Head Injuries and Concussion
Andrew D. Pearle MD
Ronnie Barnes ATC
Russell F. Warren MD

Traumatic events can lead to both primary injuries [e.g., scalp lacerations, intracranial bleeds, skull fractures, concussion, and diffuse axonal injury, (DAI)] and later, secondary injuries (e.g., herniation syndromes, diffuse edema, and hypoxic/ischemic injuries).
Initial evaluation and treatment should progress through the ABCs (airway, breathing, and circulation) of trauma resuscitation. Glasgow Coma Scale is a standard measure of level of consciousness.
Orientation (time, place, and person), retrograde amnesia, dizziness, visual changes, nausea, tinnitus, pupils, and head/neck pain should also be assessed.
Physical examination should include detailed head and neck assessment for crepitus/deformity, bruising (e.g., the Battle sign), blood or fluid leakage [e.g., cerebrospinal fluid (CSF) leak], and tenderness. A careful neurologic examination, including cranial nerve assessment, is important; mini-mental status examinations can be helpful in the evaluation of neurocognitive function.
Intracranial hemorrhage can be divided into epidural, subdural, and subarachnoid.
The most common definition of concussion is a “clinical syndrome characterized by immediate and transient posttraumatic impairment of neural functions, such as alteration of consciousness, disturbance of vision, equilibrium, etc. due to brain stem involvement.” A concussion is sometimes called mild traumatic brain injury (MTBI).
Any head trauma associated with a loss of consciousness is a concussion. Headache (46%), dizziness (42%), and blurred vision (16%) are the most common symptoms.
It is estimated that 20% of patients with head trauma will suffer postconcussive symptoms (impaired memory and concentration, persistent headache, fatigue, mood and sleep disturbances, and dizziness).
Second-impact syndrome is a rare, potentially life-threatening event that results when a second impact occurs before complete recovery from the initial concussion. It can occur with a relatively minor impact. Clinically, the athlete appears to be stunned, then collapses within minutes. Pupils become dilated, and patient is semicomatose and develops respiratory distress.
Dementia puglistica (ataxia, pyramidal tract dysfunction, tremor, impaired memory, dysarthria, and behavioral changes) is a chronic brain injury that results from multiple concussions. Chronic traumatic brain injury (CTBI) is the term that has been used more recently. It includes decreased processing speed, short-term memory impairment, concentration deficit, irritability or depression, fatigue and sleep disturbance, a general feeling of fogginess, and academic difficulties.
The management of concussions has three crucial components: identifying neurologic emergencies, preventing second-impact syndrome, and avoiding the effects of repetitive concussions.
Once an athlete is asymptomatic, progression of activity is instituted over several days and is followed with continued monitoring. Each step takes a minimum of 1 day. The recommended steps from the 2001 Vienna Conference on Concussions in Sport are (a) no activity, rest until asymptomatic; (b) light aerobic activity; (c) sport-specific training; (d) noncontact training drills; (e) full-contact training; and (f) game play.
Head injuries are a major concern to the team physicians, and these injuries represent a wide spectrum of trauma. Concussion remains the most common type of sports-related head injury and is the focus of this chapter. However, more severe intracranial lesions, such as intracranial hemorrhage, cerebral contusion, and related complications, must be considered with any head injury.
Primary injuries arise from an initial traumatic event and include scalp and skull injuries, extraparenchymal hemorrhage, cortical contusion, concussion, and diffuse axonal injury. As with any trauma, patients are at risk of subsequent secondary injury, which in the setting of head injury can be particularly devastating because of the limited and fixed cranial space and the inability of cerebral tissue to repair or to regenerate. Secondary injury includes herniation syndromes, secondary infarcts and bleeds, diffuse cerebral edema, hypoxic and ischemic injuries, and hydrocephalus. The fundamental goals in treating head injury are to recognize primary injuries and to prevent secondary lesions.

Athletic head injuries usually result from direct impact and/or deceleration-rotational events. Classic principles of brain contusions include the concept of coup injury, in which a forceful blow to a stationary head imparts maximal brain injury directly beneath the point of impact. On the other hand, contrecoup injuries occur when the energy of impact is transferred to the mobile brain, such that it glides against the fixed, sharp surfaces of the dural reflection or skull base ridges, thus resulting in a contusion at a site opposite that of the initial external trauma. The effect of forces on the brain parenchyma normally are buffered by the cerebrospinal fluid (CSF), which serves as a shock absorber, protecting the brain by converting focal stresses to more uniformly distributed compressive stress. The contrecoup injury pattern occurs because, in the moving head, the floating brain lags behind the leading skull edge, diminishing the protective CSF in the trailing surface and creating a thickened layer of CSF at the leading cranial surface. In the setting of skull fractures, the depressed fragment may directly injure the adjacent parenchyma.
