Chapter 4 Gene Therapy in the Treatment of Knee Disorders
The knee is one of the most commonly injured joints in athletes and the most common load-bearing joint to progress to development of arthritis.172,238 Over the past 20 years, treatments for knee disorders have been advanced by improved technologies and a greater understanding of normal knee biology and biomechanics. Sports medicine specialists focus on the treatment of traumatic and overuse knee injuries to allow early return to athletic activities and to help prevent progression to joint failure, typically in the form of osteoarthritis (OA). Ligament reconstruction in anterior cruciate ligament (ACL) and posterior cruciate ligament (PCL) injuries helps athletes regain anterior/posterior translational and rotational stability, and treatment of meniscal injuries relieves joint line pain and tenderness, as well as locking and catching, which can limit an athlete’s activities. Early treatment of joint instability secondary to ligamentous injury, meniscal tears, and defects in the load-bearing portion of the joint may help prevent joint failure.87,121 Ongoing work to evaluate the benefit of anatomic repair of both ACL and PCL in improving knee stability and in preventing OA is under way.68,456 Meniscus repair and allograft replacement have become the standard of care when possible, and partial meniscectomy has been limited to the region of disease, as meniscectomy has been proven to increase the risk of OA.70,92–94 Finally, chondral defects associated with joint injury and OA are better appreciated with newer imaging techniques, and this may lead to improved therapy.13,321 A broad array of treatments for cartilage defects are available, and newer tissue engineering–based treatments are continually in the pipeline, with the goal of improving the durability of repair tissue. Overall, improved surgical techniques aim to restore joint biomechanics, which may continue to improve early and late outcomes of knee injury.
Despite these improvements, the field of sports medicine remains bound by the realities that many intrajoint tissues do not heal well, and that acute joint injury, instability, altered joint biomechanics, and changes in the load-bearing surface contribute to secondary OA.252 Traumatic joint injuries, including ACL tears and meniscal tears, are associated with increased risk of OA.179,191,252 ACL reconstruction is associated with a roughly 20% complication rate, which includes instability and failure, and ACL reconstruction does not appear to significantly decrease the risk of OA associated with ACL injury.* Cartilage wear is known to increase with removal of meniscal tissue. In addition, despite significant advances in treating chondral and osteochondral defects, a significant number of cases go on to develop early OA. Further, posttraumatic OA after tibial plateau fracture was reported to range from 21% to as high as 44% in approximately 7 years, which may be secondary to both acute injury and poor reapproximation of the articular surface.186,345 Because ligamentous and meniscal injuries of the knee rarely occur as isolated injuries,117,377,399 many have hypothesized that the total effects of acute traumatic knee injury likely play a role in progression to OA, as do changes in biomechanics. Although sports medicine continues to better address repair of soft tissues and to restore normal biomechanics to the knee, a great deal of work remains to better understand and treat acute joint injury and to promote repair. Ultimately, joint replacement represents an excellent salvage procedure for the knee; however, knee joint prostheses do not recapitulate the function of a normal functioning knee and are prone to wear and alteration in knee stability and biomechanics. Gene therapy and tissue engineering represent alternative approaches to biologics therapy. Gene therapy has the potential to play a role in the treatment of joint injury, acutely and chronically, as well as in tissue engineering and repair applications in the knee, which ultimately may change the rate of progression to OA or joint failure.
