Fig. 9.1
The “slider crank” concept of wrist flexion and extension [Reprinted from Linscheid RL, Dobyns JH, Beabout JW, Bryan RS: Traumatic instability of the wrist J Bone Joint Surg. 1972; 54(8): 1612–1632 with permission of the Journal of Bone and Joint Surgery]
Although most investigators had long believed that the center of rotation (COR) of the wrist was confined solely within the head of the capitate, Wright reported in 1935 that the COR of the wrist joint resided in the head of the capitate during wrist flexion and shifted to the intercarpal joint during extension [14]. The distal carpal row DCR rotates about a fixed axis within the head of the capitate throughout radioulnar deviation [15, 16]. The distance between the base of the third metacarpal and the distal radial articular surface (carpal height index (CHT)—normal C/MC = 0.56) on a neutrally positioned anteroposterior image is constant throughout radio ulnar motion.
CHI can be used to measure carpal collapse [15, 16]. The perpendicular distance from the distally projected longitudinal axis of the ulna to the axis of rotation for radioulnar deviation on a neutrally positioned anteroposterior image is used to measure carpal translation. Three-dimensional analysis of the instantaneous screw axes (ISA) calibrated for the position of the third metacarpal base with respect to the distal radius revealed that the COR of wrist motion is not fixed or limited to the capitate during global motion [3]. ISA data also demonstrated that translational motion in the normal wrist may account for the difference between this study and previous reports.
In 1921, Navarro conceptualized a columnar wrist model to better explain the sophisticated and multidirectional movements of the wrist [17]. He theorized that three interdependent columns best correlated carpal anatomy with function. The lateral column (scaphoid, trapezium, and trapezoid) supported the thumb and transferred load between the other two carpal columns. The central column (lunate, capitate, and hamate) flexed and extended the wrist. Rotation was controlled by the medial column (triquetrum and pisiform). In 1978, Taleisnik modified the column theory to exclude the pisiform, recognizing that it played no integral role in intercarpal motion [18]. He also determined that the bones of the normal DCR are securely fixed together by stout ligaments, have very little intercarpal motion, and act as a unit. He therefore included the trapezium and trapezoid, along with the capitate, hamate, and lunate, as parts of the central column. The DCR is also rigidly secured to the bases of the second and third metacarpals and, consequently, moves with the hand (Fig. 9.2). Weber took a slightly different view of the columnar theory [19]. He divided the carpus into two columns; the load-bearing radial column composed of the scaphoid, lunate, trapezium, trapezoid, and capitate and the ulnar control column, consisting of the triquetrum and hamate. He viewed the helicoid triquetrohamate joint as the key to wrist position during rotation and load changes.
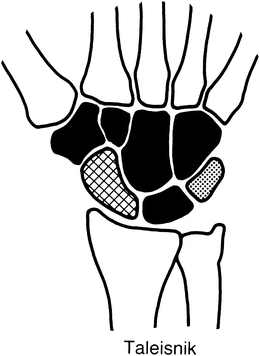
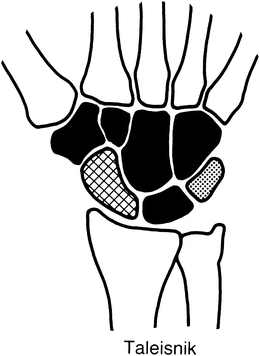
Fig. 9.2
Taleisnik’s columnar concept
Lichtman et al. formulated the next step toward a better understanding of three-dimensional wrist motion with their “oval-ring” theory [20] (Fig. 9.3). Their theory perceived the wrist as four interdependent segments: the DCR, scaphoid, lunate, and triquetrum. Ligamentous links connect each segment to its two adjacent elements. The scaphotrapezial (radial link) and triquetrohamate (ulnar link) joints form two reciprocal physiologic links. Radial deviation creates an unbalanced flexion moment at the radial link inducing proximal row flexion and palmar capitate and hamate subluxation (physiologic VISI). An unbalanced extension moment at the ulnar link causes the triquetrum to extend against the hamate with the PCR following and the capitate and hamate transitioning dorsally (physiologic DISI). Continuity of the ligaments assures synchronous synergistic carpal motion within the moving wrist. Disruption of any link(s) results in dysfunction. Craigen and Stanley pointed out that certain elements of both the column and row theories, although sometimes contradictory, are useful in our understanding of multidimensional wrist motion [21].
