Chapter 18 Genes and Genetics of Murine Lupus
INTRODUCTION
Systemic lupus erythematosus (SLE) is a disorder of systemic autoimmunity characterized by the formation of a variety of autoantibodies and subsequent development of immune complex (IC) glomerulonephritis, that is, lupus nephritis.1 The pathogenesis of SLE is a complex process in which major histocompatibility complex (MHC)—linked and multiple non—MHC-linked genetic factors contribute to the overall susceptibility and progression of the disease, along with contributions of hormonal and environmental factors. The involvement of genetic factors in SLE was initially suspected because of a familial tendency for SLE, leading to the concept of a special genetic background necessary for contracting SLE. However, in monozygotic twins the concordance rate was only between 30 to 50%, thus indicating that both genetic and environmental factors are critically involved in the pathogenesis of SLE. Because of the complex nature of the disease, a sophisticated genetic analysis of SLE is only possible by using animal models with well-defined backgrounds. Thus, the availability of several murine strains with distinct genetic backgrounds, such as (NZB × NZW)F1, MRL, and BXSB, which all spontaneously develop an autoimmune syndrome resembling human SLE, has offered an invaluable opportunity for elucidating the genetic basis underlying the etiopathogenesis of SLE. Indeed, genome-wide linkage analyses involving lupus-prone and nonautoimmune strains have helped identify multiple lupus susceptibility loci. Current efforts are focused on evaluation of candidate genes located within these susceptibility loci by analyzing autoimmune phenotypes in mice congenic for different susceptibility intervals.
MURINE MODELS OF SLE
Mice of the (NZB × NZW)F1 hybrid strain, and MRL and BXSB strains have been extensively used as experimental models of human SLE.2 They are characterized by a wide spectrum of autoimmune manifestations culminating in the development of IC-mediated lupus nephritis. The severity of kidney lesions is closely associated with the increase in serum titers of IgG autoantibodies directed against various nuclear antigens, such as DNA and chromatin, and of circulating retroviral gp70-anti-gp70 IC (gp70 IC).
The NZB (H2d) and NZW (H2z) strains were developed in New Zealand from a murine stock of undefined background by selection on black and white color, respectively. NZB mice develop autoimmune hemolytic anemia, but neither NZB nor NZW mice develop a typical lupus-like syndrome. In contrast, (NZB × NZW)F1 hybrid mice develop a severe autoimmune disease resembling human SLE, which affects the females earlier than the males, and sex hormones have been shown to be responsible for the early development of disease in the females. These F1 mice have been used as the classical animal model of SLE. The MRL strain (H2k) is derived from a series of crosses involving four strains (LG/J, AKR/J, C3H/Di, and C57BL/6). The spontaneous and recessive lpr (lymphoproliferation) mutation in the MRL strain results in a generalized lymphadenopathy due to massive accumulation of a unique subset of T cells (TCRαβ+, CD4−, CD8−, B220+).3 The lpr mutation consists of an insertion of an endogenous retrovirus in the Fas gene that codes for a receptor implicated in apoptosis of lymphocytes.4 The presence of the Faslpr mutation markedly accelerates the progression of SLE-like autoimmune syndrome in MRL mice. Besides the SLE-like autoimmune syndrome, MRL-Faslpr mice produce high titers of IgM and IgG rheumatoid factors and develop arthritis-like joint lesions resembling human rheumatoid arthritis.5 The BXSB mouse (H2b) is a recombinant inbred strain derived from a cross between a C57BL/6 (B6) female and an SB/Le male. These mice spontaneously develop an SLE-like disease that affects male animals much earlier than females. The male-determined accelerated disease is independent of sex hormones, but due to the presence of an as yet unidentified mutant gene, designated Yaa (Y-linked autoimmune acceleration), present in the Y chromosome of BXSB mice, which is originally inherited from the SB/Le strain.6
MULTIGENIC FEATURES OF MURINE SLE
Since the development of an SLE-like syndrome was first reported in the F1 progeny of the NZB and NZW strains, the genetic basis for SLE in (NZB × NZW)F1 hybrids has been investigated in a number of laboratories. Since the major features of (NZB × NZW)F1 autoimmune disease are not present in the parental strains, it is clear that genes from each parent act in concert to produce the F1 phenotype. Early genetic studies on New Zealand mice have demonstrated that many individual autoimmune traits segregate independently of each other in (NZB × NZW)F1 × NZW back-cross mice,7 in (NZB × NZW)F2 mice8 and in recombinant inbred strains derived from the NZB strain through crosses with non-autoimmune strains of mice.9 This suggests that there is no common genetic defect causing overall autoimmune responses, but rather that each of the autoimmune traits is under the control of a separate genetic mechanism.