Three types of stresses commonly are imparted to brain tissue—compression, tensile, and shear—and these types may occur in combination. Compressive forces result in direct impact to the brain parenchyma, as in the case of depressed skull fractures. Tensile and compressive forces may occur with linear acceleration injuries, such as in the coup/contrecoup injury patterns. Shearing injuries are often caused by rotational forces and are poorly tolerated by brain parenchyma. These injuries are thought to be related to differences in the physical properties of gray matter and axonal fibers (density differences), such that they decelerate at different velocities; thus, shearing injuries typically occur at gray matter–white matter junctions (1).

On-the-Field Evaluation
The initial on-the-field evaluation is directed toward assessing the levels of consciousness and of associated injuries, particularly cervical spine trauma. The medical personnel must first assess the patient’s airway, breathing, and circulation (i.e., ABCs). If the patient is unconscious, the initial respondent must assume that an associated cervical spine injury has occurred, and the neck should be stabilized immediately. A rapid assessment must be made concerning the need for possible emergent transport to a hospital setting.
The standard method for assessing the level of consciousness is the Glasgow Coma Scale (Table 9-1). In addition to observing the patient’s motor, eye opening, and verbal responses, medical personnel should assess for orientation to person, place, and time and for the presence of retrograde amnesia.
Symptoms such as dizziness, visual changes, and head or neck pain must be ascertained before removing the athlete
from the field. If the athlete is able to understand and follow commands, he or she may be assisted to a seated position. If the initial respondent is confident that the athlete has sufficient strength and coordination, the patient can be helped to his or her feet and assisted off the field. Medical personnel should consider the use of a motorized cart or stretcher if the athlete cannot maintain balance in the seated or standing position.
from the field. If the athlete is able to understand and follow commands, he or she may be assisted to a seated position. If the initial respondent is confident that the athlete has sufficient strength and coordination, the patient can be helped to his or her feet and assisted off the field. Medical personnel should consider the use of a motorized cart or stretcher if the athlete cannot maintain balance in the seated or standing position.
Table 9-1 Glasgow Coma Scale | ||||||||||||||||||||||||||||||||||||||||
---|---|---|---|---|---|---|---|---|---|---|---|---|---|---|---|---|---|---|---|---|---|---|---|---|---|---|---|---|---|---|---|---|---|---|---|---|---|---|---|---|
|
On-the-Bench Evaluation
Athletes should be questioned for specific symptoms when they are taken off the field. In particular, athletes should be questioned about dizziness, visual changes (e.g., photophobia, double vision, or blurriness), headache, nausea, vertigo, and tinnitus. Many of these symptoms may not be present immediately but may occur in a delayed fashion. Vomiting may be indicative of a significant head injury with elevated intracranial pressure.
Physical examination should be directed at careful inspection and palpation of the head and neck. Facial bones should be evaluated carefully for crepitus and fracture. Mandibular fractures can be detected based on pain or malorientation with teeth clenching and grinding. Nasal fractures can be determined by palpation or visualization of deformity.
Certain findings are indicative of specific fracture patterns. Leakage of cerebral spinal fluid from the nose is suggestive of a cribiform plate fracture of the skull. Spinal fluid adjacent to the tympanic membrane is suggestive of a fracture in the temporal bone. The Battle sign (i.e., ecchymosis posterior to the ear in the mastoid region) is indicative of a posterior skull fracture.
A careful cranial nerve examination should be performed. Salient features of this examination include changes in pupil size, which can suggest increased intracranial pressures or a unilateral sympathetic nerve response; deficient oculomotor function caused by injury to the third cranial nerve from a skull fracture or subdural hematoma; asymmetric upward case because of infraorbital blow-out fractures resulting in entrapment of the inferior rectus muscle; and cranial nerve VII palsy from basilar skull fractures. In addition, transient nystagmus often is seen after shearing or rotatory injury to the brainstem. A complete neurologic examination, including assessment for strength, sensation, and coordination, should be performed.
A neurocognitive assessment should include the athlete’s orientation to person, place, and time; recent memory, new learning, and delayed recall can be tested using short word recalls. Concentration can be evaluated by having the patient say the months of the year in reverse or by subtracting with serial sevens. The Mini-Mental Status Exam is a formalized, brief screening tool that discriminates patients with moderate or severe deficits (2). Other structured sideline assessments of neurocognitive dysfunction may be employed to standardize evaluation after head trauma (Appendix 1) (3,4,5).