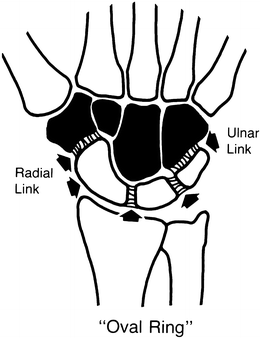
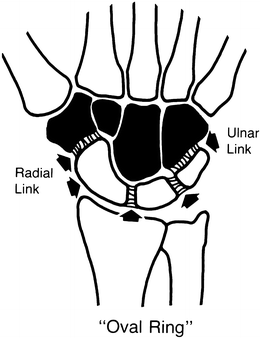
Fig. 9.3
Lichtman’s oval ring [Redrawn from Lichtman DM, Schneider R, Swafford AR, Mack GR. Ulnar midcarpal instability: Clinical and laboratory analysis. J Hand Surg 1981; 6(5): p. 515–523. With permission from Elsevier]
During wrist flexion-extension, the motion of the capitate closely follows that of the third metacarpal, while the lunate motion is approximately 50 % of the total motion, the triquetrum 65 %, and the scaphoid 90 % [2, 22]. Similar differences in motion for these carpal bones occur during radioulnar deviation, circumduction, and the DTM. This suggests that the scaphoid, lunate, and triquetrum do not normally function as a single unit, but that each bone has a unique arc of motion during global wrist motion. This three-dimensional study of carpal rotational behavior supported a row concept of wrist motion as opposed to a carpal column model [2, 22].
Perhaps, at this point in time, we can conclude that the forearm, wrist, hand, and articular contact position at the moment of impact; point of impact; columns; rows; individual carpal bones; amount, direction, and rate of the applied and resistance forces; individual bone and articular cartilage morphology and containment; and viscoelastic ligament properties and relative strengths, especially in the PCR, interact to provide various multiplanar global wrist motions and play a role in injury susceptibility. Each of these parameters allows some measure of integral static and continuous dynamic quantification for biometric analysis, comparisons, communication, and management of these injuries as we unravel the comprehensive three-dimensional geometrics of normal and abnormal carpal motion with and without loading.
Normal Carpal Kinematics
The PCR is intercalated between the radius and ulna proximally and the DCR distally [23]. The scaphoid pivots over the radioscaphocapitate ligamentous fulcrum at its waist. The distal pole of scaphoid flexes as the trapezium and radial styloid close over it during wrist flexion and/or radial deviation. The scaphoid extends as the distance between the trapezium and radial styloid widens during wrist extension and/or ulnar deviation. The scaphoid and lunate flex and pronate during wrist flexion and/or radial deviation, while extending and supinating during wrist extension and/or ulnar deviation. Scaphoid flexion, extension, and rotation exceed that of the lunate [2, 22]. During full wrist flexion in the sagittal anatomic plane, the scaphoid flexes 35° more than the lunate and pronates three times as much. Lunate radioulnar and dorsopalmar translation in its radial fossa is minimal, but exceeds that of the scaphoid. The distal pole of the scaphoid has relatively more motion than has the proximal dorsal pole. The scaphoid moves somewhat as a rotating triplanar pendulum [2, 22]. The palmar SLIL fibers lengthen and the dorsal fibers shorten with wrist flexion [24]. The opposite occurs with wrist extension.
Radial and ulnar deviation primarily occurs in the midcarpal joint. Midcarpal motion accounts for 60 % of radial deviation and 86 % of ulnar deviation [25]. The radiocarpal and scapholunate joints remain relatively stable. In radial deviation the PCR flexes and the capitate moves slightly radiodorsally relative to the lunate. The scaphoid flexes and radially deviates. In ulnar deviation, the PCR extends and the capitate moves slightly ulnopalmarly on the lunate. Midcarpal motion relative to the capitate rotates in a plane from dorsoradial to ulnopalmar, similar to the DTM [2–9, 25, 26].
The PCR translocates radiopalmarly and rotates dorsally during ulnar deviation as the triquetrum pronates and shifts palmarly, distally, and ulnarly on the hamate’s helicoid articular slope [16, 19]. The midcarpal joint slightly flexes. Conversely, the PCR translocates dorsoulnarly and rotates palmarly during radial deviation as the triquetrum supinates and shifts dorsally, proximally, and radially. The lunate geometry accommodates the rotational shifts within the PCR, maintaining a constant carpal height throughout radioulnar wrist motion in the frontal plane [15, 16]. The scaphotrapeziotrapezoidal ligament complex (STTL) and the lateral portion of the RSCL support the distal scaphoid throughout extension and ulnar deviation.