Classic progeny studies have provided only limited information on the number, identity and chromosomal location of the lupus susceptibility genes. However, the availability of polymorphic microsatellite markers covering the entire mouse genome has permitted to map more precisely the genetic loci linked with a wide spectrum of autoimmune traits, that is, production of autoantibodies, development of lupus nephritis and production of nephritogenic gp70 autoantigen. Results from genome-wide linkage analyses in mice obtained through by intercrosses or back-crosses of different lupus-prone and nonautoimmune strains led to the identification of multiple autoimmune susceptibility regions distributed all over the murine genome.10–12 These analyses have shown that (1) lupus-like disease is controlled by sets of susceptibility loci that independently or additively contribute to the overall susceptibility and progression of the disease, (2) heterogeneous combinations of multiple disease-promoting genes operate in a threshold-dependent manner to achieve full expression of the disease, and (3) contributions are unlikely to be linked to “true” genetic mutations, but are rather due to polymorphic alleles with subtle functional differences, except for the Fas and Yaa mutations observed in MRL and BXSB mice, respectively.
SPONTANEOUS MUTATIONS PREDISPOSING TO SLE IN LUPUS MICE
Fas and Fas Ligand Gene
The identification of defects in Fas mapped to chromosome 19, which is involved in apoptosis, in lupus-prone MRL mice with the lpr phenotype, represented an important contribution to our understanding of the genetic basis of SLE.4 This mutation produces a massive enlargement of lymph nodes with the accumulation of a particular subset of T cells that are phenotypically TCRαβ+, CD4−, CD8−, but express the B220 molecule characteristic of B cells.3 Notably, the gld (generalized lymphoproliferative disease) mutation, discovered in a colony of the C3H/HeJ strain, induces marked lymphadenopathy phenotypically indistinguishable from that induced by the lpr mutation.13 As a matter of fact, gld was identified as a mutation of the gene encoding the Fas ligand (FasL), present in chromosome 1.14 These Faslpr and Faslgld mutations not only accelerate the progression of autoimmune disease in lupus-prone MRL mice, but also induce the production of a broad spectrum of autoantibodies in various strains of mice, including those not predisposed to SLE.2,15,16 Fas is highly expressed in activated B and T cells, while the expression of FasL is limited to activated T cells.3 However, the Fas apoptosis pathway does not appear to be essential for negative selection during T and B cell development in thymus and bone marrow, respectively.17,18 Therefore, it has been speculated that the abnormal regulation of the Fas apoptotic pathway could result in prevention of antigen-induced apoptotic death of autoreactive lymphocytes in the periphery, thereby promoting the development of lupus-like autoimmune responses. However, it should be stressed that the mutation of Fas or FasL alone is not sufficient to induce severe autoimmune disease in mice that are not predisposed to SLE,13,15,16 underlining the importance of other still undefined lupus susceptibility background genes in the development of full-blown SLE.
