Miguel E. Cabanela
Impaction Grafting of the Femur
OVERVIEW
The incidence of revision total hip arthroplasty is increasing,1,2 and the number of patients requiring management of bone deficiencies during revision arthroplasty procedures has increased markedly.3 As hip replacement technology is applied to younger and more active patients, the multiply revised patient with poor femoral bone stock is becoming increasingly prevalent. Introduced into clinical practice over 20 years ago, the use of packed morselized bone in revision hip surgery to treat bone stock deficiency of the femur has become an important and accepted technique that offers some distinct advantages. Although the use of uncemented stems with diaphyseal engagement is the mainstay of revision femur work, impaction bone grafting is a valuable technique that offers the potential for restoration of bone stock and is particularly useful when faced with a large ectatic femoral metaphysis or diaphysis.
The basic concept of femoral impaction grafting involves tight packing of contained cavitary defects with compressed particulate bone graft to support the subsequent insertion of a prosthetic implant. A femoral component is then cemented into a mechanically supportive neomedullary canal. While fairly straightforward in concept, this technique is technically challenging and requires meticulous execution to obtain a satisfactory outcome.
Proper use of impaction bone grafting affords good initial support for an implant that otherwise would be inadequately supported by native bone.3 In addition to a stable construct, the potential exists to restore femoral bone stock for future reconstructions if required. Impaction grafting offers a potential biologic alternative to many of the other methods of dealing with bone loss.
INDICATIONS AND CONTRAINDICATIONS
Femoral impaction bone grafting may be utilized in any patient undergoing revision of the femoral stem where restoration of femoral bone stock is considered advantageous, a scenario typically encountered in younger patients. This technique has been employed by some as a routine femoral revision technique, whereas others have limited it to specific revision scenarios. Impaction bone grafting is well suited in the case of a patulous femoral metaphysis or diaphysis that exceeds the diameter of available implants and precludes an adequate scratch fit for cementless fixation. When planning a cemented revision stem, impaction bone grafting is useful when a smooth endosteal canal is encountered, and little cancellous bone remains for mechanical interlocking fixation.
The presence of active infection is an absolute contraindication to impaction bone grafting. However, despite theoretical concerns about introducing bone graft in a previously infected host, clinical studies have demonstrated success when bone grafts are required for a subsequent reconstruction of a previously infected joint.4,5 English et al. reported on 53 patients who underwent impaction grafting of the femur during the second stage of a two-stage revision for infection and demonstrated 93% success rate in the elimination of infection at a mean follow-up of 53 months, results comparable to other techniques that do not use allograft bone.5
Relative contraindications include old age or extensive medical problems that would place the patient at jeopardy for complications with a long operation. Although meshes or cortical struts may be used to reconstruct the femoral tube, extensive or severe cortical defects and abnormally thin cortical bone may argue against this technique when other options are feasible. Diaphyseal bone fracture is a common serious complication of this procedure and speaks to the importance of proper patient selection.
BASIC SCIENCE
Bone Graft The original description of impaction grafting involved the use of morselized cancellous bone.6 Morselized cancellous bone was felt to be preferable to cortical bone Over-view given the more rapid revascularization, efficient osteogenesis, and early incorporation with host bone,7 and this remains the benchmark for modern impaction grafting techniques.3 However, despite slower rates of revascularization, morselized cortical grafts have theoretical advantages in providing increased initial structural support.8,9 Kligman et al. have reported decreased implant migration and improved clinical outcomes with morselized cortical graft in both femoral and acetabular reconstructions.10,11
The impacted graft may be either autograft or allograft. Autograft bone is osteogenic, osteoconductive, and osteoinductive, all of the characteristics required to stimulate new bone formation. Several experimental and clinical studies have confirmed its superiority to allograft, which is primarily osteoconductive and functions primarily as a scaffold for new bone formation.3,8 One of the earliest reports of a successful use of this technique involved the use of posterior iliac crest autograft.12 However, for practical reasons, allograft is used widely in clinical practice. The quantity of bone required to reconstruct large defects often exceeds the amount of autograft that is reasonably available. The risk of donor site morbidity is also avoided when using allograft. Femoral head allograft is the most common source of graft in our practice at the present time.