The SLIL has three anatomic regions [27, 28]. The dorsal SLIL (dSLIL) is thick and composed of short, transversely oriented collagen fibers. The palmar SLIL (pSLIL) is thin and contains obliquely oriented collagen fascicles. The proximal mid-region of the SLIL (mSLIL) is composed of fibrocartilage, with a few superficial, longitudinally oriented collagen fibers and extends distally into the scapholunate joint (SLJ) space, resembling a meniscus. The mesenteric-like radioscapholunate ligament (RSLL) separates the mSLIL and pSLIL, and spreads distally over the proximal scaphoid, SLIL, and lunate. The RSLL encloses small caliber neurovascular elements to and from the scaphoid, lunate, and SLIL. The yield strength of the dSLIL component is 260 ± 118 N, the mSLIL 63 ± 32 N, and the pSLIL 118 ± 21 N. The RSLL provides little, if any, structural support and depends upon its elasticity and neighboring structures for protection.
There is no dorsal radioscaphoid ligament (DRSL) [29–31]. Such a ligament would require an elastic coefficient three times its resting length. This prerequisite exceeds the inherent physical capacity of ligaments. The dorsal radiolunotriquetral (DRLTL) and dorsal intercarpal (DICL) ligaments form a V-shaped configuration on the dorsum of the wrist with its converging apex on the ulnar side. The DRLTL originates from the dorsal lip of the distal radius, passes over the proximal pole of the scaphoid, extends obliquely, attaching to the distal dorsal ulnar lunate, dorsal ulnar lunate ligament (DULL), and dorsal LTIL, and inserts onto the dorsal tubercle of the triquetrum. The DRLTL lends support to the midcarpal joint and passively pronates the attached carpus during forearm pronation. The DICL has a thick proximal and a thinner distal transverse band. The DICL originates from the dorsal triquetrum and hamate, extends radially, attaches to the dorsal lip of the lunate, and inserts its thickest attachment into the dorsal groove of the scaphoid where its anterior fibers blend imperceptibly with the strong dorsal fibers of the dSLIL before fanning onto the dorsal trapezium and proximal trapezoid.
The DVL substitutes for some of the function that a DRSL might provide throughout normal carpal kinematics by maintaining an indirect stabilizing effect on the proximal pole of the scaphoid by threefold narrowing and widening of the distance between the DRLTL origin and the scaphoid insertion of the DICL during maximum wrist extension and flexion, respectively. The STTL, RSCL, DRLTL, and the DICL individually and conjointly are secondary stabilizers of the scapholunate joint (SLJ) [29–32].
The normal relationship of the scaphoid, lunate, and their related ligaments is illustrated in Fig. 9.4. The normal SLIL has an inverted V appearance with the apex of the V distally (“Tushy sign”), as visualized from the radiocarpal (3-4) arthroscopic portal. From the radial midcarpal portal, the normal SLJ is congruently aligned and immobile (Fig. 9.5). The scaphoid is flexed approximately 47° relative to the third metacarpal-capitate-lunate-radial longitudinal axis in the lateral X-ray view. The SLJ space is stable, congruent, and does not exceed 2 mm on the AP X-ray.