Although Fas mutations have been identified in children with a rare autoimmune lymphoproliferative syndrome (ALPS),19,20 this was not really the case in SLE patients.21 Nevertheless, studies in mice bearing the lpr or gld mutation clearly indicate the importance of the genes regulating apoptosis in the development of SLE. It can be speculated that one of the defects in lupus-prone mice may be the failure to efficiently eliminate autoreactive B cells upon interaction with autoantigens. In fact, the resistance of mature B cells to B-cell receptor (BCR)-mediated apoptosis has been reported in lupus-prone (NZB × NZW)F1 mice.22 This concept is consistent with the findings that transgenic overexpression of the antiapoptotic proto-oncogene bcl-2 in B cells led to spontaneous development of an SLE-like autoimmune syndrome in certain strains of mice,23 and that the constitutive expression of the bcl-2 gene is able to counteract the apoptotic death of autoreactive B cells upon interaction with autoantigens in the periphery.24
Yaa Mutation
In contrast to the accelerated development of SLE in (NZB × NZW)F1 female mice, male BXSB mice develop disease much more rapidly than their female counterparts.2 This striking sexual dimorphism is not hormonally mediated, but results from a mutant gene, Yaa, present in the Y chromosome of the BXSB strain.25–27 The contribution of the Yaa mutation to lupus susceptibility remains limited without other background genes, since nonautoimmune strains, such as CBA/J and B6, were largely unaffected by the Yaa mutation. Notably, when B6.Yaa consomic males are mated with NZW females, which are phenotypically normal but have a genetic potential to develop SLE, F1 hybrid males bearing the Yaa mutation develop typical SLE.26 In addition, studies in B6 or C57BL/10 (B10) mice carrying different lupus susceptibility loci derived from either NZB, NZW or BXSB mice have shown that the combination of a single lupus susceptibility locus with Yaa can be sufficient to induce the development of lupus-like autoimmune syndrome, although the severity of the disease was variable, depending on the individual lupus susceptibility locus studied.28–30 These results indicate that the Yaa mutation by itself is unable to promote SLE in mice which are not predisposed to autoimmune diseases, but in combination with autosomal susceptibility alleles present in different lupus-prone strains, it can induce or accelerate the development of SLE.
It is clear that the molecular characterization of Yaa would give valuable information on the general mechanisms implicated in the development of SLE. Unfortunately, it is impossible to map this locus by conventional genetics because of the lack of homologous recombination of the Y chromosome. In an attempt to identify the cell types which express the Yaa mutation, we have produced Yaa plus non-Yaa double-bone-marrow chimeric mice, and analyzed the origin of autoantibodies produced in these mice. This analysis revealed that only B cells of Yaa origin participated in the production of IgG anti-DNA autoantibodies, and that they maintained this production even after selective depletion of T cells of Yaa origin.31,32 Thus, the Yaa abnormality is functionally expressed in B cells, but not in T cells. Based on this finding, it has been hypothesized that the action of Yaa may be to decrease the threshold for BCR-dependent stimulation, thereby promoting the activation of autoreactive B cells.33 This hypothesis is in agreement with the selective autoimmune enhancing effect of the Yaa mutation: the Yaa effect appears to be essential for the promotion of autoimmune responses in mice having only a limited activity of T-helper cells specific for a given autoantigen.34 It has been reported that lupus-like autoimmunity could be detected in genetically engineered mice lacking or overexpressing molecules implicated in the regulation of BCR signaling, which indicates that the deregulation of BCR signaling may be a critical element in the triggering and activation of potentially autoreactive B cells. According to this model, the Yaa mutation would have a direct enhancing effect on BCR signaling and thereby trigger the cascade of events initiating SLE.
A unique cellular abnormality associated with the Yaa mutation is monocytosis.35 At 8 months of age, monocytes reached a frequency of more than 50% of peripheral blood mononuclear cells in BXSB Yaa male mice. The development of monocytosis was closely associated with the progression of SLE, since monocytosis was observed in (NZB × B6.Yaa)F1 male mice developing SLE, but not in B6.Yaa males, which fail to develop a lupus-like autoimmune syndrome.36 Furthermore, recent analysis of B6 × (NZB × B6.Yaa)F1 back-cross males bearing the Yaa mutation revealed a remarkable correlation of monocytosis with autoantibody production and subsequent development of lupus nephritis,37 indicating that monocytosis is a useful and predictive marker for severe SLE. Significantly, Yaa-mediated monocytosis resulted in selective expansion of a monocyte subset expressing the CD11c dendritic cell marker,36 which is therefore considered to be a potential source of tissue-resident dendritic cells. Thus, the association of monocytosis with the development of SLE could be explained by a possible expansion of dendritic cells, which would further promote the production of pathogenic autoantibodies. In addition, given the considerable role of infiltrating macrophages in the progression of glomerular lesions, and given the implication of stimulatory IgG Fc receptor (FcγR) in murine lupus nephritis,38 monocytosis could promote glomerular inflammation and injury through increased secretion of proinflammatory cytokines, reactive oxygen species, and matrix-specific proteases, possibly as a result of IC-mediated, FcγR-dependent activation of infiltrating macrophages. Although the precise cellular and molecular basis of the Yaa gene-linked development of monocytosis has not been defined, the analysis of Yaa plus non-Yaa mixed-bone-marrow chimeras showed no overrepresentation of monocytes of Yaa origin over those of non-Yaa origin, indicating that the development of monocytosis is not due to an intrinsic abnormality in the growth potential of monocyte lineage cells from Yaa mice.36 Rather, Yaa-mediated monocytosis may result from an excessive production of monocyte-specific growth factor(s)—for example, by activated macrophages—possibly due to hyper-responsiveness of FcγR to autoimmune IC that arise during the course of the disease.
