Chapter 15 Steven R. Goodman; Cory B. Choma Gunn intramuscular stimulation (Gunn-IMS) is a technique for the treatment of patients with myofascial pain syndrome (MPS) based on a comprehensive diagnostic and therapeutic model that identifies the aetiology of myofascial pain as neuropathic (i.e., due to disease or dysfunction in the nervous system). Further, it identifies the nerve root as the locus of the pathology, and thus it is a radiculoneuropathic model. It was developed in the 1970s by Dr. C.C. Gunn when treating injured workers and evolved from his clinical observations distinguishing those workers who succeeded in returning to work from those who failed to do so (Gunn & Milbrandt, 1976a). Gunn’s model is a derived clinical model. What grew out of the desire to understand his patients’ persistent pain and offer them treatment ended in a completely new way to see and treat this most universal of all human afflictions: pain. He was the first physician to recognise the subtle physical examination signs of neuropathy and to describe the pathophysiology of neuropathic pain (Gunn & Milbrandt, 1978; Gunn et al., 1980). Gunn’s work reflects not only the great tradition of empirical science, but is also built upon the work of great scientists before him. In an example par excellence of the often quoted homage of scientific progress to the work of all who precede one in the history of science and medicine, ‘dwarfs standing on the shoulders of giants’, Gunn realised the pathophysiological explanation for what he observed clinically in the work of Walter Cannon, the distinguished early 20th century physiologist. Cannon’s research on ‘The Supersensitivity of Denervated Structures, a Law of Denervation’ is an important law of neuropathophysiology that has been previously underrecognised by the medical community (Cannon, 1939; Cannon & Rosenblueth, 1949). Although an entire field of basic and animal neuromuscular physiology research has grown out of this work known as ‘Cannon’s Law’ (CL), it had remained a purely academic and nonclinical pursuit limited to the laboratory until Gunn: Gunn built a bridge from the research laboratory to the medical clinic and rescued CL from experimental obscurity to practical posterity. The prevailing medical–surgical management of persistent spinal and regional musculoskeletal pain is based on what could be called the ‘spondylosis-nociception-inflammation’ model. This model attributes pain to nociceptive and inflammatory aetiologies due to altered structure in a normal peripheral nervous system. Typical diagnoses in this model include ‘disc rupture, degeneration, and inflammation’, ‘nerve root impingement’, ‘facet joint arthropathy’, ‘rotator cuff tear’, ‘extensor elbow/Achilles tendonitis’, ‘hip bursitis’, ‘patella-femoral dysfunction’, and ‘plantar fasciitis’, to name a few. Diagnosis and treatment decisions in this model are based largely on the structural findings of imaging studies (plain x-ray, CT/MRI, nuclear medicine) or the presumption of inflammation, and myofascial pain syndrome is not thought of as bearing any relationship to these entities. This model, however, cannot account for many clinically ‘inconvenient’ facts, including the lack of correlation between anatomic findings and pain (Savage et al., 1997; Borenstein et al., 2001) or the absence of examination findings or histological evidence of inflammation (Khan et al., 1999; Alfredson & Lorentzon, 2002). Connecting the dots between radiculopathy, neuropathy, and myofascial pain, one could say that Gunn discovered the ‘missing link’ between three entities previously thought of as separate, even disparate. Gunn’s radiculo-neuropathic-myofascial pain (RNMP) model explains many of the failures and paradoxes of the traditional model and accounts for many of the facts that a nociceptive and inflammatory model alone cannot. These include the common clinical observations of painless nerve impingement, why pain may resolve despite imaging evidence of persistent nerve impingement or electrodiagnostic evidence of ongoing acute denervation, or why pain may persist even after surgical nerve root decompression or in the absence of detectable inflammation. Understanding persistent spinal and regional musculoskeletal syndromes as manifestations of RNMP and not inflammatory in aetiology explains the common failure of antiinflammatory therapy for these conditions. It also explains why strengthening exercise, which normally produces muscle shortening, often fails to relieve pain, and not infrequently worsens it as it aggravates the already present muscle shortening seen in RNMP syndromes. Alternatively, it explains why such therapies as osteopathic manipulation, myofascial release, stretching, transcutaneous electrical nerve stimulation (TENS), diathermy, acupuncture, trigger point injection, and spinal cord stimulation may be effective. It would predict that muscle relaxant as well as antineuropathic medications such as gabapentin might be effective (Audette et al., 2005). Gunn’s identification of myofascial pain as essentially a neuropathic condition leads to additional insights. As a treatment for MPS, Gunn-IMS is hardly different from the other techniques of superficial and deep dry needling described in this textbook, and not only predicts but readily recognises the efficacy of these approaches. Yet