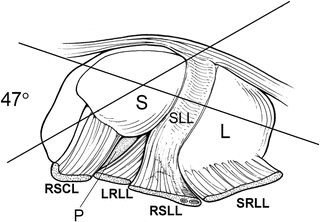
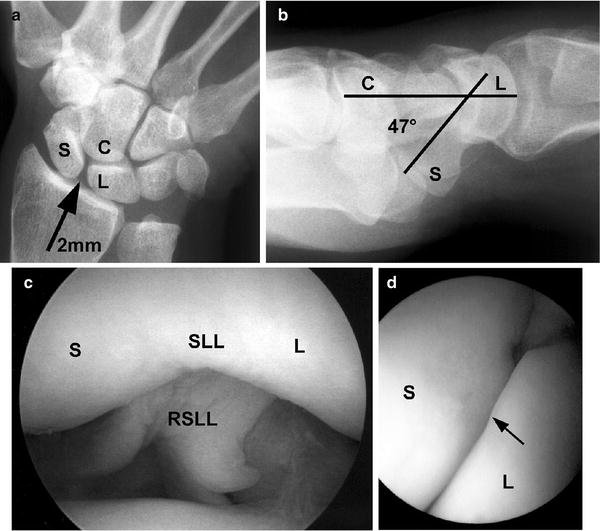
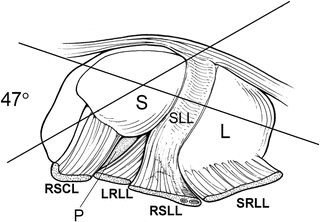
Fig. 9.4
Normal scapholunate alignment. S scaphoid, SLL scapholunate ligament, L lunate, RSCL radioscaphocapitate ligament, P interligamentous sulcus leading to the space of Poirier, LRLL long radiolunate ligament, RSLL radioscapholunate ligament, SRLL short radiolunate ligament
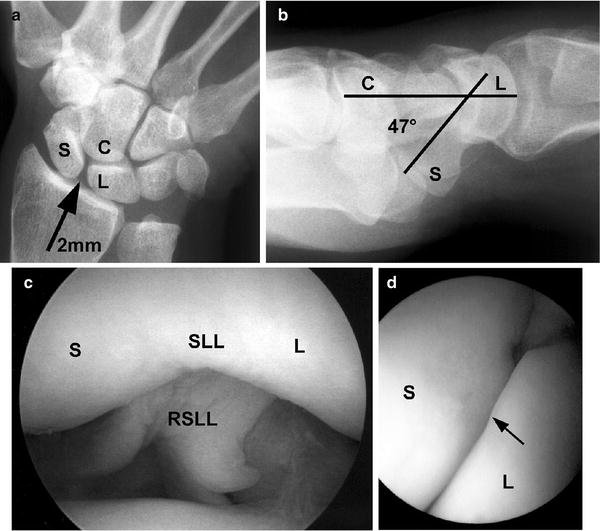
Fig. 9.5
Normal scapholunate alignment. (a) Normal AP X-ray (arrow points to the scapholunate joint). (b) Normal lateral X-ray and scapholunate angle. (c) Radiocarpal (3-4 portal) arthroscopic image demonstrating a normal SLL and RSLL. (d) Midcarpal arthroscopic view with normal scapholunate alignment
The triquetrum is the fulcrum for wrist rotation and motion in the radioulnar plane. The helicoid geometry of the triquetrohamate joint is instrumental in accommodating this screw-like movement [20]. The tensile strengths of dLTIL and pLTIL are the obverse or reciprocal of the dSLIL and pSLIL. The dLTIL yield strength 121 ± 42 N, the mLTIL 64 ± 14 N, and the pLTIL (pLTIL) 301 ± 36 N [33]. Although the pLTIL is stronger than the dLTIL, it is less flexible.
Classification of Carpal Instabilities
Although there is probably no single uncontested, comprehensive, or perfect classification for carpal instabilities, several parameters allow some measure of quantification that is useful for analysis, comparison, communication, and management of these injuries [34]. These parameters include chronicity, constancy, etiology, location, direction, and pattern.
Chronicity
Classification by chronicity relates to the time interval between injury and diagnosis. This categorization is based upon the capacity for carpal reduction and, especially, upon the intrinsic capability for ligament healing after treatment. Acute carpal instabilities are those diagnosed and treated within 1 week of injury. These instabilities are reducible and have the highest potential for ligament healing. Subacute tears are those diagnosed and treated between 1 and 6 weeks after injury. Although they are reducible, the capacity for primary ligament healing is diminished. Injuries seen after longer than 6 weeks from the time of injury are considered chronic, have little capacity for ligament healing, and may occasionally be irreducible.
Constancy
Carpal instability and symptoms may be apparent immediately in some cases; in others, they may take an initially indeterminate amount of time to appear as the carpi settle or the tear(s) extend. Ligaments that tear beyond the axis connecting the centers of rotation of two bones often have carpal malalignment that may be seen on standard wrist X-rays with the wrist at rest. This type of carpal collapse is termed static carpal instability. This category may be further divided into reducible and irreducible injuries.
Patients with lesser ligament injuries often have normal standard X-rays, yet carpal malalignment and symptoms occur during motion and loading. This type of carpal collapse is termed dynamic carpal instability. The diagnosis of dynamic instability may require stress X-rays, such as an anteroposterior (AP) distraction X-ray with digital traction, manual stress X-rays, a six-view AP (radial deviation, neutral, and ulnar deviation), and lateral (extension, neutral, and flexion) X-ray with a tightly gripped fist; fluoroscopic cineradiography; enhanced gap-free MRI; and/or arthroscopic evaluation. Attenuation and partial ligament tears may propagate over a period of time and use. Carpal collapse, correlative symptoms, and classification may advance accordingly.