In addition to injury and overuse, rheumatologic diseases, in particular rheumatoid arthritis, affect the knee and ultimately can lead to severe arthritis requiring joint replacement. Rheumatoid arthritis (RA) causes significant synovitis, which over time leads to destruction of the intra-articular tissues; classic disease-modifying therapies are only moderately effective and are patient dependent. The invention of biologics, namely, anti–tumor necrosis factor (TNF)-α and anti–interleukin (IL)-1 therapies, has revolutionized the treatment of RA by dramatically decreasing inflammation and associated pain and possibly joint destruction.134,451 Even with this new treatment, many continue to have low-level inflammation and joint destruction, and a small population of individuals with RA do not respond to available anticytokine therapies. In addition, because of the strong anti-inflammatory potency of the biologics, the risk of systemic infection is much increased, particularly for tuberculosis and histoplasmosis.409,410 Gene therapy offers the benefit of local, intra-articular treatment that may dramatically decrease systemic side effects; in addition, the therapy may be longer lasting and therefore may decrease the cost of care.104
Gene Selection
Gene selection depends on the intended use of gene therapy. Maintaining a progrowth, anticatabolic phenotype of cells or the cellular apparatus through addition of growth and differentiation factors and anti-inflammatory proteins/peptides has proved to be an effective approach to the treatment of musculoskeletal disease in animal models. Gene therapy has been used for inhibition of inflammatory processes, which can lead to breakdown of connective tissues and can inhibit repair. Inhibition of proinflammatory cytokines, TNF-α, IL-1β, and, more recently, IL-6 has proved effective in treating inflammatory arthritis both in animal models and in human disease. It is not surprising that gene therapy trials for RA have focused on targeting inhibition of proinflammatory cytokines such as TNF-α and IL-1β, and early data suggest some efficacy (for review106). In addition to IL-1β and TNF-α, gene therapy targeting cytokines such as IL-13,163,439 IL-4,164,438 IL-18–binding protein/IL-4 fusion,245 IL-10, and modified prothymosin,376 and small interfering RNA targeting TNF-α,214 IL-1/IL-6/IL-18,215 and the p65 component of nuclear factor kappa B (NFκB)66 have shown some efficacy in inflammatory arthritis models.
Gene therapy–targeted tissue repair requires a basic understanding of tissue development and of the biology of normal tissue homeostasis, as well as of normal repair mechanisms and proanabolic factors. For example, articular cartilage is well studied using both in vivo and in vitro tissue engineering models; factors such as insulin-like growth factor (IGF)-1/2, transforming growth factor (TGF)-β (1 to 3), bone morphogenetic proteins (BMPs), and basic fibroblast growth factor (bFGF) have all been used effectively to enhance chondrogenesis and/or cartilage biosynthesis (for review99,106,166,386). Therefore, gene therapy strategies have focused on these growth and differentiation factors. Similarly, growth factors such as FGF, IGF, platelet-derived growth factor (PDGF), TGF-β, growth and differentiation factor (GDF)-5–7, and vascular endothelial growth factor (VEGF) all may have some efficacy in ligament and tendon repair; many of these factors have also been used with some efficacy in a gene therapy–based approach to augment healing (for review435). An alternative approach to overexpressing or inhibiting small growth factors and cytokines may involve targeting the intracellular signaling apparatus of the cell, including progrowth and differentiation-specific transcription factors. Gene therapy may also be used to deliver extracellular matrix molecules, which may alter the organization or composition of the extracellular matrix. Alternatively, gene therapy may focus on enhancing joint lubrication by increasing the production of enzymes important in hyaluronic acid synthesis or in synthesis of lubicin, or it may target inhibitors of proteases responsible for degradation of the extracellular matrix. Given the diverse and biologically complementary candidate genes for gene therapy, technology may provide a robust arsenal of potential treatment options for the future treatment of knee pathology.
Vectors and Expression
The method of transfer of genetic material is of importance; nucleic acid transfer efficiency, immunogenicity, delivery, and stability of construct are all necessary considerations. Gene transfer vectors are usually divided into nonviral vectors, which include all methods of transfection, and viral vectors, which use genetically altered viruses to infect the cell with the DNA of interest through a process known as transduction (Table 4-1).
Nonviral Vectors
The transfer of genetic material, namely DNA, without the use of a virus is referred to as transfection (for review79,166,441). The most basic transfection method is performed with DNA plasmid alone or complexed to calcium phosphate and introduced through “holes” formed in the cell membrane; transfection efficiency is low.109,157,432 Exposure of cells or tissue to pulsed electric fields, a process called electroporation, transiently increases the permeability of the cell membrane and encourages the transit of more DNA, typically in the form of plasmids.42,43 The use of liposomes as delivery vehicles allows fusion with the lipid membrane and enhances the delivery of nucleic acid contents into the cell, enhancing transfection efficiency.244,272 Complexing the DNA with polycations or protein ligands relies on cell endocytosis and has been proven to increase transfection efficiency as well.120,398 The “gene gun” is a process by which microprojectiles of gold or tungsten are coated with the DNA of interest, accelerated by high-pressure helium or a high-voltage electrical discharge, and essentially shot through the cell membrane.222 The gene gun method is a single-cell-at-a-time gene delivery method and therefore is likely used more appropriately as an ex vivo technique. Once inside the cell, the DNA, often in the form of a plasmid, is able to harness transcriptional machinery for expression of the DNA. Because plasmids typically do not integrate into the chromosomal DNA, the plasmid may be lost through cell division or other cellular mechanisms, thus limiting lifetime expression. To achieve long-term expression, transposons are being used to enhance the integration of plasmid DNA into the host genome, as are specific nucleic acid sequences, to aid in homologous recombination and the addition of endonucleases to the plasmid or viral gene repertoire that assist in DNA integration.212,329 Advantages of nonviral vectors include their relatively low cost and nearly complete lack of immunogenicity. The major disadvantage of nonviral vectors, as alluded to, is their limited transfection efficiency and transient expression.