MHC ASSOCIATION OF MURINE SLE
Association of H2d/z Heterozygosity with Murine SLE
Extensive studies in New Zealand mice have demonstrated a strong association of H2d/z heterozygosity (vs. H2d/d or H2z/z) with the development of SLE, indicating a co-dominant contribution from each strain, i.e., H2d from NZB and H2z from NZW.39,40 However, it is still unknown how this H2 heterozygosity mechanistically contributes to murine SLE. It has been proposed that mixed haplotype class-II molecules produced by heterozygous pairing of an α-chain from one haplotype with a β-chain from the other haplotype might play a critical role in the development of SLE. However, the lack of disease enhancement by an Abz transgene introduced into H2d homozygous (NZB × NZW.H2d)F1 mice41 and by Ez or Az transgene in (B6 × NZB)F1 × NZB back-cross mice42,43 argue against this possibility. Significantly, a comparative serologic analysis of two different nephritogenic anti-DNA and anti-gp70 autoantibody productions in (NZB × NZW)F1 × NZW and (NZB × NZW)F1 × NZB back-cross mice revealed that in the F1 × NZW back-cross, H2d/z (compared with H2z/z) was associated preferentially with the production of anti-gp70 rather than anti-DNA autoantibodies, whereas the opposite influence was noted for H2d/z (compared with H2d/d) in the F1 × NZB back-cross.44 These results suggest that enhancement of disease by H2d/z heterozygosity is related to separate contributions from H2d and H2z, thus providing one explanation as to why H2d/z heterozygosity is required for full expression of disease in (NZB × NZW)F1 mice.
Association of H2b with Murine SLE
Another contribution of the MHC to the regulation of murine SLE, which is different from that seen in (NZB × NZW)F1 hybrid mice, has been well documented in BXSB and (NZB × BXSB)F1 mice, in which lupus susceptibility was more closely linked with the H2b haplotype than with the H2d and H2k haplotypes.45–47 However, this MHC effect was limited, as it was markedly influenced by other factors in the genetic background of individual lupus-prone mice. In the context of the BXSB background, in which the development of SLE is dependent on the Yaa mutation, the H2d or H2k haplotype almost completely prevented the development of autoimmune responses occurring in H2b-bearing conventional BXSB mice. In contrast, (NZB × BXSB)F1 female hybrids homozygous for H2d still developed typical SLE, although its development was markedly delayed as compared with mice homozygous for H2b. This indicates that the genetic complementation of NZB and BXSB genomes allows the development of spontaneous autoimmune responses in the context of H2d, even without the Yaa mutation. More strikingly, the Yaa mutation dramatically accelerated the progression of SLE in (NZB × BXSB)F1 H2d mice to an extent comparable with that observed in F1 H2b mice. Thus, no more MHC association was evident in these F1 hybrid males when they expressed the Yaa gene. Notably, similar results were observed in mice bearing the Faslpr mutation; the production of autoantibodies in B6 mice bearing the Faslpr mutation was highly dependent on H2b,48 while lupus-like disease was developed equally well in both H2k and H2b lupus-prone MRL-Faslpr mice.49 All these experiments indicate that the MHC class-II genes likely provide at least some of the genetic requirements for the predisposition to SLE, and that conventional MHC class-II molecules are sufficient in mice with an appropriate autoimmune genetic background. Most significantly, the MHC-linked autoimmune promoting effect is no longer apparent in mice which are highly predisposed to SLE, for example by powerful autoimmune accelerating genes, such as Yaa or Faslpr.