Etiology
Trauma and synovitis are the principal causes of carpal ligament disruption. The former is more common. The healing capacity of traumatic ligament injuries is limited and unreliable, owing to a disrupted and/or meager blood supply and technical difficulties in achieving successful repair by suturing. Synovitis erodes ligaments and renders them irreparable.
Location
Location refers to the specific ligament(s) injured, fracture(s), and carpal bones and joints involved.
Direction
Direction is primarily determined by evaluating a true lateral X-ray in the sagittal plane with the resting wrist in a neutral position. The axial and tangential methods of radiographic measurement are equally accurate in assessing carpal angles [35–37] (Fig. 9.6). The scaphoid tubercle and pisiform must be maximally superimposed to assure a true lateral (sagittal plane) wrist X-ray or image and to eliminate or minimize observer variability [38, 39]. The normal scapholunate angle on lateral X-ray views increases from an average of 35° of flexion in full wrist extension to 76° in full flexion. With the normal wrist in a neutral position, the scaphoid is flexed at about 47° (the angle of Alexander).
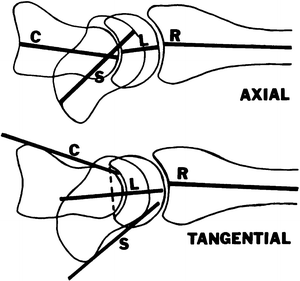
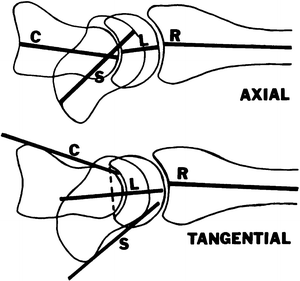
Fig. 9.6
Axial (above) and tangential (below) methods of measuring carpal angles. C capitate, S scaphoid, L lunate, R radius [Reprinted from, Garcia-Elias M, An KN, Amadio PC, et al.: Reliability of carpal angel determinations. J Hand Surg 1989; 14(6): 1017–1021. With permission from Elsevier]
The doubly intercalated lunate is a prime radiographic sentinel for both normal and pathophysiologic carpal kinematics [23]. First, the lunate is intercalated BETWEEN the scaphoid and the triquetrum. Lunate flexion and extension occur with the PCR and wrist during normal motion. The lunate is collinear with the capitate in a true lateral (sagittal plane) X-ray or imaging study in the normal resting wrist. The lunate is normally balanced between the scaphoid, which independently tends to flex, and the triquetrum, which intrinsically tends to extend.
In scapholunate dissociation the lunate dorsiflexes and the lunocapitate and scapholunate angles increase [23]. In lunotriquetral dissociation, the lunate palmar flexes and the lunocapitate angle increases in the direction opposite that of scapholunate dissociation. The lunotriquetral angle increases.
Second, the lunate is intercalated WITHIN the proximal row [23]. In midcarpal instability (MCI), when the lunate dorsiflexes and subluxes under the head of the capitate, the injury is termed a dorsal intercalary segment instability (DISI). When the lunate palmar (volar) flexes and subluxes over the head of the capitate, the injury is termed a volar intercalary segment instability (VISI). Knowledge of the lunate as a marker helps to sort out midcarpal, adaptive, and combined or complex carpal instabilities.
Pattern
Four intrinsic carpal instability patterns are recognized. When the ligaments restraining the scaphoid and triquetrum to the lunate are intact, the PCR flexes and extends as a unit. Scapholunate or lunotriquetral ligament injuries cause dissociative and reciprocally opposite rotation of the scaphoid and the triquetrum, respectively, within the PCR. These injuries are therefore defined as carpal instability dissociative (CID). Displaced transtriquetral, and especially transscaphoid, fractures may result in similar patterns. In displaced scaphoid fractures the proximal scaphoid fragment extends with the lunotriquetral unit and the distal fragment flexes owing to opposing forces. Ligament injuries between the radius and/or ulna and the PCR (the radiocarpal and/or ulnocarpal joints) or between the PCR and DCR (the midcarpal joint) are labeled carpal instability nondissociative (CIND). Carpal instability adaptive (CIA) refers to MCI (CIND) from a skeletal injury adjacent to, but not directly involving, the carpal bones and their connecting ligaments. Extra-articular distal radial fractures and malunions with loss of dorsal inclination and dorsal second and third carpometacarpal dislocations or fracture dislocations may cause CIA [40, 41]. If CIA is corrected with reduction of the causative skeletal deformity, the carpal bones usually realign in a normal or nearly normal posture. Coexisting CID and CIND are classified as carpal instability combined or complex (CIC).