The processing of allograft may be another important factor in the outcome of impaction grafting. While fresh frozen bone is commonly used, preservation of allografts may be performed by freezing, freeze-drying, or irradiating the bone. Processing of the graft significantly reduces the immune response to the graft and speeds bony incorporation by eliminating bone marrow cells and fat.8,13,14 It also reduces the risk of disease transmission; cases have been reported of HIV and hepatitis C transmission with nonprocessed bone allograft.15,16 However, processed bone has decreased mechanical properties.17,18 Increased subsidence of the stem and lack of radiographic evidence of bone incorporation have been anecdotally reported in association with irradiated bone.19 Clinically, fresh frozen bone is currently associated with the best long-term results.20 However, given the variability in reported results, a recent Cochrane database review found no level 1 evidence upon which to draw conclusions about the clinical effectiveness of fresh frozen compared to processed bone.21
The densely packed bone graft must provide mechanical support for a femoral implant. The general consensus is that the particles should be as large as practical to ensure stability and cement penetration.22–24 The optimal size for graft particles for acetabular grafting has been reported to be between 7 and 10 mm, larger than the 2 to 5 mm graft particles obtained from most standard bone mills.25–27 The reverse reaming technique of a bone slurry graft has been shown to be inferior with mechanical testing.28 On the femoral side, the largest practical size for distal packing is thought to be between 3 and 5 mm,22 whereas the proximal quarter of the canal may be packed with larger 8 to 10 mm chips.29 Standard bone mills typically produce particles between 2 and 5 mm; a rongeur or bone nibbler produce a more optimal-size graft. A fine-milled bone slurry lacks the mechanical properties required for adequate impaction and should be avoided.
The optimal uniformity of particle size and the desired amount of compaction remains a matter of debate. In their original paper, Gie et al.30 recommended “vigorous impaction” for grafting of the femur, although this is difficult to quantify. While using a range of bone chip sizes and tightly packing the graft improves the initial stability, it also decreases graft permeability (by filling in the voids between large particles with smaller particles), potentially leading to reduced new bone ingrowth31,32 and less cement penetration.22,24
Preparation of the graft also influences the mechanical properties of the construct. Prewashing of the bone to remove fat and marrow fluid has been shown to allow the production of a stronger compacted graft that is more resistant to shear, presumably by decreasing the presence of incompressible fluid and interparticle lubricants.23,24,33 The biologic effects of removing potentially positive growth factors and negative immunogenic factors through rinsing are less clear.
Various materials have been used to augment the cancellous bone graft in an attempt to improve initial stability. Ceramic particles have been mixed in with the morselized bone with good short-term results.34 As a complete alternative to allograft, initial in vitro results of compacted porous titanium particles are also encouraging.35 In addition to structural augmentation, biological enhancement and modulation has also been explored with the addition of marrowderived autogenous progenitor cells,36 bone morphogenic proteins,32,37 and bisphosphonates37 with mixed results.38–40 At this time, the role of adjuvants in impaction grafting remains unclear.
Graft Incorporation and Regeneration The biologic fate of impacted bone grafts in total hip arthroplasty is largely unknown. The difficulty in interpreting the status of the bone graft on radiographs,41 combined with the lack of large numbers of autopsy retrievals, means that we have limited definitive scientific evidence on the long-term histologic status of these grafts.3
The long-term success of impaction grafting, and the ability to achieve the major benefit of restoration of bone stock, is dependent upon the biologic interplay between the host bone and the graft.22 Following bone grafting, an early phase of inflammation occurs, followed by revascularization.26,42 Positron emission tomography (PET) scans have suggested increased blood flow and bone formation adjacent to the allograft as early as 8 days postoperatively.43 Animal models have confirmed that graft resorption, woven bone deposition, and subsequent remodeling occurs over a period of several weeks, leading to almost complete incorporation at 12 weeks and full consolidation and revascularization by 24 weeks.44 The incorporation of impacted graft has been likened to the fracture healing process with endochondral ossification observed in the graft bed.44,45
Biopsies taken at the time of revision surgery and cadaveric specimens have given us some insight into the longer term fate of the impacted bone. Consistent evidence of living bone and osteoid formation in the graft has been reported at 4 months, although a significant proportion of the allograft appears to remain necrotic several years postoperatively.32,45 Biopsies from human specimens have suggested that the graft regenerates by 15 months,46 with complete incorporation and replacement with viable bone seen several years out from surgery.27,68
However, in a study of eight femoral biopsies taken at revision surgery and six femurs obtained at autopsy, Linder found a more inconsistent and variable spectrum of bone healing and regeneration.41 The most typical histologic pattern, seen in over half of specimens, was one of varied trabecular incorporation including fibrous tissue and necrotic bone. Variable amounts of graft bone were commonly seen to have been embedded into dense fibrous tissue, resulting in a composite tissue that could potentially bear load and support the prosthesis. The key biologic processes appeared to occur early given that the histologic appearance did not appear to differ or deteriorate after 6 months.41 There was poor correlation between the histologic findings and the radiographic images. Ullmark and Obrant reported a prospective series of 19 patients treated with impaction femoral grafting during revision surgery who subsequently had percutaneous biopsies taken from Gruen zones 1 and 2 at 1 to 48 months postoperatively.45 Fibrous tissue invasion of the graft and new bone formation occurred from the periphery of the graft and were complete by 11 months. The innermost layer of the graft bed consisted of dead trabecular graft with fibrous invasion but without the evidence of graft resorption. Although healing was more complete by 48 months, areas of necrotic graft persisted.