Viral Vectors
The transfer of genetic material by infection with a virus is known as transduction and occurs with high efficiency as long as the tissue can be penetrated to allow viral access to cells. Under normal conditions, viruses are designed to infect cells by transferring their genetic material in the form of DNA or RNA into the cells. The viral DNA then harnesses the cellular machinery to replicate its DNA and virus particle constituents for virus propagation. For the purpose of gene therapy, viruses are reengineered, eliminating the viral genes responsible for viral replication, cytotoxicity, immunogenicity, and viral particle packaging, while maintaining only what is necessary for expression of the engineered nucleic acid contents (for review397). To achieve virus formation, a second virus, or “helper virus,” such as herpes simplex virus (HSV), is cotransduced and contains the additional viral genes necessary for packaging of the engineered viral construct. The engineered viruses are then purified classically by density centrifugation, or more efficiently by column chromatography, for in vivo or ex vivo administration.161 Despite significant work, viruses still possess some immunogenicity, which can negate some of their beneficial effects and may pose at least a theoretical safety risk.356 Viruses used to transfer genetic material for gene therapy include HSV, adenovirus (AdV), and adeno-associated virus (AAV), whose nucleic acid material exists as a separate episome within the cell nucleus, as well as lentiviruses and oncoretroviruses, whose genetic material integrates into the host genome, allowing for long-term stable transgene expression. Each virus has a unique set of advantages that make it amenable to certain uses; all vectors are designed to mediate gene delivery and transgene expression while minimizing harmful side effects. For musculoskeletal disease, AdV, AAV, and lentiviruses have been used most frequently.
AdV is a double-stranded DNA virus that is capable of infecting both dividing and nondividing cells with high efficiency; however, transgene expression is transient both because the genetic material does not integrate into the host genome and because of its immunogenicity. Although the original AdV vectors were highly immunogenic,334,446,447 reengineering of the virus has dramatically decreased its immunogenicity, which results in prolonged transgene expression and improved safety.397 AdV was identified early on as the virus of choice for musculoskeletal tissue engineering uses, in part because of its high infection efficiency and its limited transgene expression lifetime.99 The original musculoskeletal gene therapy trial was performed for the treatment of RA and used AdV ex vivo gene therapy to transfer the IL-1–soluble receptor (IL-1Ra) into affected metacarpophalangeal (MCP) joints to block signaling of IL-1, a cytokine associated with inflammation and cartilage breakdown.96,100
Adeno-associated virus (AAV) is a small, single-strand DNA virus with limited transgene expression and low immunogenicity (for review397). Despite good infectivity, native AAV transgene expression is somewhat limited secondary to poor conversion of the single-strand DNA genome to double-strand DNA necessary for transgene expression and stabilization.116 This has been partially overcome by the development of self-complementary (sc) AAV, which was used by Kay et al for delivery of IL-1Ra with improved efficiency of transduction and expression.210,285 In addition to its low conversion efficiency, AAV has space for only approximately 2.5 kb of genetic material, although it may be expanded with possible concatemerization of multiple transgene sequences to reconstitute a larger transgene sequence.84,85,455 Similar to AdV, AAV exists as a nonintegrating episome in the nucleus and may limit duration of expression; however, AAV is not known to cause human disease and is much less immunogenic than AdV. Because of its safety and lack of viral gene expression, AAV is gaining favor as the vector of choice for joint disease. Ongoing work to identify additional serotypes may allow better targeting to cells of interest.397 Boissier et al evaluated the ability of different AAV serotypes to infect synovial fibroblast in vitro and identified AAV1>AAV2>AAV5>AAV8; however, this finding was balanced by the greater presence of neutralizing antibodies in the synovial fluid of AAV1 and AAV2 compared with AAV5 and AAV8.145 To date, AAV5 and AAV2 are used most frequently in mouse and rat models of joint diseases with good effect.5,14,216 A patient enrolled in a gene therapy trial for RA using AAV transfer of a TNF-α inhibitor died during the treatment trial.131 Although investigators found that the death was most likely due to systemic histoplasmosis, a fungal infection, rather than to effects of the virus, additional trials may be more carefully scrutinized as a result of the death.