Intracranial hemorrhage can be divided into three types: epidural, subdural, and subarachnoid (Fig 9-1). Each type of intracranial hemorrhage is potentially devastating, so prompt, accurate assessment and appropriate treatment must be instituted. The initial presentation ranges from headache to neurologic deficits to loss of consciousness. Blood is an irritant to brain tissue and may precipitate a seizure.
Arterial epidural hematomas often are associated with temporal bone fractures and may rapidly enlarge. The bleeds frequently are the result of middle meningeal arterial injuries. Blood rapidly accumulates between the skull and dura, and the amount of blood may reach a fatal accumulation in 30 to 60 minutes. Classically, patients are described as having a lucid interval after an initial loss of consciousness, but this only occurs in approximately one third of patients (6). Early diagnosis and rapid evacuation of large or expanding hematomas are essential in many cases. A good outcome can occur with appropriate craniotomy and evacuation, whereas an unrecognized bleed may be fatal.
Subdural hematomas occur between the dura and arachnoid and often are the result of torn bridging veins. Subdural hematomas are a leading cause of death in athletes, and they are approximately threefold more common than epidural hematomas (7). Unlike epidural hematomas, subdural hematomas commonly are associated with insult to brain tissue that can make recovery guarded. Acute subdural hematomas can lead to herniation-induced brainstem dysfunction with a high mortality rate, even with neurosurgical intervention (8). Subdural hematomas can present with rapid-onset, focal neurologic signs, including hemiparesis, aphasia, and a “blown pupil.” Chronic subdural hematomas, on the other hand, may present in a delayed
fashion, with persistent symptoms of headache and subtle changes in mental, motor, or sensory function.
fashion, with persistent symptoms of headache and subtle changes in mental, motor, or sensory function.
Finally, subarachnoid hematomas are hemorrhages confined to the CSF space along the surface of the brain, usually as a result of rupture of the small, surface brain vessels. Nuchal rigidity may be present because of the meningeal irritation. Subarachnoid hemorrhage, even if posttraumatic, can irritate the meninges and cause vascular spasm, leading to infarcts.
Cerebral contusion is a “bruise” of the brain parenchyma. These lesions may occur in the setting of a depressed skull fracture or when acceleration–deceleration forces cause the brain to impact with the inner table of the skull. Cerebral contusions most commonly are supratentorial and are associated with concussion. They may progress to focal neurologic deficits over the first 24 to 48 hours; close monitoring with serial computed tomographic (CT) evaluation is essential.
Diffuse axonal injuries occur with high-velocity rotational forces. In this injury, multifocal microhemorrhages and edema may occur that can be detected with CT scanning, although magnetic resonance imaging (MRI) is more sensitive. Common locations for the hemorrhages include the gray matter–white matter junctions, deep gray matter, upper brainstem, corpus callosum, and internal capsule.

Overview
Now commonly referred to as mild traumatic brain injury (MTBI), concussion is clearly the most common athletic head injury, but agreement on its definition remains elusive. The most commonly cited definition, proposed by the Committee on Head Injury Nomenclature of the Congress of Neurologic Surgeons, is a “clinical syndrome characterized by immediate and transient posttraumatic impairment of neural functions, such as alteration of consciousness, disturbance of vision, equilibrium, etc. due to brain stem involvement” (9). The cardinal features of concussion are an alteration of mental status after head injury, a Glasgow Coma Scale ranging from 13 to 15, and negative findings on neuroimaging; these features may or may not be accompanied by a loss of consciousness (10).
Although most athletes fully recover from a concussion, an unknown percentage of patients will have persistent sequelae that can be disabling and, in some cases, permanent. Most physicians are aware of the dangers of second-impact syndrome, which is the catastrophic scenario of a second head trauma in a patient who remains symptomatic from a previous concussion (11); however, more subtle chronic sequelae of repetitive concussive episodes, such as impairment of cognitive processes, mood, and behavior as well as increased risk of subsequent MTBIs, are less understood (12,13,14,15). At present, we have no treatment for acute concussions or the chronic sequelae, no established means to predict chronicity, and no definitive guidelines for return to play. However, early diagnosis of concussion and postconcussive symptoms and utilization of preventative strategies are likely to result in better treatment of athletes with head injuries.
