Joint replacement (%)
All fractures (%)
Staphylococcus aureus
12–23
30
Coagulase Negative Staphylococcus
30–43
22
Gram Negative Bacilli
3–6
10
Anaerobes
2–4
5
Enterococci
3–7
3
Streptococci
9–10
1
Polymicrobial
10–12
27
Unknown
10–11
2
Implant Infection and Epidemiology
Fixation methods have evolved in the past century to provide surgeons with a variety of methods to stabilize a fracture. As our understanding of bone healing has become more sophisticated, so too has our implant selection process become more elaborate. Just as implant design influences bone healing, these same characteristics impart a susceptibility to complications [2]. Copious amounts of literature have explored the effects of implant architecture, surface interaction, and material constitution on infection resistance; but adequate discussion of that topic is a chapter unto itself. In this chapter we will briefly discuss some relevant implant features encountered on a routine basis.
Several studies have demonstrated that surface nanotopography influences susceptibility to infection. In one bench top study, a porous coated cobalt chromium implant required 40 times lower inoculum of bacteria to generate osteomyelitis than its smooth polished control. Yet another study reported greater infection resistance in textured titanium implants as compared to a smooth polished stainless steel control [8]. While surface topography is an important feature, it appears that implant material, which confers biocompatibility, is a more significant consideration.
The most common materials used in fixation or joint replacements are electropolished stainless steel (EPSS) or titanium. Titanium implants have the potential of direct anchorage to bone via a process called osseointegration. Implants that undergo osseointegration connect directly to living bone and have enhanced structural stability. This is in contrast to EPSS implants, where evidence suggests that a thin fibrous tissue is interposed between the device and bone. The presence of any barrier further inhibits circulation and penetration of immunocompetent elements into an already isolated space. This is more common among EPSS materials than titanium [7, 9, 10]. Some authors have concluded that titanium is less susceptible to infection and colonization because its biocompatibility profile also reduces tissue reaction and has less corrosion than traditional stainless steel fixation [11].
Implant architecture is another feature that influences infection potential. Cannulated devices have an intrinsic dead space that is susceptible to colonization and growth. Studies of intramedullary nails found that solid devices were less likely to develop an infection compared to the cannulated designs. Subsequent investigations found that reamed intramedullary devices nails were more likely to become infected than unreamed implants. The authors concluded that unreamed nails better preserve the medullary blood supply and minimize the deadspace [1]. Continuing with the theme of preservation of native tissue, consider the role of plate fixation. Dynamic compression plates (DCP) compress tightly to bone which often leads to necrosis of adjacent periosteum. In a preclinical comparison study conducted by the AO foundation, investigators found that a locking plate design imparts less pressure on the underlying tissue and was ten times more resistant to infection than the DCP [7].
In addition to design characteristics and technique, the function of the implant also impacts the epidemiology of infection. For example, reports indicate that prosthetic joint implants are more prone to infection by gram-positive cocci than gram-negative rods [1]. Even within the domain of prosthetic implants variation exists; where larger weight bearing joints have higher infection rates than smaller and non-weight bearing implants, referring to those of the hand and shoulder versus ankle and knee [1].
The setting of implantation is very important and has a significant impact on the risk of infection. Few studies truly examine the long-term outcomes of overall surgical site infection (SSI) with fixation, however studies indicate that elective surgery carries a lower risk of infection than trauma-related surgery. Roughly 5 % of all inserted internal fixation will become infected including all types [4], and that is of great concern to any surgeon. A large, single center retrospective analysis of all patients undergoing “clean” elective podiatric surgery over a 7-year period found a surgical site infection (SSI) rate of 3.1 %. They reported that 71 % of the cultured pathogens were coagulase positive or negative staphylococcus and an alarming 87 % were resistant to penicillin or ampicillin [12]. Their analysis found that cases that included internal fixation had a statistically significant higher risk for developing post-op infection. A large case–control trial used a multiple logistic regression model to determine that the odds of prosthetic joint infection were 36 times greater in the setting of a SSI [OR: 35.9; 95 % confidence interval 8.3–154.6] [13]. Recent years have seen an increased interest in foot and ankle joint replacements. Technological advancements, particularly in total ankle replacements (TAR), have increased the relevance of periprosthetic joint infection (PJI) to foot and ankle surgeons. PJI is considered to be among the most devastating of prosthetic complications because the majority of infected implants will go on to failure [5, 14]. A 2012 case–control study evaluated the risk factors for periprosthetic ankle joint infection in 130 cases. Risk factors such as any prior surgery in the joint, procedure duration, and low preoperative functional ability were all statistically significant. Among the most significant risk factors for PJI they identified persistent wound dehiscence (OR: 15.38) and wound drainage (OR: 7.0). Conclusions of that study were that prior surgery at the site increased infection by greater than 4.5 times, dehiscence increased chances of wound infection by 15 times, and infection was 7 times more likely with a draining wound [15].