The autoimmune inhibitory effect of the H2d and H2k haplotypes, as compared with H2b, can be related at least in part to the expression of I-E molecules, since mice bearing the H2b haplotype do not express I-E because of the deletion of the promoter region of the Ea gene. The development of SLE was almost completely prevented in BXSB (H2b) mice expressing two copies of an Ea transgene encoding I-E α-chains, which is the case of H2d and H2k BXSB mice. In addition, the expression of two functional Ea (one transgenic and the other endogenous) genes in either H2d/b (NZB × BXSB)F1 or H2k/b (MRL × BXSB)F1 mice provided protection from SLE at levels comparable to those conferred by the H2d/d or H2k/k haplotype.47 These results suggest that the reduced susceptibility associated with the I-E+ H2d and H2k haplotypes (vs. the I-E− H2b haplotype) is largely, if not exclusively, contributed by the Ea gene. This idea is further supported by the recent demonstration that (NZB × NZW)F1 mice expressing I-Ad but lacking I-E molecules developed SLE as severe as that of wild-type H2d/z heterozygous (NZB × NZW)F1 mice.50 However, it should be stressed that since H2d/z (NZB × NZW)F1 mice express I-E, the unique autoimmune-promoting effect conferred by the H2d/z heterozygosity apparently overcomes the protective effect of I-E in this genetic background, as in the case of (NZB × BXSB)F1 mice expressing the Yaa mutation and MRL mice bearing the Faslpr mutation.
The precise mechanism(s) responsible for the Ea gene-mediated protection from SLE remains to be elucidated. Studies of Ea transgenic and nontransgenic mixed-bone-marrow chimeras revealed that these chimeric mice developed a typical lupus-like autoimmune syndrome, in which anti-DNA autoantibody production was preferentially induced by nontransgenic B cells.51,52 These results suggested that B cells are the major target of Ea-mediated suppression of autoimmune responses, and that Ea gene expression may interfere with an efficient interaction between autoreactive T and B cells, possibly by modulating the presentation of pathogenic