HSV is a complex double-stranded DNA virus with a large capacity for foreign DNA sequences.237 Native HSV-1 is a large virus that can enter a lytic or a latent state with infection; the virus has a natural tropism for neurons and is capable of infecting and remaining latent within neurons for long periods, making it an ideal vector for treatment of neuropathology. Similar to AdV, HSV efficiently infects both dividing and nondividing cells, remains as an episome (nonintegrating), infects a wide range of host cells, and possesses high immunogenicity and cytotoxicity.356 The advantage of the virus is that transgene expression may occur over the long term without the risk of insertional mutagenesis; also, the virus is able to tolerate approximately 40 kb of DNA, which allows for polygene or large gene expression. Although significant reengineering of the virus has taken place, maintaining transgene expression with concomitant latency of the virus continues to be a challenge.397 Despite these limitations, intra-articular gene therapy using an HSV vector containing IL-1Ra was effective in reducing inflammation in a rabbit model of IL-1β–induced arthritis,323 and studies have demonstrated gene expression by intra-articular injection for at least a year.431
Oncoretroviruses were the first gene therapy vectors and have been used extensively for gene therapy with the capacity to infect only dividing cells (for review324,350,397,441). Oncoretroviruses are RNA viruses that are reverse transcribed to DNA and can be integrated into the genome, which provides long-term stable expression of the transgene but increases the risk of insertional mutagenesis. To this point, an ex vivo approach using hematopoietic progenitor cells transduced with murine leukemia virus (MLV), carrying a transgene encoding for the γ C-chain cytokine receptor subunit for treatment of severe combined immunodeficiency (SCID), resulted in cure of the disease; however, a subpopulation of treated patients developed leukemia as the result of insertion of the transgene into the T-cell oncogene, LMO2, indicating the need for caution with use.63,165 To date, retroviruses have been used extensively for ex vivo gene therapy in tissue engineering constructs with some benefit; however, some believe that long-term stable transgene expression may not be required and may be harmful in tissue engineering approaches. Retroviruses represent an ideal vector choice for ex vivo gene therapy with tried and true results.
Lentiviruses are also retroviruses, but they possess the ability to infect nondividing cells. Similar to oncoretroviruses, lentiviruses contain RNA that is reverse transcribed into DNA; however, they differ from oncoretroviruses in that lentiviruses may penetrate the nuclear envelope to incorporate into the host genome, allowing them to infect both dividing and nondividing cells. Limitations of lentiviruses include the difficulties encountered in the production of high-titer virus stocks and safety concerns related to their derivation from human immunodeficiency virus (HIV) or HIV-like viruses.266 Gouze et al explored the use of lentiviral vectors for intra-articular gene therapy of IL-1Ra for treatment of IL-1β–induced arthritis and showed good efficiency of gene transfer to synovial cells; however, transgene expression was limited to approximately 20 days.153 Later work with better engineered lentiviral vectors, addressing limitations of this earlier study, reported transgene expression for longer than 6 weeks.155 Lentiviruses also have been used in animal models to treat monogenic autosomal recessive diseases, which require long-term expression, including mucopolysaccharidosis type VII, in mice.51 More recently, mucopolysaccharidosis VI, caused by deficiency of N-acetylgalactosamine-4-sulphatase that affects multiple tissues, including cartilage, and results in development of severe arthritis, has been treated with intra-articular injection of a lentiviral vector containing the deficient enzyme.55 Although the viral vector was unable to penetrate the cartilage or ligament, previous enzyme replacement studies suggest that sufficient enzyme was expressed by the synovium to promote treatment.27 Finally, a recently published human study showed the efficacy of ex vivo lentiviral therapy in hematopoietic stem cells for the treatment of adrenoleukodystrophy in two patients, providing early data on the safety and efficacy of treatment.61 Lentiviruses may serve as a tool for both in vivo and ex vivo gene therapy in musculoskeletal disorders.