although Gunn-IMS does not differ from dry needling (DN) in much of its technique, or the ‘how’, it does differ substantially from other DN techniques in its understanding of the ‘what’, ‘where’, ‘why’, and ‘when’ of MPS. It differs in explaining ‘what’ causes MPS and trigger points and so how to examine the patient and thus ‘what’ for and ‘where’ to look on physical examination. This leads to a rationale for ‘where’ to treat the patient (i.e., in a segmental or radiculoneuropathic pattern of myotomal involvement). In its recognition of MPS as a fundamentally neuropathic condition, it proposes an explanation of ‘why’ Gunn-IMS and many other forms of counterirritation reflex stimulation are effective in reversing neuropathic supersensitivity. Understanding the time frame for experimental reversal of neuropathic supersensitivity (Lomo & Rosenthal, 1972; Lomo & Westgaard, 1975), it also provides a ‘when’—that is, a rationale for the expected length and course of treatment based on the severity of the physical examination findings. These and not the technique per se are what differentiate Gunn-IMS from DN. Gunn’s model recognises the ‘myofascial trigger point’ (TrP), but it recognises the TrP as just one of many clinical manifestations of RNMP. Because it is a radiculopathic model, it predicts the presence of TrPs in a myotomal distribution, including the posterior ramus, and recognises the importance of treating such points. Yet despite these differences Gunn-IMS practitioners share in common with all practitioners who treat MPS the recognition of both the prevalence of MPS and the success in treating it early and properly. Indeed the most important aspect of Gunn’s contribution is not even necessarily the technique of Gunn-IMS (although important), but that it will hopefully lead to wider recognition by the medical community of the significant incidence and prevalence of MPS in the general population. Epidemiological studies suggest that MPS is an important source of morbidity in the community (Cummings & White, 2001), yet it is commonly overlooked in the clinic (Skootsky et al., 1989). This is corroborated by the fact that it is found in 85% of patients seen in chronic pain clinics (Fishbain et al., 1989). MPS is the most common cause of chronic pain, and so ‘it is essential to authenticate that chronic refractory myofascial pain is…a ubiquitous neuromusculoskeletal disease resulting from spondylotic radiculopathies induced partial denervation with denervation supersensitivity’ and to recognise it as a ‘global public health disease’ (Chu et al., 2016). Despite all of the rich resources we have thrown at this problem by pursuing the standard paradigm of ‘spondylosis-nociception-inflammation’—strengthening exercise programs, imaging studies, spinal injections, surgery, multidisciplinary pain clinics, opioids, spinal cord stimulators, and pumps—we have ended up with increasing suffering, impairment, opioid dependence, disability, and unsustainable costs (Deyo et al., 2009). The only things we have not done is recognise and treat myofascial pain early and properly. By placing myofascial pain squarely within the pathophysiological schema and thus diagnostic algorithm of spondylotic pain, myofascial pain can be properly seen as the prevalent condition that it is. Clinical presentations of myofascial pain are protean in their manifestations: pain referral, although adhering to general patterns, is individually variable, inconsistent, and sometimes enigmatic, and it can be overshadowed by the nonspecific nature of the nonpain complaints referable to autonomic mediation that suggest primary visceral pathology (Fricton et al., 1985). All of these features make it difficult to standardise case definition, thus making diagnosis elusive. Gunn’s model accounts for this variability and provides an objective approach to the evaluation and treatment of these patients. Rather than a possible afterthought when the existing model fails, MPS will hopefully be moved to the forefront of the algorithmic evaluation of pain that persists for more than 3 to 6 months. With greater recognition and proper early treatment there is hope of stemming the global epidemic tide of chronic pain that is overwhelming Western medical systems. Yet, although Gunn-IMS is a treatment for myofascial pain, the RNMP model that it is employed within represents more than simply a technique for treating TrPs. It represents an entirely new way to understand, examine, and effectively treat patients with persistent pain. As such, his work represents a true paradigm shift. Gunn’s RNMP model provides a unified model of peripheral neuromusculoskeletal pain that points the way to improved treatment for these costly clinical and societal problems. Although Gunn has exploited CL in the service of treating pain primarily, the implications of this law and Gunn’s therapeutic model go beyond the treatment of neuromusculoskeletal pain. Although beyond the scope of this chapter, taken to its logical and inevitable conclusion, Gunn’s model proposes a rational basis for the treatment of syndromes caused by the autonomically mediated visceral epiphenomena of segmental radiculoneuropathy, including such varied complaints as vertigo, tinnitus, irritable bowel syndrome, and infertility, to name but a few. Current research interest in the role of