Cerebral Pathophysiology of Concussion
Concussion injuries are thought to result from diffuse axonal injuries caused by rapid acceleration/deceleration of the head, which results in stretching of nerve fibers (16). During the past decade, significant insight has been gained regarding the pathophysiology of concussion. The postconcussive defects clearly do not occur with significant anatomic perturbation and do appear to resolve over time. This has led to the concept that MTBI results in temporary neuronal dysfunction rather than cell death. However, whereas brain cells are not irreversibly destroyed by MTBI, neural cells remain in a vulnerable state before recovery. The neurometabolic events after experimental concussive brain injury have been elucidated, yielding insight concerning the etiology of postconcussive symptomatology and vulnerability (17).
Experimental animal models have demonstrated metabolic dysfunction to be the key physiological event after a concussive event. Disorganized release of neurotransmitters results in poorly regulated neuronal depolarizations and abnormal ionic shifts. The altered cellular physiology causes a hypermetabolism of glucose and a “cellular energy crisis” that is exacerbated by a decrease in cerebral blow flow (18). This cellular energy crisis is thought to cause the increased vulnerability to a second concussive event (19). After the initial period of hypermetabolic glucose consumption, the concussed brain is thought to enter a period of depressed metabolism that may impair posttraumatic neural connectivity, leading to lasting changes in cognitive potential (17). Cerebral metabolic function can be adversely affected for days in animals, but the time course appears to be significantly longer in humans (17). As the clinical significance and duration of the neurometabolic events after MTBI are defined further, more appropriate guidelines for return to play will be established.
Epidemiology
Overall, it is estimated that approximately 300,000 sports-related concussive events occur in the United States annually (20). It has been estimated that 3.9% to 7.7% of high school and college athletes sustain a concussion each year (21). Contact sports in particular place athletes at risk for head trauma. Football is recognized as having the highest risk; however, basketball, softball, soccer, baseball, rugby, and ice hockey also have a moderate to high risk of concussion [9].
The incidence of concussion among high school and collegiate football players is approximately 3% to 20%. In a recent study using comprehensive concussion assessment to
investigate the incidence of concussion among 17,500 football players, Guskiewicz et al. (9) found that 5.1% of athletes sustained a concussion in a single season; the greatest incidence of concussion was found at the high school level, followed by the division III college level. Recent data from the National Football League collected between 1996 and 2001 demonstrated a concussion rate of 0.41 concussions per NFL game (22).
investigate the incidence of concussion among 17,500 football players, Guskiewicz et al. (9) found that 5.1% of athletes sustained a concussion in a single season; the greatest incidence of concussion was found at the high school level, followed by the division III college level. Recent data from the National Football League collected between 1996 and 2001 demonstrated a concussion rate of 0.41 concussions per NFL game (22).
Recent studies have highlighted an increased predisposition to future concussive events after an initial MTBI. Guskiewicz et al. (23) found a threefold increase in concussive risk if a player had suffered three or more MTBIs during a period of 7 years. In a separate study, the incidence of a recurrent concussion within a single football season was 14.7% (9). Recurrent concussive events also may be associated with slower recovery severe and more severe symptoms. Players with two or more previous concussions require a longer time for symptom resolution (23), and athletes with three or more previous concussions have an eightfold increased risk of experiencing loss of consciousness after MTBI (14). The increased risk of repeat MTBI is thought to result from postconcussive neurometabolic changes that produced increased cerebral vulnerability to recurrent injury.
Mechanism of Injury
A committee of representatives from the NFL Team Physicians Society and the NFL Athletic Trainers Society, NFL equipment managers, and scientific experts in the area of traumatic brain injury recently investigated the location and direction of helmet impacts in the NFL using sophisticated acquisition of high-speed video impact data and biomechanical reconstruction to determine impact velocity, change in head velocity, and acceleration forces (22,24,25). Data from this study group have demonstrated the striking observation that concussed players in the NFL experienced average head impacts of approximately 21 mph. The change in head velocity of injured players averaged 16 mph, which was significantly higher than the change in head velocity for the uninjured players involved in the collision (average, 9 mph) (25). The majority of concussions occurred by impact with another player’s helmet (24). Interestingly, concussion occurred with the lowest head acceleration if a player was struck on the side of the face mask as compared with impacts to other areas of the helmet. Those authors suggest that impacts to the face mask twist the head in addition to accelerating it, and this combination of rotation coupled with translation may influence concussion tolerance (25). These data provide initial information regarding the biomechanics of athletic concussion in football and begin to provide insight regarding the forces that are required to cause MTBI.