It is contextually important to distinguish between the “clean” elective procedures discussed above and the less controlled environment of trauma. In the setting of traumatic injury, preventing SSI or implant associated infection becomes much more complicated and is heavily dependent on whether it is an open versus closed fracture pattern. Research suggests that trauma cases are 2.5 times more likely to develop SSIs than elective cases [16]. Severity of trauma is strongly related to implant infection risk with closed fractures reported as having an incidence of 1–2 % infection versus infection rates as high as 30 % in open fractures [17]. Focusing on ankle trauma, a database study of 57,183 patients reported that open injuries posed a four times greater risk of developing post-op infection as compared to closed fractures following ORIF [18]. To further elaborate the effect of soft tissue integrity, consider the following outcomes. Internal fixation of tibial fractures via an intramedullary nail has demonstrated infection rates of 2–3 % in closed fractures. In the presence of an open fracture the incidence jumps to 21 %, and in the setting of severe soft tissue damage the incidence of SSI has been as high as 50 % [7].
When dealing with open injuries it is always prudent to focus on the amount of soft tissue destruction and potential coverage. A further complicating issue is the necrosis, perfusion limitations, and lack of immune system ability to reach edematous or severely disrupted soft tissue envelopes [7].
Tenants of Treatment
The previous sections of this chapter have presented the epidemiology, pathogenesis, and risk factors of infected implants. The remaining sections will focus on treatment principles and protocols that enhance outcomes. Because other chapters provide in-depth discussion of imaging and laboratory-based diagnosis/monitoring of bone infections, this chapter will minimize those features. Similarly, specific antibiotic agents and surgical excision extensively covered elsewhere in this book will be limited to their role in the overall treatment paradigm of infected implants.
Much of current treatment practices are an amalgam of traditional teaching, anecdotal evidence, and medicolegal considerations. Just as individual variation exists, so too is there significant variety of treatment protocols between institutions and countries [5]. Despite a diversity of settings and patient factors, the primary management objectives are: elimination of the infection, promote osseous stability, and optimize patient function in the context of the intended original procedure. Management of infected implants and nonunions require a multidisciplinary collaboration that integrates local and systemic antimicrobial therapy with adequate debridement, wound, and dead space management while anticipating the need for osseous stabilization in the setting of ununited bone segments and large deficits with bone graft.
Before discussing specific management protocols we need to consider factors that alter treatment. The importance of timing from the implantation, vector of infection, and host risk factors have been established by countless publications and often direct decision making.
Although there is overlap with treatment approach in a variety of situations, there are other areas where divergence is significant. Merging current practices into a cogent protocol that encompasses every clinical scenario is an impossible task. Instead we’ve consolidated the treatment protocols by the surgical setting and implant’s functional objective, specifically relative to the purpose of the implant within the body. We refer to this as the Intended Original Procedure. For instance, when dealing with osteotomies, fractures, and fusions, the therapeutic goals are fundamentally similar. In these settings the implants are intended to promote osteosynthesis, maintain appropriate alignment of osseous segments and rigid stability in an effort to restore or optimize function. Beyond similar therapeutic goals, bone healing in fractures and osteotomies can be treated as equivalent since the process of vascularization and bone formation are analogous despite variations in the details of healing [19, 20].