the nervous system in chronic, or ‘parainflammation’, suggests even broader and significant implications of Gunn’s model (Tracey, 2002). Gunn-IMS is a procedure that can carry significant risks, especially when treating deeper paraspinal muscle contractures or anywhere overlying the lungs or near vascular structures. Despite these risks, properly qualified healthcare providers, both primary care and specialist, can be taught to apply it safely and readily to many of the most commonly encountered clinical problems. In addition, Gunn-IMS, similar to all DN techniques, is ‘low tech’, inexpensive, and easily employed in clinics worldwide. Yet although any practitioner can easily be taught to stick a pin into a muscle, as mentioned previously it is the understanding of ‘what’ may cause the TrP, ‘where’ and ‘how’ to treat the patient, ‘what’ responses are sought by needling, ‘why’ needling is likely effective, and ‘when’, or how often and for how long to treat the patient that constitute the proper application of Gunn-IMS. In seeking to understand his clinical findings, Gunn found an explanation in Cannon and Rosenblueth’s ‘The Supersensitivity of Denervated Structures, a Law of Denervation’. After the identification of segmental myalgic hyperalgesia (‘tenderness at motor points’) as a correlate of radiculopathy (Gunn & Milbrandt, 1976a), subsequent observations included the heretofore unrecognised neuropathic findings in these patients: increased muscle tone, neurogenic oedema, vasomotor disturbances with hypothermia, exaggerated pilomotor and sudomotor reflexes, and dermatomal hair loss (Gunn & Milbrandt, 1978). Cannon is credited with originating the concept of the ‘fight or flight’ response, introducing the term ‘homoeostasis’, and popularising the use of barium to visualise the gastrointestinal tract. He and Arturo Rosenblueth, former head of the department of physiology and pharmacology at University of Mexico, also performed animal research demonstrating the effects of motor nerve denervation. CL quantified experimentally the pathophysiological responses to somatic and autonomic motor denervation in a variety of target end organ tissues, including skeletal and smooth muscle, spinal neurons, sympathetic ganglia, adrenal glands, sweat glands, and brain cells. These reactions can all be described as forms of supersensitivity (i.e., abnormal tissue responses to stimuli) and although Cannon investigated the effects of motor denervation (techniques to study sensory receptors did not exist then), the phenomena of neuropathic supersensitivity first described by him is the same as that which we recognise clinically in peripheral sensory neuropathies (e.g., diabetic, alcoholic) as dysesthesia, allodynia, and hyperalgesia. In other words, stimuli that normally should not trigger a response now do: it is not the stimuli that are abnormal but the system that senses them. Cannon & Rosenblueth’s Law is summarised as: ‘When a unit is destroyed in a series of efferent neurons, an increased irritability to chemical agents develops in the isolated structure or structures, the effect being maximal in the part directly denervated’. Gunn, as a practicing physician, first recognised the clinical manifestations of CL: ‘This law is seldom cited to explain neuropathic pain; it deserves to be better known. It points out that the normal physiology and integrity of all innervated structures are dependent on the arrival of nerve impulses via the intact nerve to provide a regulatory or trophic effect. When this flow, which is probably a combination of axoplasmic flow and electrical input, is blocked, innervated structures are deprived of the trophic factor, which is vital for the control and maintenance of cellular function… A-trophic structures become highly irritable and develop abnormal sensitivity or supersensitivity’ (Loeser et al., 2001). All of the tissues studied by Cannon and Rosenblueth (skeletal and smooth muscle, spinal neurons, sympathetic ganglia, adrenal glands, sweat glands, and brain cells) develop denervation supersensitivity. Their research quantified this phenomena as: (1) increased susceptibility: lessened stimuli, which do not have to exceed a threshold, can produce responses of normal amplitude; (2) hyperexcitability: the threshold of the stimulating agent is lower than normal; (3) superreactivity: the capacity of the muscle to respond is augmented; and (4) superduration of response: the amplitude of response is unchanged but its time course is prolonged. Numerous animal experiments have confirmed that denervation supersensitivity is indeed a general phenomenon. In the muscle, the previous responses are demonstrated by a lowered threshold to acetylcholine (ACh) inducing a contraction. It has also been shown in both striated and smooth muscle that the surface area of the muscle fibre that is sensitive to ACh increases. That is to say ‘extrajunctional’ areas on the surface away from the zone of innervation, normally the only area receptive to ACh stimulation, now respond to ACh. This phenomenon is detectable 4 to 5 days after denervation and reaches a maximum within about a week, at which time the entire surface of the muscle fibre is as sensitive to ACh as the neuromuscular junction (Axelsson & Thesleff, 1959). Another manifestation of denervation supersensitivity in the muscle fibre