self-peptides by MHC class II molecules. This could occur as a result of increased formation of peptides derived from the I-E α-chains. In fact, one of the peptides, Eα52-68 peptide, has been identified as one of the major self-peptides presented by I-A molecules.53,54 The hypothesis that the protective action of the Ea gene is mediated through binding of its degradation products by MHC class-II molecules, thereby competing with potentially pathogenic self-peptides, was further supported by the demonstration that the protective effect of the Ea transgene was highly dependent on the host H2 haplotype.55 This idea was consistent with recent in vitro demonstration that the capacity of Ea transgenic B cells to activate T-helper cells by presenting I-A—restricted peptides of foreign antigens was substantially diminished, compared with that of nontransgenic B cells.56
The MHC class-II Ea gene apparently contributes to the reduced susceptibility to SLE by suppressing autoimmune responses in mice, but its protective effect is influenced by the host H2 haplotype. However, the Ea gene is not the only gene encoded within the MHC region that determines the genetic susceptibility to murine SLE, and the MHC region likely encodes additional lupus-associated genes, which can potentiate or suppress the development of SLE by acting at various levels of the disease process. Studies have claimed the presence of a lupus suppressor gene, Sles1 (SLE suppressor 1), within the H2 region of the NZW strain,57 although it remains to be confirmed whether the observed suppressive effect was due to this novel suppressor gene and not to MHC region polymorphisms. In addition, the Tnfa allele of the NZW strain, which is associated with down-regulated TNF-α synthesis, has been previously proposed as a candidate gene that may underlie the H2z contribution to lupus in (NZB × NZW)F1 mice.58,59
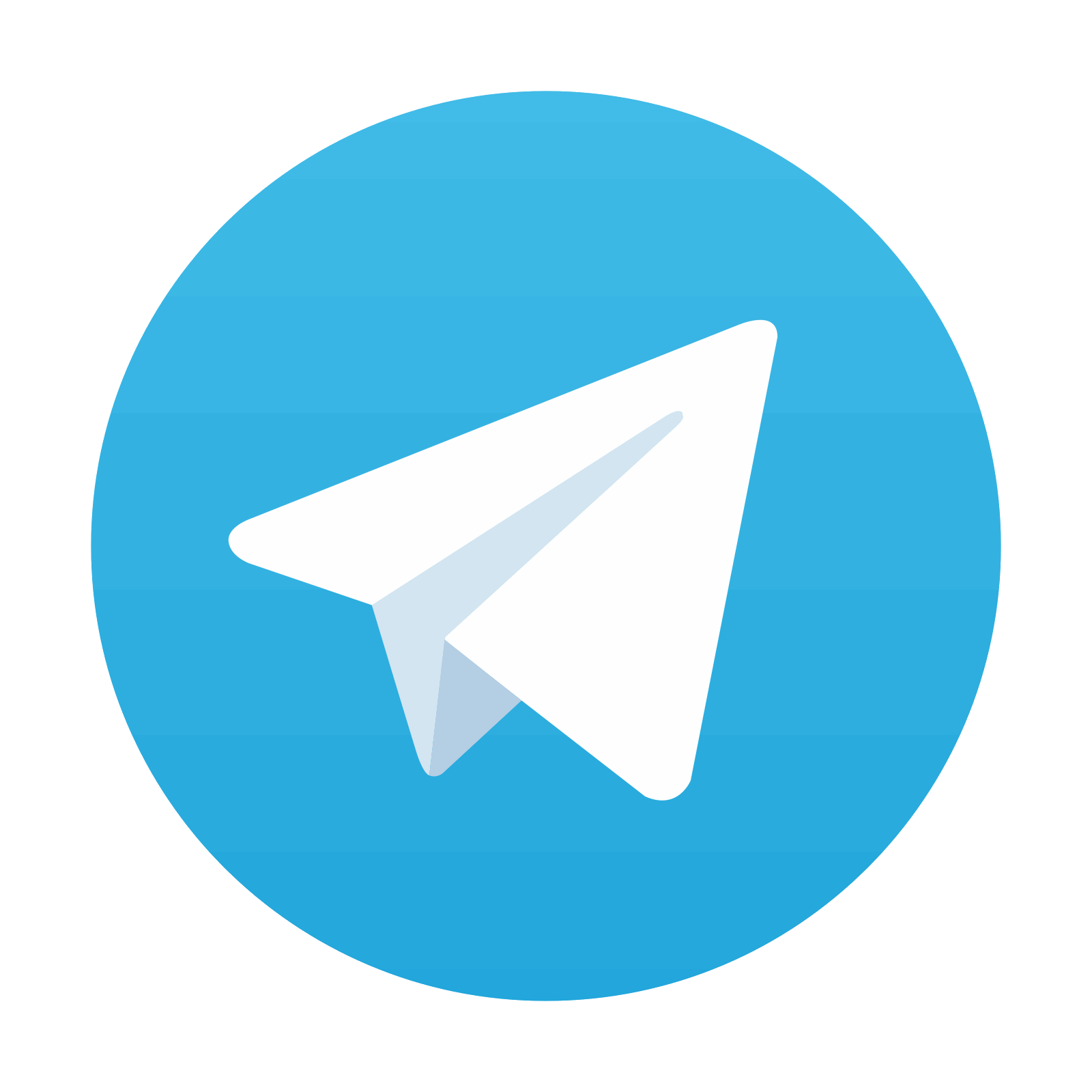
Stay updated, free articles. Join our Telegram channel

Full access? Get Clinical Tree
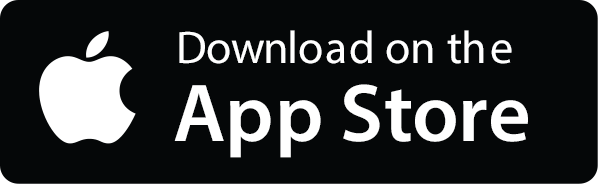
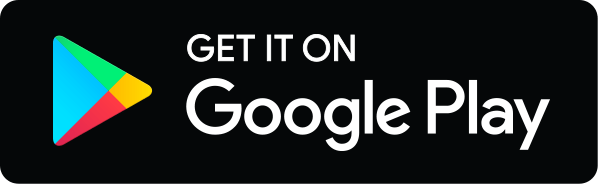