The other implant setting we will discuss is that of prosthetic joints. Like the previous example, these implants seek to provide good alignment but desired stability is related to the periarticular-implant interface which preserves function of the joint. Prosthetic joints don’t require osteosynthesis to achieve their objectives, but compromise of the interface leads to instability and diminished function. The management tenants of these two broad groups not only have several areas of overlap but also significant differences. We will highlight similarity and diversity of treatments later in the formal protocols.
Timing and vector of colonization are innately connected, therefore they are presented here in concert to add to the overall context. Much of the knowledge base regarding these two factors is derived from the prosthetic joint literature and the following discussion will reflect that fact.
The method of infection dissemination aids in identifying the pathologic organism. All surgical implants are vulnerable to two methods of implant colonization: exogenous and hematogenous. Exogenous refers to an inoculation from outside of the body that can occur during the implantation procedure itself or during a subsequent procedure and it usually arises within 2 years postoperatively [1, 21]. An example is postoperative arthrocentesis of the joint. An infection is classified as acute when it arises within just days and is usually due to Staphylococcus aureus; or subacute that occurs within weeks and is likely due to Coagulase Negative Staphylococcus [1]. The hematogenous vector occurs when the implant is seeded from another source in the body via the blood stream at any point after surgery [1]. As noted earlier in the chapter, prosthetic implants are at a lifelong risk of colonization and many reports have concluded that any presentation of infection more than two years post-implantation is likely due to hematogenous spread [5, 13, 21–23]. The risk of hematogenous colonization increases in the setting of bacteremia; studies report the rate of PJI as 34–39 % while all other nonarticular implants were shown to have a 7 % chance of seeding [1, 5, 23, 24].
For PJI, the classes are defined as early, delayed, and late [1, 5, 21, 23]. In the early phase, within 1–3 months of joint replacement, the origin is exogenous and it is likely that infection was seeded during implantation. The delayed phase is any time from 3 months up until 2 years postoperative. Clinical presentation typically includes vague symptoms of chronic pain in the absence of systemic signs of infection [22]. It is important to note that any painful prosthetic could represent a PJI or aseptic loosening, and therefore diagnosing and treating this is heavily relied on clinical judgment [21]. Seeding of delayed infection is thought to be through an exogenous source that was acquired during implantation. The late phase of PJI is characterized by onset of greater than 1–2 years postoperatively. The two main causes of late infection are described as hematogenous spread or a late manifestation of infection acquired during insertion. This will present with a more acute and abrupt onset of pain and symptoms and can be accompanied by a concomitant infection at another site in the body [21]. Statistics for elective surgery show that rates of hematogenous spread of infection for total knee or hip are around 1–2 %[21] while elbow or ankle arthroplasty are >5 % with primary implant surgery [1]. In support of that data, a study by Kessler of 26 infected ankle replacements found that 4 (15 %) were seeded by hematogenous origin and the remaining 22 (85 %) were through exogenous means [15]. The overall risk of hematogenous spread to a prosthetic joint is estimated to be 0.25–0.5 % per year, per prosthesis [1]. This points out the lower likelihood of hematogenous spread, however it is a real entity that cannot be overlooked.
The timing of infection in nonarticular fixation is similar to but also differs from that of prosthetic joints. The familiar early, delayed, and late presentations remain, however the time periods of onset are very different as are the most common organisms involved [25]. The early period represents less than two weeks and is described as acquired preoperatively during the trauma or during the implant surgery. These are often high virulence organisms, most commonly Staphylococcus aureus and Gram-Negative Bacilli such as E. coli [26, 27]. The delayed time frame is between 2 and 10 weeks, delayed and late manifestations are similar in presentation, course, and outcome and therefore grouped together in this discussion [28]. The late presentation is represented by greater than ten weeks and can be acquired during the trauma, implant surgery, or postoperative wound-healing period. This is described as being caused by an organism of low virulence such as Coagulase Negative Staphylococcus or Propionibacterium Acnes. In comparison to prosthetic joint replacements, infection of fracture fixation is rarely caused by hematogenous spread as described above at 7 % versus nearly 40 % of prostheses [24]. The type of pathogen involved in these infections also differs by type of fixation as discussed earlier. A large consecutive study of 132 internal-fixation device-associated infections found that the most common infectious agent was Staphylococcus aureus accounting for 30 % of the infections. Staphylococcus aureus is most commonly introduced during the preoperative initial trauma or when placing percutaneous fixation [4]. Coagulase negative Staphylococcus represented 22 % and Gram-negative Bacilli 10 % of the total isolated pathogens with greater than one pathogen isolated in 27 % of the cases [4].