is the development of spontaneous electrical activity called fibrillation. In contrast to an action potential in the muscle fibre occurring only in response to the release of neurotransmitter, action potentials now occur spontaneously due to changes in membrane potentials and conductivity. In electromyography, spontaneous depolarisations are called ‘denervation potentials’ and reflect loss of motor innervation; they are seen in diseases of the anterior horn cells, nerve roots, plexus, peripheral nerve, and muscle (Chu-Andrews & Johnson, 1986). They are manifestations of CL, reflecting the abnormally elevated sensitivity and reactivity of the muscle membrane to both ACh and the mechanical stimuli of the electromyography needle as it provokes depolarisation, a result of the disinhibiting effect of denervation. Significantly, in addition to the spontaneous depolarisations that produce action potentials, ACh slowly depolarises the supersensitive muscle membrane, inducing electromechanical coupling in which tension develops slowly without generating action potentials (Eyzaguirre & Fidone, 1975). Cannon and Rosenblueth’s original work was based on complete loss of motor innervation for supersensitivity to develop. Subsequently it became recognised that actual physical interruption and total denervation are not necessary: any injury or illness that impedes the flow of motor impulses for a period of time can rob the target organ of its excitatory input and cause supersensitivity in that structure and, significantly, in associated spinal reflexes (Loeser et al., 2001; Cangiano et al., 1977; Gilliatt et al., 1978). Supersensitive skeletal muscle fibres overreact to a wide variety of chemical and physical inputs, including stretch and pressure. This process of nerve dysfunction with impaired or interrupted neural impulses (neurapraxia with partial or complete conduction block from dysmyelination/demyelination) and at times associated with partial denervation (incomplete axon loss from axonostenosis, axonocachexia, axonotmesis, Wallerian degeneration) is not uncommon in adults and is known as ‘neuropathy’, or ‘nerve-sickness’, literally. It is important to recognise that such nerves can still conduct nerve impulses, can synthesise and release transmitted substances, and, in the case of motor nerves, can evoke both muscle action potentials and muscle contraction. The causes of neuropathy are legion and include congenital, neoplasms, inflammatory, traumatic, vascular, toxic, metabolic, infectious, degenerative, and idiopathic aetiologies. Commonly recognised neuropathies include the peripheral sensory neuropathies associated with diabetes, nutritional deficiencies, chemotherapeutic agents, alcoholism, and postherpetic; however, the far more common cause of nerve dysfunction is injury, especially that due to spinal strain and trauma, including acute, subacute, and chronic. Sciatica, a type of spondylotic traumatic compressive neuropathy, accounts for the highest incidence of all causes of neuropathy, five times that of the second most common cause, diabetic neuropathy (Bridges et al., 2001). Spondylosis is defined as the subacute or chronic (gradual, insidious) structural disintegration and morphological alterations in the intervertebral disc and pathoanatomic changes in surrounding structures that leads to damage of the nerve roots and spinal nerves (Wilkinson, 1971). In addition to disc degeneration and bulging, osteophyte and spur formation, ligamentous hypertrophy, spondylolisthesis, decreased disc height, and facet joint arthropathy all combine to cause narrowing of the spinal canal and intervertebral foramina leading to radiculopathy and myelopathy in some patients. Evidence of spondylosis on imaging studies is common: it is found in more than 50% of those over 40 and 85% of those over 60 years old, yet as many as 75% to 90% of asymptomatic 60- to 65-year-olds show significant disc degeneration at one or more levels, confirming the poor correlation between structural abnormality and symptoms (Kelly et al., 2012). The spinal nerve roots and spinal nerves are especially vulnerable to injury as result of anatomic features specific to these structures, especially the absence of the protective perineurium and epineurium around the roots; experimental research has documented the significantly lower compressive forces that the nerve roots are able to withstand compared with peripheral nerves before developing electrical conduction block (Sharpless, 1975). Axonal transport is blocked experimentally at pressures as low as 30 mm Hg, and the spinal nerves are limited to 15% elongation beyond their original length by stretching before developing intraneural blood flow and electrical conduction block (Rydevik et al., 1985); tethering by the dura, the intertransverse and mamilloaccessory as well as accessory ligaments further limit their mobility, with the mamilloaccessory a common source of entrapment neuropathy in patients with chronic low back and/or leg pain (Sihvonen, 1992). As little as 9 degrees of axial rotation will produce a myelographic filling defect at L5-S1 in normal subjects, with the angulation of the nerve over the edge of the vertebral body producing beyond normal physiological stretching of the nerve and potential neural deficit (Farfan, 1984). Spondylosis is thus associated with