Pathophysiology
Patients with ulnar negative variance are at increased risk of SLIL injury [42]. Patients with ulnar negative variance and a lunate fossa with an increased radioulnar slope on anteroposterior images are at increased risk of MCI [43]. Larger and deeper scaphoid fossae and greater palmar tilt of the distal radius and greater proximal articular curvatures may shield the wrist from incurring SLIL injury or developing instability after SLIL injury [44]. Wrists with a TYPE II lunate tend to be less susceptible to SLIL injury and progressive perilunar instability (PPI) than patients with a TYPE I lunate; however, they have an increased risk of developing lunohamate arthrosis [45, 46].
Ligament injury is one of degree and may be divided into attenuation or stretch injuries (in which the elastic coefficient of the ligament is exceeded without tearing), partial tears, and complete tears [47]. Attenuation may occasionally be associated with predynamic instability that is unapparent even with joint loading or stress. Attenuation and partial ligament tears are frequently associated with dynamic instability that is apparent only with joint loading or stress. Complete ligament tears, and in some instances specific isolated ligament sectioning, are associated with static instability, owing to some degree of adjacent external secondary ligament injury or division. Bone avulsion may occur in concert with ligament tears and sometimes helps to locate and identify these injuries.
Vertical Carpal Instabilities
Scapholunate Instability (SLI) and Progressive Perilunar Injury (PPI)
PPI results from sequential ligamentous injuries due to wrist extension, ulnar deviation, and supination in relation to a stable forearm during a fall or force on the outstretched hand (FOOSH) [48]. The sequence continues until the energy from the force is completely expended. These forces may also disrupt the wrist ligaments when they occur during other wrist injuries, particularly radial styloid fractures and intra-articular, or even extra-articular, distal radial fractures [48, 49]. Injuries to the SLIL and/or LTIL ligaments are classified as lesser arc injuries [50]. Transscaphoid, transcapitate, and transtriquetral fractures are greater arc lesions (Fig. 9.7). Fractures, in these instances, are more easily treated and heal more reliably than ligament injuries. Concurrent proximal pole fractures of the scaphoid and SLIL tears have been reported and are challenging both to diagnose and treat [51–53].
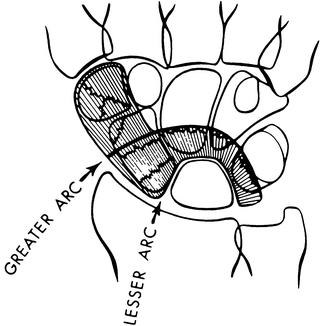
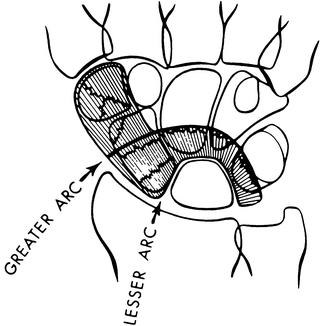
Fig. 9.7
The lesser and greater carpal arcs [Reproduced with permission from Blazar PE, Lawton JN. Diagnosis of carpal ligament injuries. In: Trumble TE (ed.): Carpal Fracture-Dislocations. Rosemont, IL: American Academy of Orthopaedic Surgery; 2002]
Mayfield et al. have reported four progressive, representative, integral STAGES within the SPECTRUM of PPI [48] (Fig. 9.8). STAGE I injuries are characterized by disruption of the SLIL and adjacent ligaments. The SLIL is the primary stabilizer of the scapholunate joint [54–56]. The RSCL and STTL are secondary stabilizers. The SLIL usually tears from its attachment to the scaphoid. PPI STAGE I Injuries attenuate or tear the space of Poirier palmarly and distally and progressively extend proximally to disrupt the pSLIL until there is scapholunate diastasis with disruption of the RSCL or radial styloid fracture and tearing of the dSLIL and scaphoid insertion of the DICL. Complete scapholunate instability (SLI) occurs when the DICL attachment to lunate is disrupted. Attenuation and partial SLIL tears anterior to the scapholunate axis between the centers of rotation of the scaphoid and the lunate usually result in dynamic scapholunate instability. Partial SLIL tears dorsal to the scapholunate axis between the centers of rotation of the scaphoid and the lunate and complete SLIL tears result in static scapholunate instability.