Foundations of Treatment
Every protocol presented in this chapter will start with the same basic step: incision and drainage with adequate debridement. This stage which we will refer to as the initial debridement and is the foundation of our care. The data obtained during this phase will direct all aspects of the comprehensive treatment plan. The initial debridement will: (1) Identify the infectious organism, (2) Excise nonviable tissue and abscess, (3) Stabilize osseous segments, (4) Control infection via culture-directed antimicrobials, (5) Determine the necessity of soft tissue and dead space management.
The decision to perform the initial debridement is dependent on several factors, one of the most important is overall status of the patient. An example is a case where a patient treated with a foot or ankle implant has been hospitalized for sepsis. Infectious disease and the critical care team are unable to identify the source of the infection but suspect that the retained implant may be the nidus. The patient is immunosuppressed and therefore the absence of local signs of infection is unreliable. The medical team believes that the implant must be excluded as the source and determine that the patient is stable enough for incision and drainage with debridement and removal of the implant. This is a clinical scenario that you’ve either encountered or will encounter at some point in your career. In these instances it is advisable to perform the initial debridement because of the life-threatening consequences. Even if the implant is not the source, the procedure is not wasted effort. The likelihood of a patient with documented sepsis seeding an implant is quite high at just under a 40 % and removal may prevent a future occurrence [1]. Aside from this scenario, the decision to perform the initial debridement is unclear.
Once the decision is made to proceed with initial debridement, performance of the five foundations is critical to an optimal outcome. Other authors have discussed the importance of intraoperative cultures so our focus will be on optimal collection practices as they relate to infected implants and nonunions. During the course of debridement, tissue samples should be collected for aerobic and anaerobic cultures and pathology. Specimens should be collected from deep sources and sinus tracts are notoriously an unreliable culture source when present. If there is a communicating sinus tract or long-standing chronic ulceration then that specimen should be sent to pathology separately to evaluate if malignant transformation has occurred [17]. If circumstances dictate that the implant should be removed, then it should be submitted for microbiologic culture as well. But sensitivity of implant derived cultures can be imprecise. One emerging method of enhancing sensitivity is implant sonication. This technique can dislodge bacteria from the implant and is requested when submitting the implant for cultures. One study of hip and knee infections found that sonication had a sensitivity of 78.5 % while traditional culture only had a sensitivity of 60.8 % in the same patients [21].
Identification of the organism is so important that current recommendations are to withhold antibiotics for 2 weeks prior to debridement in order to increase organism yield from intraoperative samples if medically possible. Many resources advise that intraoperative cultures should be taken from multiple sites to improve accuracy, but the anatomic restrictions of the foot and ankle may restrict this methodology. Finally, in situations where the diagnosis of infection or aseptic loosening is unclear, it is possible to send specimens for frozen section to determine a rapid assessment [21]. This is easily accomplished at most institutions and can have significant repercussions on the treatment plan. Regardless, some organisms do take longer than others to grow in culture so it may be prudent to wait 14 days prior to finalizing plans with the patient.
Debridement has already been discussed in other chapters but will be briefly discussed in the context of this chapter. Aggressive and thorough debridement is an important part of the process since it reduces bioburden, eliminates the nidus of infection and eliminates nonviable tissue in the local environment [15, 29]. Inadequate debridement can lead to spread of the infection and additional tissue loss. It also predisposes the patient to chronic infection and its attendant sequelae. Multiple debridements may be necessary; in fact, a study by Patzak has reported that 26 % of cultures remained positive after the initial debridement [30]. Our experience has been that the more extensive the infection, particularly with gross purulence, that multiple debridements are necessary. This should not be viewed as poor or indecisive care; rather it reflects the difficulty in achieving balance between adequate debridement and tissue preservation.