repetitive and cumulative compression, angulation, traction, and torsional injuries to the spinal nerves and roots, some leading to transient impaired intraneural blood flow, axonal transport, and electrical conduction block, and contributing to the development of neuropathic supersensitivity (Lorkovic, 1975; Gilliatt et al., 1978; Rydevik et al., 1985). Yet extrinsic neural compression may be absent, and the patient may have only partial denervation with intraneural fibrosis, with no evidence of a ‘pinched nerve’ on MRI and no evidence of acute denervation on EMG (Murphy, 1977; Sihvonen, 1992). Many of these cumulative injuries to the spinal nerves associated with reversible electrical conduction block may produce no or only transient symptoms (e.g., the ‘stinger’ in football) before the patient returns to an asymptomatic state, although subclinical neuropathic morbidity persists. When considered in its totality, spondylosis accounts for the most common form of neuropathy seen clinically: radiculoneuropathy, representing a far larger group of patients than those with radiculopathy, in which there are reflex and sensorimotor deficits on physical examination, a herniated disc seen compressing a nerve on MRI, and/or an abnormal EMG revealing acute denervation. Because the nerve roots and spinal nerves contain motor, sensory, and autonomic fibres, it follows that the clinical manifestations of injury to them reflect the effects of neuropathy that develop to varying degrees in each of these three components. Ochoa and colleagues have demonstrated experimentally that tourniquet compression selectively demyelinates larger diameter fibres, sparing the smaller diameter myelinated and unmyelinated fibres (Ochoa et al., 1972). Because the largest diameter myelinated fibres in the spinal nerve and roots consist of the alpha motor neuron efferents and proprioceptive muscle spindle afferents, these are typically the earliest fibres damaged by the spondylotic process due to compression, either transient or prolonged. As noted earlier, in neuropathic skeletal muscle ACh slowly depolarises the supersensitive muscle membrane, inducing electromechanical coupling of actin and myosin in which tension develops slowly without generating action potentials. Due to the extended time frame over which this occurs, no action potentials are seen on electromyography, and this shortening is called contracture rather than contraction (Eyzagguire & Fidone, 1975). In addition, Gunn has proposed that radiculoneuropathic involvement of muscle spindle afferent fibres leads to hyperexcitability of the muscle spindle mechanism, potentiating the length-regulating feedback mechanism of the gamma loop and contributing to the development of these contractures (Gunn & Milbrandt, 1977). This mechanism may be amplified even further by sympathetic supersensitivity activating intrafusal fibres of the muscle spindle (Chu, 1995). Dysfunction of this mechanism is sometimes referred to as the ‘facilitated segment’, ‘somatic dysfunction’, or the ‘osteopathic lesion’ (Korr, 1975). Consistent with the experimental evidence showing the earliest involvement of nonnociceptive large diameter myelinated fibres (alpha motor neuron and proprioceptive muscle spindle afferents), it is noteworthy that muscle contracture and hyperexcitability of the muscle spindle produce the earliest symptoms the patient often reports: these are typically not the pain we associate with nociception (i.e., sharp, or even dull and aching). Early symptoms associated with MPS are often described as stiffness or tightness and have a peculiar cramplike sensation. This is the same phenomena seen in significant numbers of patients diagnosed with irritable bowel syndrome (IBS), who experience sensitivity to abdominal distension that is perceived as pain: hypersensitivity of the mechanoreceptors for stretch and contractile tension are implicated in this entity, although involving smooth muscle rather than striated muscle (Johnson, 2012). So, just as ‘all that glitters is not gold’, ‘all that ‘pains’ is not nociception’. Muscle spindle dysfunction also results in impaired proprioceptive and neuromuscular function, producing impaired standing balance or cursive handwriting; these symptoms even often preceding the onset of ‘painful’ symptoms. On physical examination these muscle contractures are palpable in the more superficial muscles and are commonly referred to as ‘taut bands’, ‘ropy bands’, or ‘contraction knots’ (Baldry, 2001). Deeper, nonpalpable contractures are what Gunn terms ‘the silent lesion’ (Gunn, 1996). Over time, when enough regions of the muscle develop contractures, the muscle’s overall resting length shortens, at which point the patient may become aware of decreased flexibility, noting, for example, the need to turn their upper body to check automotive traffic behind them, as the active range of motion in the cervical spine is diminished. As the process of spondylosis advances with additional acute injuries superimposed on preexisting subclinical neuropathy, the model postulates that small diameter nerve fibres eventually develop supersensitivity leading to myalgic allodynia (tenderness to light touch/palpation that is normally nonpainful) and hyperalgesia (elevated levels of pain to light touch/palpation that is normally painful). The patient may still