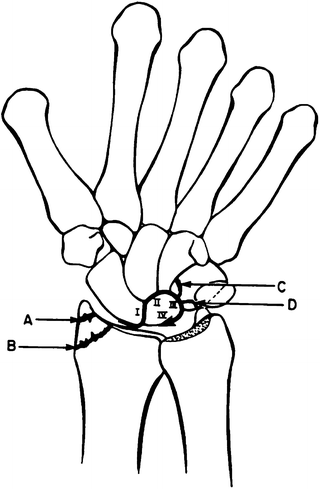
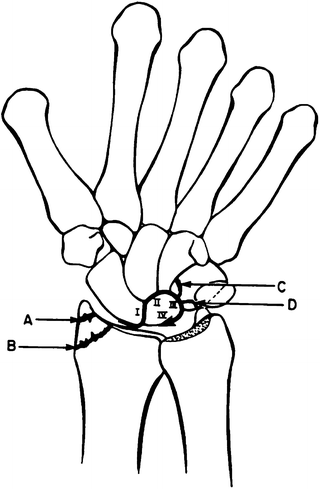
Fig. 9.8
Mayfield’s STAGES of progressive perilunate dislocation [Reprinted from: Mayfield JK, Johnson RP, Kilcoyne RK: Carpal dislocations: pathomechanics and progressive perilunate instability. J Hand Surg 1980;5(3):226–241.with permission from Elsevier]
Laboratory studies have demonstrated that in vitro complete sectioning of the SLIL alone does not cause scapholunate widening or carpal instability in the resting wrist [54–56]. In vitro sectioning of the SLIL caused mild scapholunate instability during wrist flexion and extension, but only minimal scapholunate instability throughout radioulnar deviation. Loading causes slight abnormal motion between the scaphoid and the lunate that may account for predynamic or mild dynamic instability. Although laboratory sectioning of the entire SLIL causes minimal SLI, traumatic clinical SLIL disruption can cause marked static scapholunate diastasis and instability, owing to involvement of adjacent extra-articular ligaments.
The computation of the total hysteresis area from the hysteresis effect is a sensitive technique that can determine the subtle onset of abnormal carpal motion [57]. Whereas the sectioning of the SLIL, RSCL, and STTL is required to produce changes in the hysteresis area in flexion-extension curves, in vitro sectioning of the SLIL alone increases the total hysteresis area during wrist radioulnar deviation. This subtle finding may identify the onset of dynamic SLI. The total hysteresis area of the lunate may increase as well; however, a paradoxical decrease of the total hysteresis effect of the lunate with hypermobile (lax) wrists may explain why some patients with SSI do not develop DISI deformities.
Further in vitro sectioning of the DICL causes scapholunate widening without static carpal collapse [31, 58]. Additional sectioning of the DICL attachments to the lunate causes greater scapholunate widening. The scaphoid flexes and dissociates from the extending lunotriquetral unit causing static carpal collapse. The proximal pole of the scaphoid subluxes dorsoradially in the scaphoid fossa of the distal radius [59]. Conversely, the lunate subluxes palmarly and ulnarly within the lunate fossa of the distal radius. Abnormal widening of the scapholunate and lunocapitate angles results.
In the intact wrist, scapholunate motion is greater in circumduction than in the DTM, where motion is, in fact, minimal [2–9, 54–56]. Following in vitro sectioning of the RSCL, STTL, and SLIL, the scaphoid flexes more and the lunate extends more during both circumduction and the DTM. Both before and after sectioning, scaphoid motion is greater than that of the lunate. Overall, after in vitro sectioning of the RSCL, STTL, and SLIL, scaphoid motion substantially increased, while lunate motion decreased. This helps to explain the observation that after SLIL tears with associated secondary restrain involvement and continuous motion, arthritic changes occur in the radioscaphoid fossa, but not in the radiolunate joint [60, 61].
Geissler combined radiocarpal and midcarpal diagnostic arthroscopic evaluation to classify the progressive SPECTRUM of SLIL injuries that occur in Mayfield PPI I injuries into four integral arthroscopic GRADES [62] (Video 9.1).