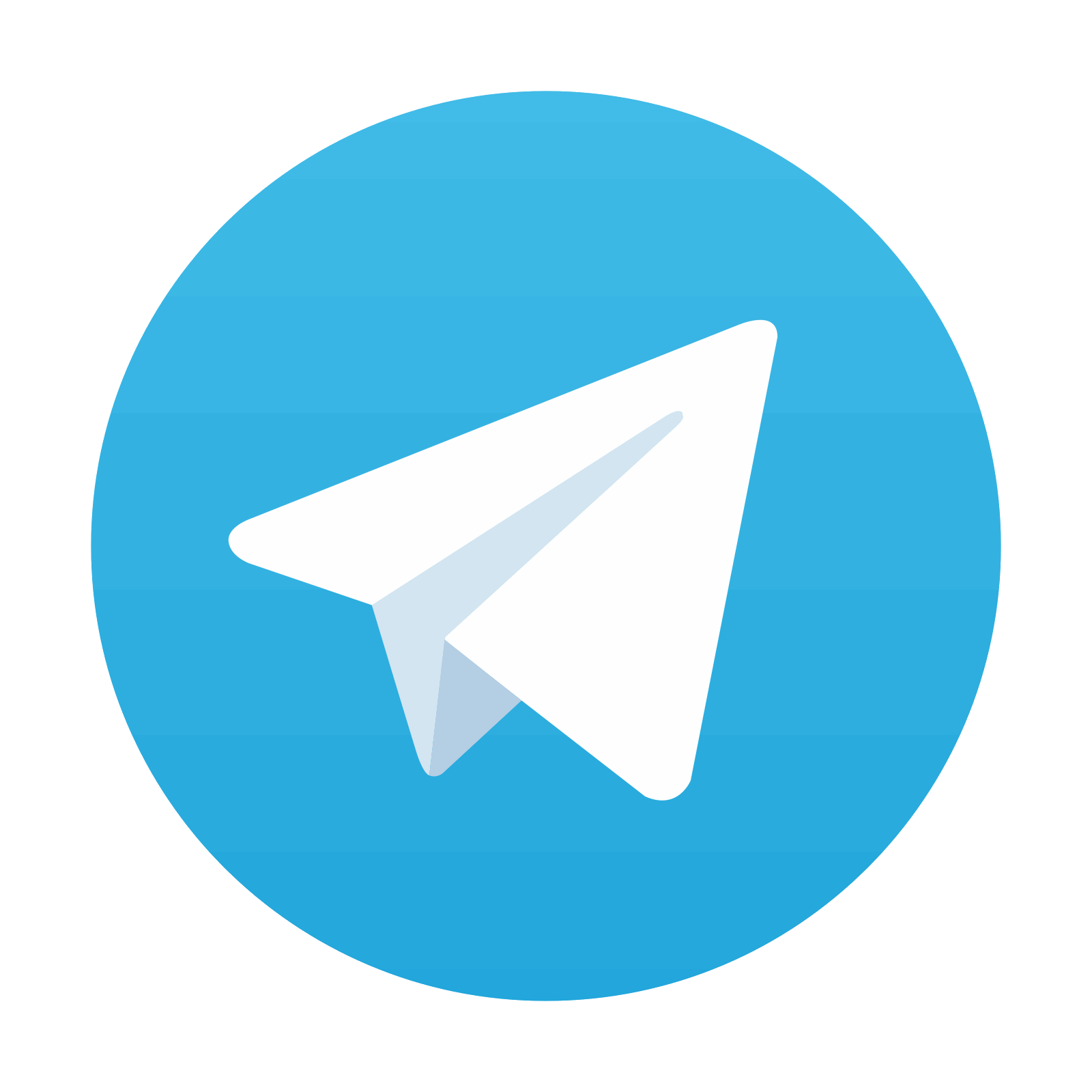
Stay updated, free articles. Join our Telegram channel

Full access? Get Clinical Tree
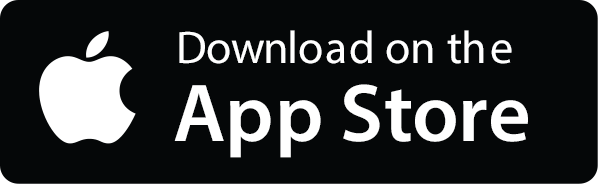
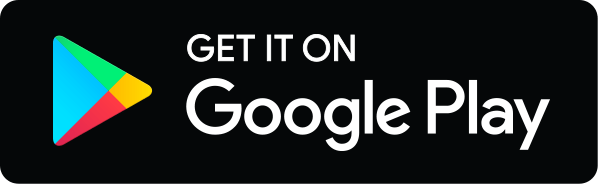