not complain of pain but may report impaired balance, stiffness, and decreased flexibility; the patient is often surprised by the pain elicited by palpation of these tender contractures, or ‘latent TrPs’ (Sola et al., 1955; Baldry, 2001). It is this morbid but generally pain-free phase that Gunn terms ‘prespondylosis’ (Gunn et al., 1980). With progressive sensitisation of small diameter nociceptor fibres and muscle shortening compressing the intramuscular type III and IV nociceptors, the patient develops ‘active TrPs’ and complains of pain at rest, in the absence of either external nociceptive stimuli or tissue inflammation. Lactic acidosis and other biochemical changes in the local milieu of the trigger point (Shah et al., 2008) chemically sensitise the nociceptive fibres further, contributing to the pain. When radiculoneuropathy involves the autonomic division of the spinal nerve, vascular, smooth muscle, pilomotor, sudomotor, and trophic responses are exaggerated. Although one of the clinical hallmarks of inflammation is warmth/heat, neuropathy results in vasoconstriction and relative coolness of affected segments. Because Cannon’s Law applies to smooth muscle fibres as well as striated skeletal muscle, they respond similarly to denervation and neuropathy by developing shortening contractures. The architecture of smooth muscle is distinct, however, from the arrangement of skeletal muscle fibres, which lay parallel to each other. The smooth muscle cells lining vascular, lymphatic, and visceral organs are arranged adjacent to each other, in sheets or layers; when they develop shortening contractures, the potential space between adjacent cells (‘cell gap’) increases, and intraluminal fluids leak out, creating neurogenic oedema (recognised as ‘cellulite’ when located in the hip girdle and thighs). Neuropathy induced smooth muscle contractures also result in impairment of efficient synchronous muscular contractile function, which can produce symptoms that can be mistaken for primary visceral pathology. Depending on the spinal segments involved, otolaryngological, cardiorespiratory, gastrointestinal, and genitourinary signs and symptoms may occur, including dizziness, bronchospasm with neurogenic oedema/ARDS, ‘irritable’ bowel, urinary frequency-urgency, and even infertility due to fallopian tube smooth muscle dysfunction precluding passage of the ova. The quality of collagen in soft and skeletal tissues is degraded in neuropathy with replacement collagen weaker due to fewer crosslinks. With muscle contractures creating increased mechanical tension on these weaker ligaments, tendons, cartilage, and bones, neuropathy expedites degenerative wear and tear, especially in activity-stressed parts of the body, causing ‘spondylosis’, ‘discogenic’ disease, and ‘osteoarthritis’. Although these entities continue to be regarded as primary diseases, they are the ‘down-stream’ cumulative effects of RNMP. Enthesopathy, thickening of the musculotendinous junction, is also seen as a result of and possibly compensation for the factors mentioned previously. Autonomic neuropathy also leads to exaggerated sudomotor and pilomotor responses, producing hyperhidrosis and ‘goose bumps/flesh’. The presence of dermatomal rather than stocking distribution hair loss (as seen in peripheral neuropathy) is another common finding, attributable to trophic changes. Gunn refers to the constellation of these autonomically mediated signs and symptoms as the ‘epiphenomena’ of spondylotic neuropathy. The combined motor, sensory, and autonomic effects of RNMP manifest as all manner of subjective complaints, including coolness, paresthesias (including tingling, buzzing, vibration, pressure), dysesthesias (including ‘pins and needles’), neuralgic pain (shooting-stabbing-lancinating-paroxysmal), and itching (Stellon, 2002). Complaints of unsteadiness, dizziness, stiffness, weakness, fatigue, and swelling are attributable to muscle shortening, muscle spindle dysfunction, and neurogenic oedema. Physical findings may include impaired single leg standing balance, decreased range of motion, coolness to touch, hyperhidrosis, goose-flesh, dermatomal hair loss, myalgic hyperalgesia with generation of referred pain, and either spontaneous or elicited local fasciculation, known as the ‘local twitch response’ (LTR). There may be cutaneous hypoesthesia and allodynia, rather than anaesthesia which is present in denervation. Over time RNMP leads to both localised intramuscular contractures and shortening of the resting length of the muscle, as well as the persistent increased mechanical tension on the musculotendinous attachments to bone leads to what previously was labelled tendonitis, implying an inflammatory aetiology, now preferably called tendinopathy or tendinosis (Khan et al., 1999). These include most of the subacute and chronic tendonitis and bursitis syndromes (Achilles, extensor forearm, bicipital, rotator cuff, DeQuervain’s tenosynovitis, patella, gluteal) as well as such entities as iliotibial band syndrome, chondromalacia patellae, muscle tension headache, pyriformis syndrome, plantar fasciitis, and temporomandibular joint. Many of these are thus seen as the effects of muscle shortening that, due to increased tension at the periosteum, create pain as well as bone spurs according to Wolff’s Law, which describes the process