Where appropriate, early debridement can disrupt biofilm formation or eliminate it completely when performed early and extensively [15]. This specifically involves opening the area, debriding infected soft tissue and washing out the implant only [22]. Occasionally debridement is so extensive that even with stable osseous segments, the potential of iatrogenic fracture becomes a concern. Several studies have concluded that even in the setting of gross stability, if debridement reduces cortical volume to less than 70 %, structural integrity is compromised and must be augmented [31–33]. Several options are available in this situation. Cast immobilization is simple but imprecise. It lacks compression, and can hamper wound care. The latter limitation can be ameliorated by bivalving the cast but at the cost of stability. A more physiologic stabilization is external fixation which imparts stability via wires and pins remote to the infected area (Fig. 8.1). It is superior to cast immobilization in that it provides precise stabilization with the ability for compression and permits wound care. The primary disadvantages are that it is costly, technically demanding, and is frequently poorly tolerated by patients. These same stabilization options are also appropriate in the setting of unstable bone segments. Although stability is obviously important in terms of preserving function; what may be less evident is that stability is beneficial for control of infection.
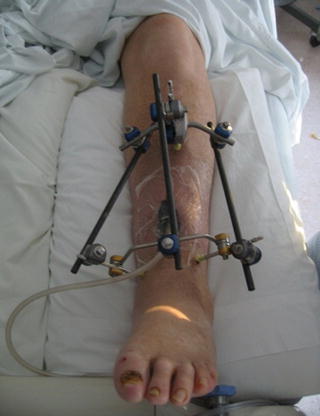
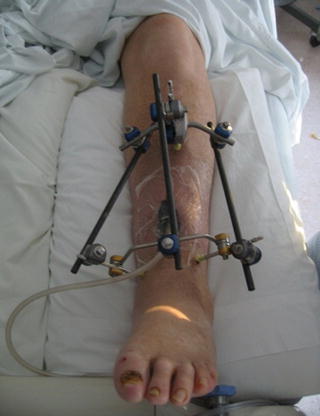
Fig. 8.1
Large segmental bone loss can lead to instability which threatens successful outcomes. External fixation provides excellent stability in a variety of settings and permits inspection of the soft tissue envelope
Preclinical research demonstrated that even with direct inoculation of a fracture site following surgery, unstable fractures were more likely to develop infections. Investigators concluded that fixated fractures were less likely to develop osteomyelitis than unfixated fractures [34, 35]. Worlock expanded on this work and used Staphylococcus aureus contaminated implants to determine the effect of rigid versus unstable fixation. He reported that less stable unlocked intramedullary pins had double the risk of developing osteomyelitis than the rigid plate fixation [36]. Earlier work by Friedrich et al., reported that severity of instability impacted infection rate and further stated that there was no observed difference in time to union between infected and uninfected fractures stabilized with rigid fixation [37]. The previous studies highlighted the importance of stability in preventing infection and the Friedrich study evinces that bone healing can occur in the setting of infection given adequate stability. This is particularly pertinent in the early postoperative phase of an osteotomy, fracture or fusion where fixation is critical to stabilization. Currently available evidence generally indicates that as long as a device provides rigid fixation, its role as a nidus and impediment to immunologic response is superseded by the stability it imparts [17, 38]. The consequences of stability on decision making are a good segue into actual treatment protocols.