On the acetabular side, van der Donk et al.42 also found variable amounts of unincorporated graft in their examination of 24 biopsies of acetabular impaction bone grafts. Incorporation was seen in 30% of the graft by 6 months and 90% by 10 years. Areas of loose fibrous stroma on which new bone had formed were also noted.
Advanced imaging studies have also given some longer term evidence that bone regeneration within the graft reliably occurs. Ullmark et al. used PET scans to study five patients treated with femoral impaction grafting at 6 years postoperatively and found that the bone metabolism had normalized compared to native bone.47 On the whole, these studies suggest a reliable and durable biological outcome including bone regeneration with optimal application of this technique.
Mechanical loading of the graft appears to stimulate incorporation and has been cited as a principal cause for the success of this procedure.32,48,49 Although the effect of load on graft incorporation has been suggested, its exact influence on the fate of the graft is difficult to demonstrate. In a recent goat model utilizing a subcutaneous pressure implant, van der Donk et al.50 found that loading of impacted graft led to only a modest increase in the area of active incorporating bone and did not affect the formation of a new bony structure.
TECHNIQUE
The technique of impaction femoral grafting with cementing of the component was introduced into clinical practice in the late 1980s. Current techniques have been popularized by Gie et al.29,30 and Slooff et al.51 The surgical technique is both demanding and critically important in avoiding complications and ensuring long-term survival of the reconstruction.
Preoperative planning includes the exclusion of infection and the assessment of bone deficiencies. Templating is performed to estimate the length of stem required to bypass any cortical or significant endosteal defects. The common practice of bypassing cortical defects by at least two diaphyseal diameters is recommended.
The femur may be approached through an anterior, a posterior, or a transtrochanteric approach. The failed femoral component is removed along with cement and debris. Thorough debridement of the endosteal canal is important to ensure future incorporation of the impacted graft. A distal well-fixed cement column may be left in place as a distal plug of the column of bone graft. An extended trochanteric osteotomy may be used without compromising the results so long as the osteotomy is repaired adequately with cables.
The femur is assessed for bone deficiencies. Contained defects are a prerequisite to impaction grafting; uncontained defects may be converted to contained defects with the use of mesh. Malleable meshes may be secured with monofilament wire or cables. Allograft struts have also been employed to create a continuous femoral tube. Cerclage wires or cables should be employed prophylactically when the cortical bone is thin. Although the use of cortical onlay allograft struts may reduce the risk of fracture, care must be taken to avoid excessive soft tissue dissection and stripping, as this may compromise blood supply to the bone and impair subsequent revascularization.3 Occlusion of the canal is required 2 to 3 cm below the most distal cavitary deficiency or below the distal tip of the implant to be used.
In our practice, the cancellous bone graft is prepared in fragments measuring 4 to 6 mm in size. The authors routinely use allograft from fresh frozen femoral heads. Using a centering guide or guidewire to ensure a uniform bone mantle, progressively sized cylindrical packers are used, starting distally in the bottom of the canal, to compress the morselized bone.3 Once the canal is two-thirds full, tamps of the same shape as the prosthesis are used to shape the proximal femoral endosteal cavity and create an endosteal canal. At this time, careful attention must be paid to the version of the tamp (Fig. 27-1A,B,C). Vigorous impacting is required to provide rotational and axial stability for the stem, although excessive force may lead to fracture. The tamps are slightly oversized when compared to the actual implant to allow for a cement mantle. Trial reduction may be performed when the stability of the tamp is adequate.
FIGURE 27-1. Schematic representation of the technique of femoral impaction grafting. A. Retrograde cancellous bone packing of canal. B. Reshaping the femoral canal with temp. C. Attention to ramp version. (Adapted from Oakes DA, Cabanella ME. Impaction bone grafting for revision hip arthroplasty: biology and clinical applications. JAAOS 2006;14:624, Copyrighted and used with permission of Mayo Foundation for Medical Education and Research, all rights reserved.)
Following final preparation and drying of the neomedullary canal, cementation is performed in standard retrograde fashion. Pressurization is performed with the use of a flexible femoral seal. Maintaining pressurization of the cement, the implant is slowly inserted to the predetermined position and held as the cement polymerizes.
CLINICAL RESULTS
Intermediate-term results have shown impaction grafting to be a reliable and durable technique for femoral revision (Table 27.1). When done well, the results of impaction grafting are excellent with 10-year survivorship in excess of 90%.52 Analysis of 1,305 femoral revisions in Sweden revealed all-cause survivorship over 94% at 15 years using impaction grafting, demonstrating the predictable nature of the procedure (Figs. 27-2–27-4).60
TABLE 27.1 Clinical Results of Impaction Grafting of the Femur at Intermediate-Term Follow-up
aDepending upon prosthetic implant.
bKaplan Meier survival analysis not performed for aseptic loosening.