of bone deposition in response to mechanical tensile forces. Thus extensor elbow tendinosis, or ‘tennis elbow’, is seen not as a local pathology but the ‘downstream’ effect of subacute and chronic C6-C7 radiculoneuropathic–myofascial pain that can sometimes be treated successfully by treatment to the cervical spine alone (Gunn & Milbrandt, 1976b). The constellation of symptoms and signs noted previously collectively constitute a picture and definition of what is called MPS, myofascial pain being a term popularised by Dr. Janet Travell, who also ‘popularised the use of the term TrP (Baldry, 2001). The pathognomonic feature of MPS is the TrP, the hallmark of which is hyperalgesia with referred pain, and which is ‘structurally…made up of a collection of dysfunctional motor endplates, juxtapositional contraction knots and neurovascular bundles with each containing blood vessels and contiguous sympathetic fibres; a motor axon and its nerve terminals; and sensory afferents attached to proprioceptors and nociceptors’ (Baldry, 2001). Whereas Gunn’s model recognises TrPs as most often found beneath motor points and the importance of directing treatment to these points (Gunn et al., 1980), the TrP is seen as just one of the many diverse manifestations of radiculoneuropathic–myofascial pain syndromes. It recognises that needling can ‘occasionally actuate muscle to fasciculation: this is usually accompanied by near-instantaneous muscle relaxation’ (LTR). It also recognises that, due to supersensitivity, the entire surface of the neuropathic muscle may respond to needling. Penetration into almost any part of the muscle can lead to relaxation, but the most rewarding sites are at tender and painful points in muscle bands (Gunn, 1989). Gunn’s finding of a correlation between tender motor points and electromyographic (EMG) evidence of partial denervation radiculopathy has been corroborated by Chu using a semiquantitative motor unit action potential (MUAP) EMG technique (Chu, 1995). Although EMG abnormalities were found in a myotomal distribution correlating with clinical findings of MPS, Chu suggested that single-fibre EMG (SFEMG) may be more useful than conventional EMG in establishing the cause of abnormalities as ‘neurogenic, myogenic, or otherwise’. The presence and severity of motor neuroaxonal degeneration correlating with TrPs and disease duration using SFEMG technique has been established by Chang, who has also found evidence of spinal accessory neuropathy in cervical MPS (Chang et al., 2008, 2011). Clinical studies (Letchuman et al., 2005; Sari et al., 2012) have also confirmed the association between (cervical) radiculopathy and myofascial tender points as originally demonstrated by Gunn (Gunn & Milbrandt, 1976a). Cannon originally experimentally documented neuropathic supersensitivity from motor denervation in both the peripheral (skeletal muscle/neuromuscular junction, autonomic ganglia) (Cannon & Rosenblueth, 1936) and central (brain, spinal neurons) (Cannon & Haimovici, 1939) nervous systems, defining it quantitatively using the neurotransmitter acetylcholine. Since then the phenomena of central sensitisation that develops in response to peripheral nociception and/or nerve damage has been further elaborated experimentally, with evidence of biomarker, neurotransmitter and neuroplastic changes in the dorsal horn, cingulate nucleus, hippocampus and other regions of the CNS leading to amplified and sustained pain in the absence of ongoing peripheral nociception; it has been precisely defined as an ‘amplification of neural signaling within the CNS that elicits pain hypersensitivity’ (Woolf, 2011). Partial deafferentation of the CNS (as in radiculoneuropathy) can result in both chronic ‘central’ pain (Loeser, 2012) and peripheral findings of supersensitivity. Gunn’s localisation of the pathology to the spinal nerve therefore accounts for both retrograde proximal–central and anterograde distal–peripheral neuropathic abnormalities. Clinically, however, the central and peripheral nervous systems function simultaneously and seamlessly as an integrated system and cannot be separated—research has confirmed the presence of peripheral sensitisation manifesting as trigger points in many syndromes associated with central sensitisation, contributing to the cluster of signs and symptoms seen in these patients (Dommerholt, 2011). What then is the evidence that Gunn-IMS can reverse RNMP? Evidence that neuropathic supersensitivity can be reversed was demonstrated experimentally by Lomo, who showed that ACh supersensitivity in denervated animal skeletal muscle could be abolished by graded electrical stimuli (Lomo & Rosenthal, 1972; Lomo & Westgaard, 1975; Thesleff, 1976). Fig. 15.1 shows how experimental denervation affects the sensitivity of a muscle membrane to ACh (bold line). Additionally, Fig. 15.1 shows how this hypersensitivity returns toward normal after electrical stimulation and does so more quickly with continuously applied stimuli. Gunn has proposed that the ‘current of injury’ (the measureable electrical microcurrent associated with damage to a cell wall membrane) created by the minor muscle fibre trauma of needling provides an intrinsic source of electrical stimulation that facilitates reversal of neuropathic supersensitivity similar to that provided exogenously by Lomo (Gunn, 1978).