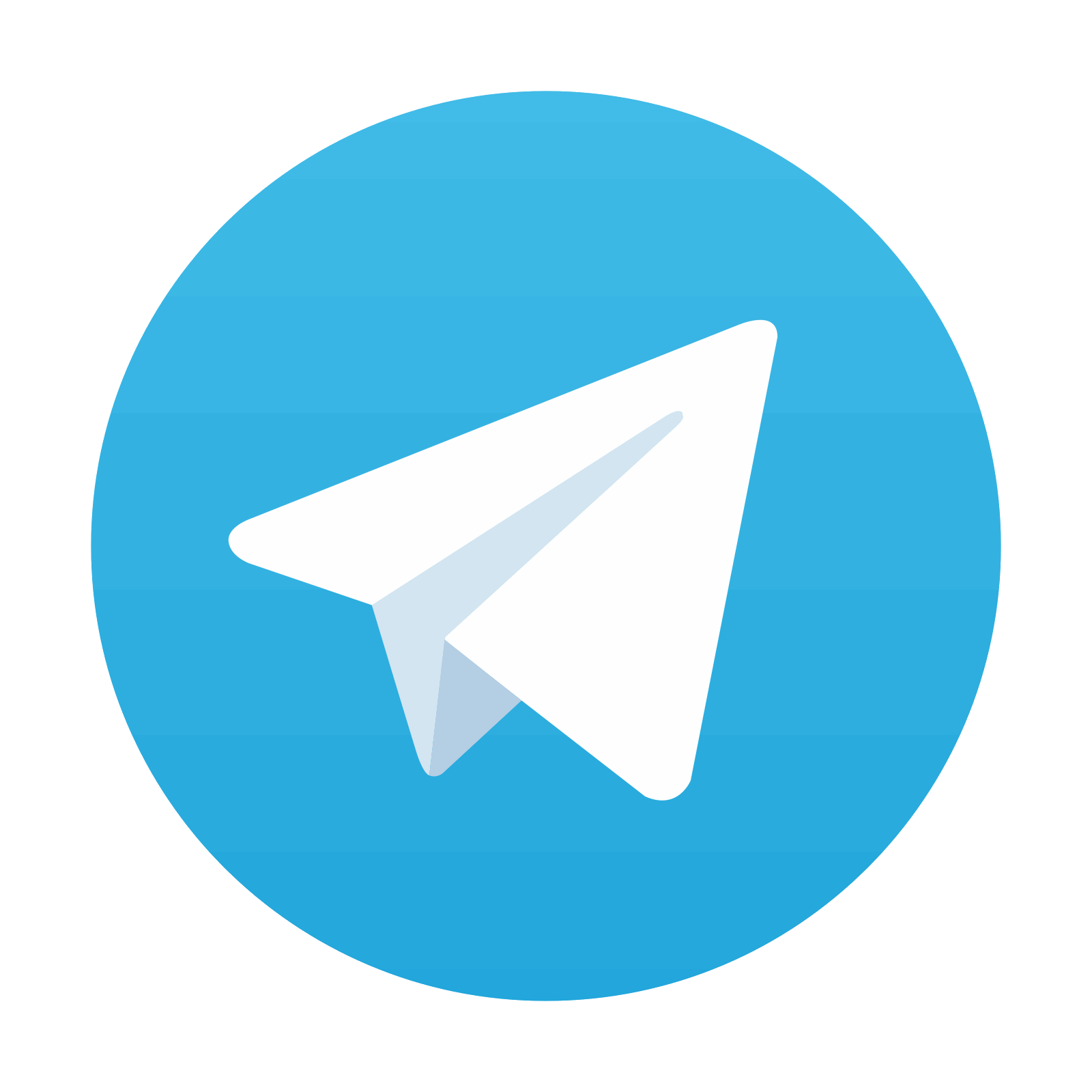
Stay updated, free articles. Join our Telegram channel

Full access? Get Clinical Tree
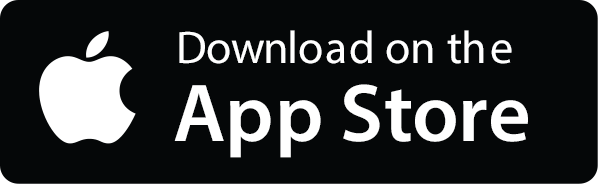
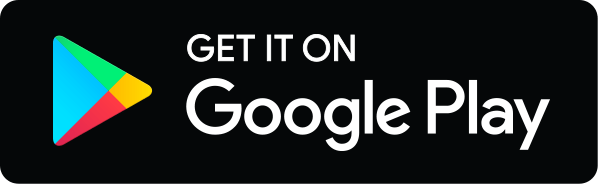