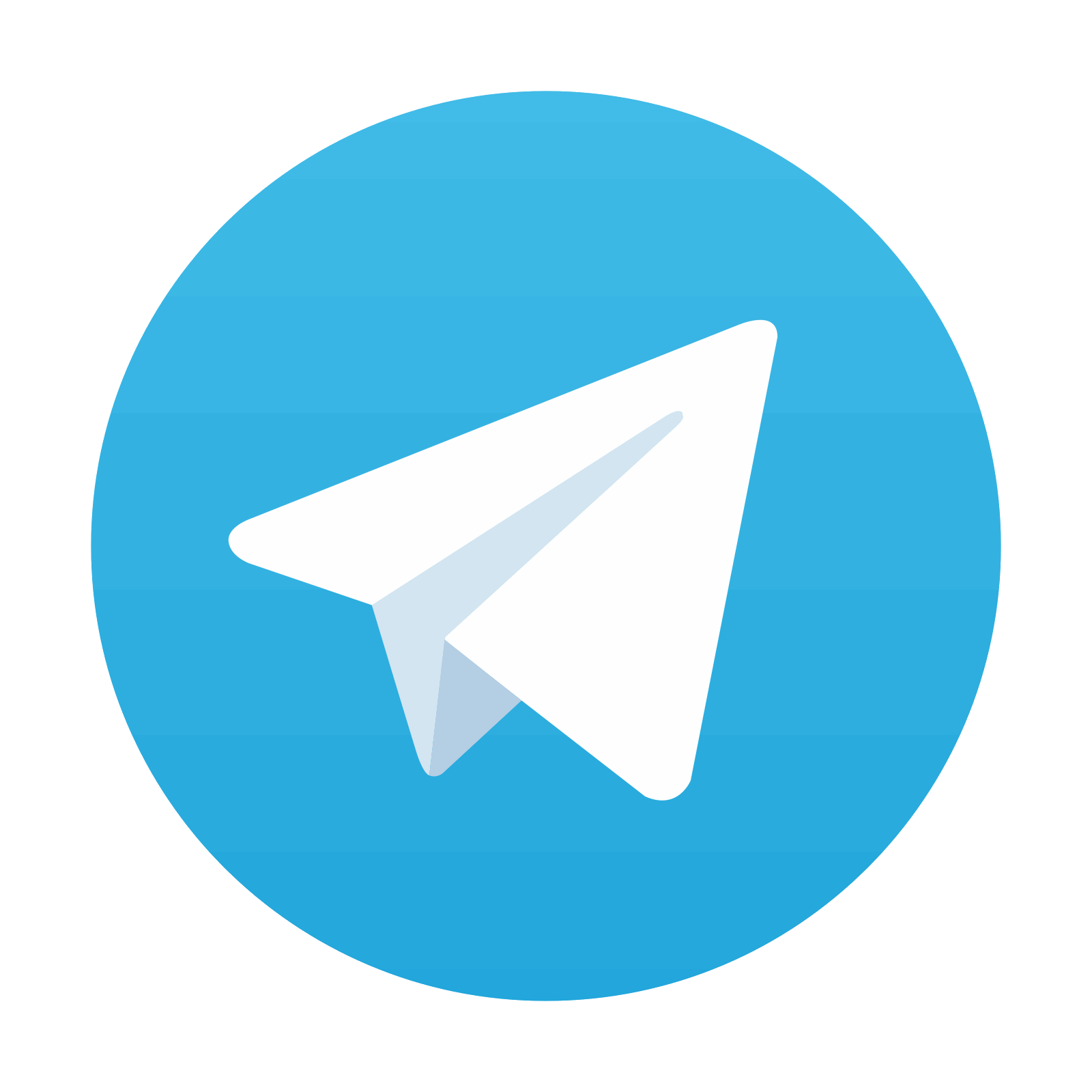
Stay updated, free articles. Join our Telegram channel

Full access? Get Clinical Tree
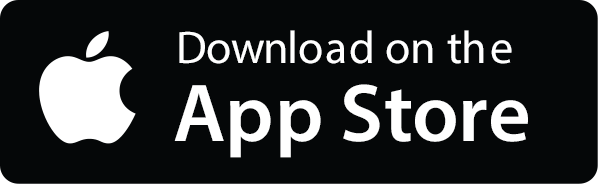
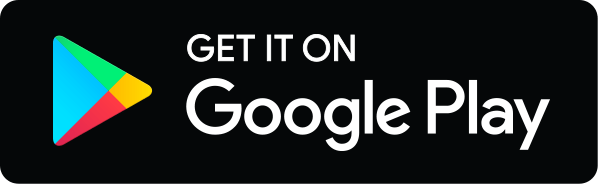