Intramuscular Stimulation
Introduction
Neurophysiological mechanism of gunn intramuscular stimulation
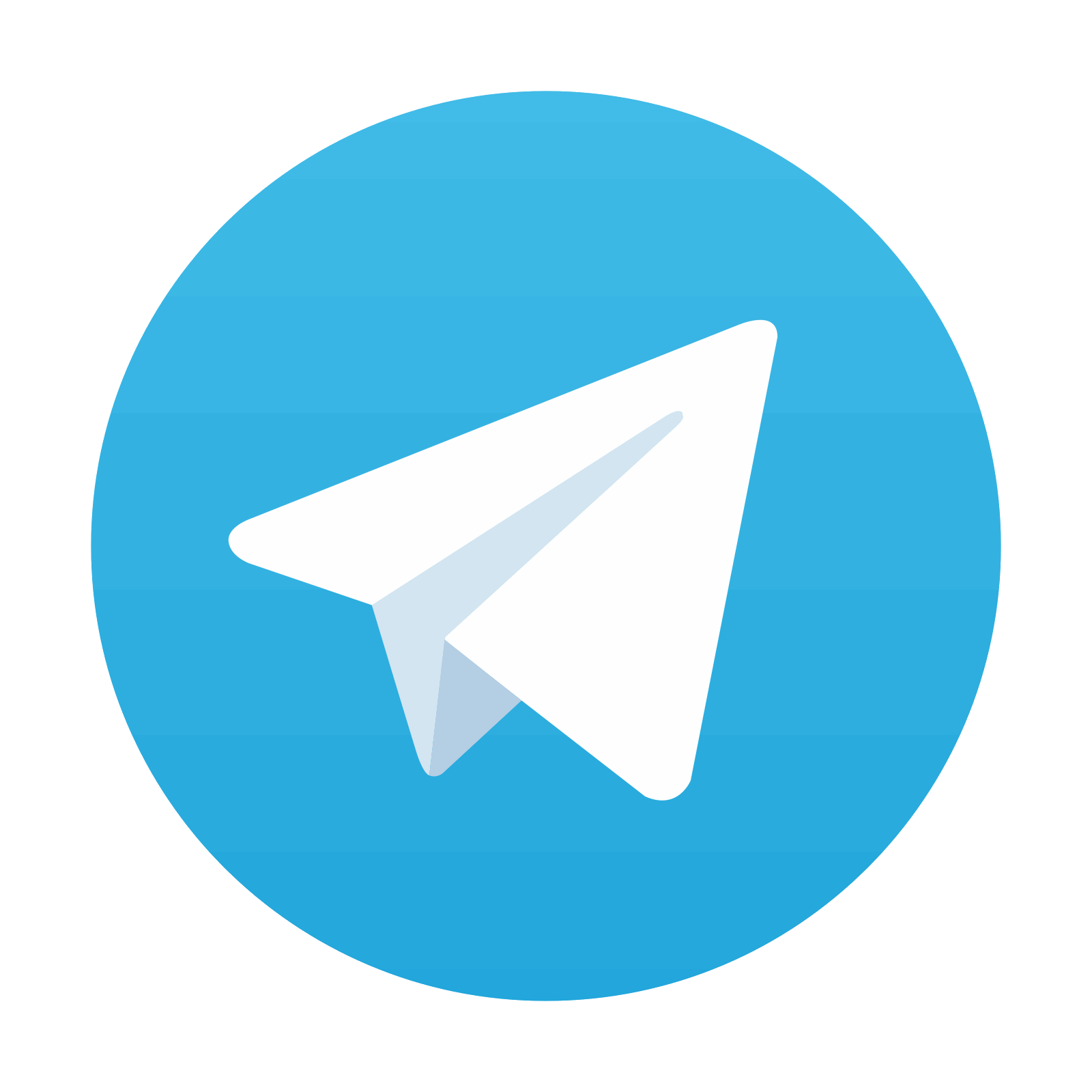
Stay updated, free articles. Join our Telegram channel

Full access? Get Clinical Tree
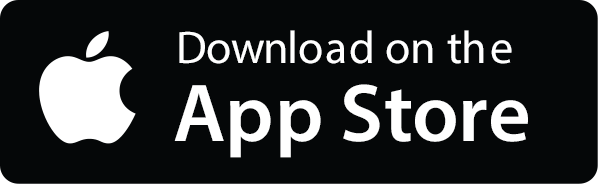
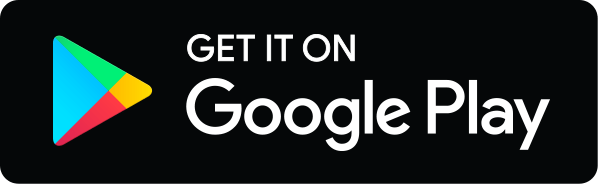