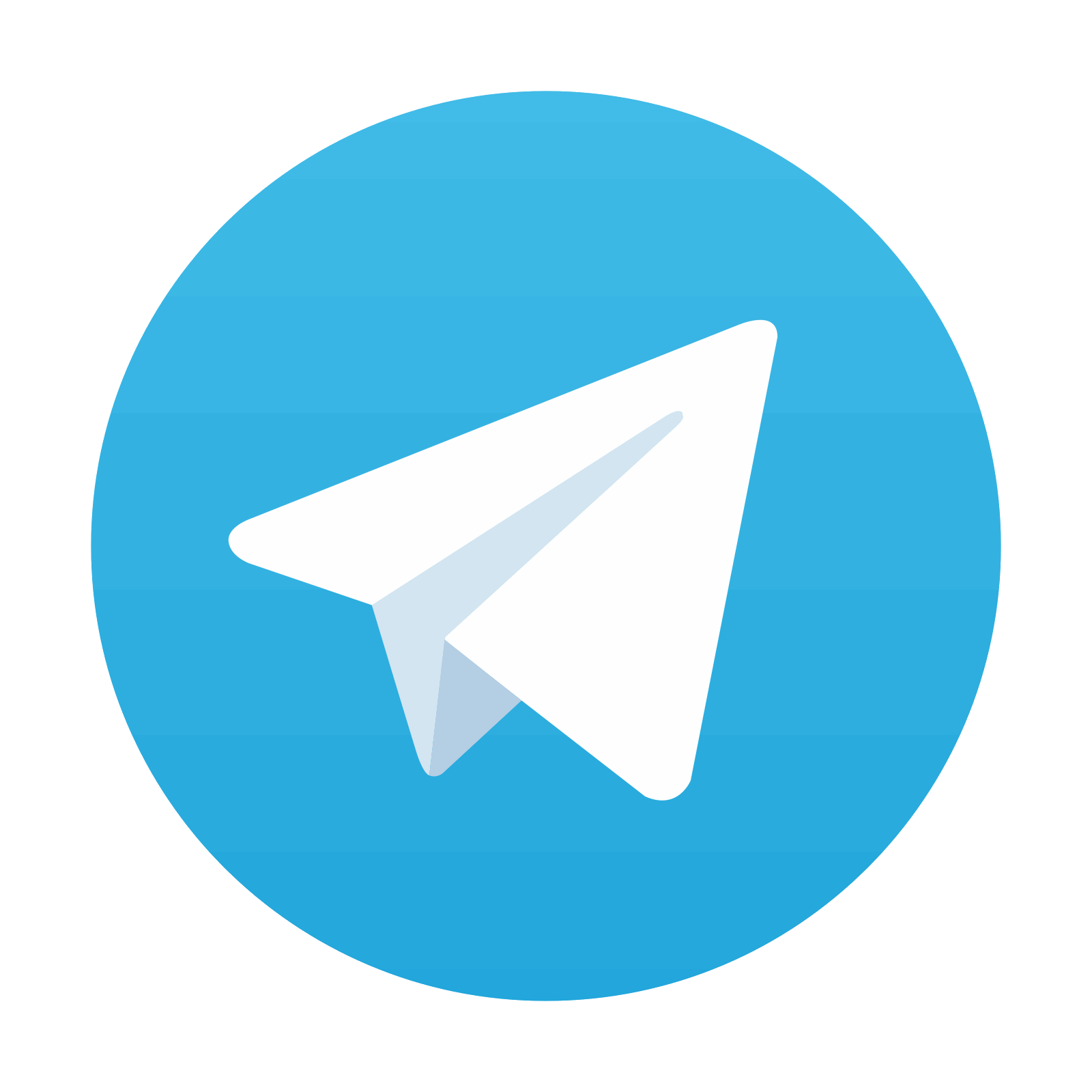
Stay updated, free articles. Join our Telegram channel

Full access? Get Clinical Tree
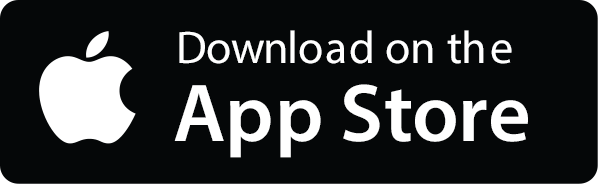
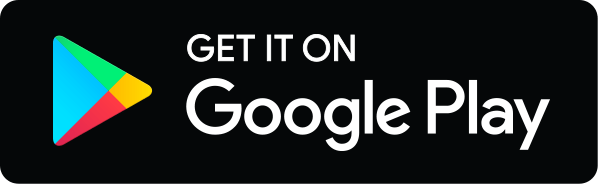