autophagic response, even under nutrient-replete conditions (Bohensky et al. 2009). In addition, Semenza and colleagues have demonstrated important contributions of HIF-1α to autophagy (Zhang et al. 2008). Relevant to disc disease, it is generally agreed that the degenerative state is exacerbated by a decrease in the permeability of the endplate cartilage and the concomitant reduction in nutrient availability. It is possible that under these nutritionally challenging conditions, increased HIF-1/-2 expression may serve to maintain nucleus pulposus survival by promoting the induction of autophagy (Box 6.2).
6.2.4.1 Box 6.2: HIF, Hypoxia, and Human Populations
Oxidative metabolism and the availability of oxygen have powered evolutionary processes that have permitted animals to populate almost every region of the planet. At high altitudes and in the depth of the ocean, the partial pressure of oxygen is low; nevertheless, organisms have evolved mechanisms to adapt to these nonphysiologic conditions. The question that has intrigued scientists is: what type of adaptation mechanism exists to enable humans and animals to reside in harsh environments characterized by the high Andean, Tibetan, and Ethiopian plateaus? For humans living at high altitudes, there may be genetic selection pressures for phenotypes characterized by raised oxygen hemoglobin saturation; one problem here is that a change in blood viscosity can elicit profound medical problems. However, studies of Tibetans living at very high altitudes show that they exhibit an SNP close to the region encoding HIF-2. Beall et al. (2010) have proposed that this mutation causes a “blunted erythropoietic response.” In this case, the change in viscosity would be minimal. There is evidence that HIF-1 isoform may also be involved. Studying the Naqu Yak, an animal that has lived on the Tibetan plateau for more than million years at an altitude of 9,000–15,000 ft above sea level, Wang et al. (2006) reported that HIF-1 is expressed at high levels in the brain, lung, and kidney. Since HIF-1 targets EPO (erythropoietin) as well as many other genes concerned with erythropoiesis, these animals would have an enhanced ability to transport oxygen in the blood stream. In summary, both animal and humans have adapted to hypoxia by expressing genes that modify oxygen transport and erythropoiesis.
References
Beall CM, Cavalleri GL, Deng L, Elston RC, Gao Y, Knight J, Li C, Li JC, Liang Y, McCormack M, Montgomery HE, Pan H, Robbins PA, Shianna KV, Tam SC, Tsering N, Veeramah KR, Wang W, Wangdui P, Weale ME, Xu Y, Xu Z, Yang L, Zaman MJ, Zeng C, Zhang L, Zhang X, Zhaxi P, Zheng YT (2010) Natural selection on EPAS1 (HIF2alpha) associated with low hemoglobin concentration in Tibetan highlanders. Proc Natl Acad Sci U S A 107(25):11459–11464
Wang DP, Li HG, Li YJ, Guo SC, Yang J, Qi DL, Jin C, Zhao XQ (2006) Hypoxia-inducible factor 1alpha cDNA cloning and its mRNA and protein tissue specific expression in domestic yak (Bos grunniens) from Qinghai-Tibetan plateau. Biochem Biophys Res Commun 15:348(1):310–349
6.3 Contribution of TonEBP to the Maintenance of the Osmotic Niche
For disc cells, the second major environmental challenge is the high osmotic pressure of the niche (this topic is also discussed in Chap. 2). As pointed out earlier, the biomechanical function of the nucleus pulposus is primarily attributed to the water binding and unique osmotic properties of the tissue. Osmotic pressurization of the nucleus pulposus balances the loads experienced by the spine. Studies by Urban and colleagues indicate that the tissue osmolarity is substantially higher than that of plasma and is in the range of 400–500 mosmol/kg (Urban and Maroudas 1981; Ishihara et al. 1997).
Until recently, the mechanism by which cells of the nucleus pulposus and annulus fibrosus control their intracellular osmotic properties was not known. Drawing on information relevant to other tissues, it was noted that cellular adaptation to hyperosmotic stress is mediated by the tonicity enhancer-binding protein (TonEBP), also called OREBP (Miyakawa et al. 1999) or NFAT5 (Lopez-Rodríguez et al. 1999). TonEBP/NFAT5 belongs to the 5-member NFAT protein subfamily (NFAT1-5) which is part of the larger Rel superfamily of proteins. Similar to other members of the Rel family, it contains the Rel homology domain, a conserved DNA-binding domain. However, no similarities are seen between TonEBP and NF-κB or NFAT1-4 outside of the Rel homology domain. It lacks the binding site for calcineurin necessary for NFAT1-4 dephosphorylation and subsequent nuclear translocation (Lopez-Rodríguez et al. 1999). It is the largest Rel family member and unlike monomeric members of the NFAT family exists as a homodimer and forms stable dimers with DNA. Upon activation, TonEBP binds to the tonicity-responsive enhancer element (TonE) of genes required for osmotolerance and cell survival. These genes include the betaine/γ-aminobutyric acid transporter, sodium myo-inositol co-transporter (Ko et al. 1997; Miyakawa et al. 1998; Rim et al. 1998), taurine transporter (Zhang et al. 2003; Ito et al. 2004), and aldose reductase (Lopez-Rodríguez et al. 1999). By regulating levels of betaine, myo-inositol, taurine, and sorbitol, these genes control the osmotic properties of the cytosol. Hsp70, a molecular chaperone that maintains cellular function under hypertonic stress is also induced by TonEBP (Woo et al. 2002; Shim et al. 2002). Most homozygous TonEBP knockout mice evidence midgestational lethality. Of the few that survive, all exhibit severe growth retardation and kidney dysfunction (Lopez-Rodriguez et al. 2004). A transgenic mouse expressing a dominant-negative form of TonEBP (DN-TonEBP) in collecting duct epithelial cells demonstrated an absolute requirement of TonEBP for expression of the urea transporter gene and aquaporin-2 (Lam et al. 2004).
Aside from osmoregulation, TonEBP is required for T cell proliferation and function (Trama et al. 2000; Go et al. 2004), and it is implicated in cancer cell migration and metastasis (Jauliac et al. 2002). A study by Wang et al. (2005) showed that expression of DN-TonEBP in lens fiber cells promoted cataract formation by causing defects in their elongation. Since TonEBP is expressed by a number of cell types, it is reasonable to assume that it serves a variety of physiologic functions, especially those that impact on tissue hydration and the osmotic environment (Maouyo et al. 2002).
TonEBP and its downstream target genes are robustly expressed in the nucleus pulposus and the annulus fibrosus (see Fig. 6.4). Importantly, TonEBP is critical for maintenance of nucleus pulposus survival under hyperosmotic conditions (Tsai et al. 2006). The observed increase in apoptosis is in agreement with studies of TonEBP null mice and transgenic animals expressing DN-TonEBP in the thymus and in the lens (Trama et al. 2000; Go et al. 2004; Wang et al. 2005). In both these conditions, there was an acceleration of cell death through apoptosis. Very importantly, recent studies in C. elegans demonstrating selective and pronounced expression of TonEBP in the notochord suggest that its osmoregulatory function first evolved in the primitive axial skeleton prior to diversification to other tissues (José-Edwards et al. 2011). The relationship between TonEBP and cell death and its expression in the notochord lends strength to the notion that this transcription factor is of critical importance in the life history of cells of the nucleus pulposus.