Regulating Expression With the Use of Promoters
Another means of controlling transgene expression is the use of regulatable promoters, often termed enhancer-promoters (EPs) (for review162). The promoter is a cis-acting genetic sequence typically upstream of the coding region that serves as a recognition signal for transcription activators to direct transcription machinery. Vectors can now be constructed to contain promoter sequences, which are constitutively on and responsive to transcriptional factors within the cell, or are responsive to the presence or absence of externally applied chemicals. Among constitutive promoters, such as Rous sarcoma virus (RSV) or human cytomegalovirus (CMV), tissue and cell selectivity has been noted.52,326 Examples of tissue-specific EPs are the glial fibrillary acidic protein (GFAP) for astrocytes375 and the collagen II promoter for chondrocytes.462 Disease-specific regulation of transgenes has been attempted with some success in inflammatory arthritis models, using inflammation-inducible promoters such as an IL-1 enhancer sequence in front of the IL-6 promoter,414 a complement C3–based promoter,414 an NFκB-responsive promoter,5,29,216,414 and a cyclooxygenase (COX)-2–based promoter.343 Disease-inducible promoters may find a home in the treatment of chronic joint disease, in particular, rheumatoid arthritis, in which the transgene is activated only with increased disease activity, which may limit side effects.
The classic pharmacology-based regulatable promoter system consists of tetracycline-activated promoters and requires the drug tetracycline (tet) to initiate or inactivate gene transcription (for review of “tet-on” and “tet-off” systems, see156,419). Using this technology, regulation of transgene expression can be accomplished by modulating tetracycline in the drinking water of the animal host, or by adding into or subtracting from the cell culture medium for in vitro applications.136,303 Besides tetracycline, chemical-based regulatable promoters have been constructed that recognize progesterones (mifepristone),425 rapamycin,348 ecdysone,320 doxycycline, and streptogramin.152,416 The tet-on regulatable system has been used with AAV–IL-10 gene transfer to regulate expression in an experimental RA model.16 Similarly, Gould et al used a doxycycline-regulated plasmid vector containing a dimeric soluble TNF-α receptor II with limited efficacy caused by poor transfection but maintained regulation of transgene expression.152 Finally, the Cre-Lox system (DuPont, Wilmington, DE) allows elegant control of initiation or termination of transgene expression; however, because a fragment of DNA is removed from the construct, this regulatory approach is irreversible.203
Methods of Delivery
Gene therapy can be administered directly into organisms (in vivo) or to explanted cells or tissue that can be reimplanted or injected (ex vivo); each of these approaches involves delivery locally to the site of interest or systemically (Fig. 4-1). The capacity to select a particular target cell using an in vivo approach is limited, and ex vivo treatment allows specific targeting of the cells of interest but requires isolation of those cells prior to treatment. Administration occurs locally or systemically, and intravenous administration of in vivo or ex vivo gene therapy may be used for the treatment of Mendelian disease or inflammatory disease with systemic manifestations. However, most repairs, tissue engineering, and treatment of joint disease can be performed through intra-articular or site-specific injection with improved safety; therefore, this has been the standard of care. Whether local or systemic, in vivo or ex vivo, the role of the immune response in transgene expression is a critical consideration for both in vivo and ex vivo therapies.
In Vivo Gene Therapy
For use in treating knee pathology, an in vivo approach with administration of the vector–transgene construct may be used through intra-articular injection; through bursa injection; to the site of cartilage, meniscal, tendon, or ligament defect; or within a tissue engineering construct. Cells embedded in dense extracellular matrix may be difficult to target; unfortunately, this is the case for many tissues of the knee. In the case of arthritis treatment or treatment of cartilage lesions, intra-articular injection of the virus–transgene construct is more likely to infect the synovial cells than the cartilage cells55,153; however, intra-articular gene therapy using smaller secreted growth factors or cytokines as transgenes generates products that are able to penetrate the dense cartilage matrix and have an impact on chondrocyte behavior, making it a potentially useful approach. In addition, sites of injury are often more exposed and may be more amenable to treatment. Tendon and ligament, because they are more vascular, may benefit from local injection near the site of injury or, in theory, controlled local intravenous injection, although systemic delivery introduces increased risk and potential side effects of gene therapy. Nonviral and viral transgene constructs may also be embedded in tissue engineering scaffolds used to enhance cartilage, meniscus, or ligament repair.
Stem Cells and Ex Vivo Delivery Devices
Stem cells are capable of long-term proliferation, self-renewal, and the ability to differentiate into multiple lineages; they also possess the capacity for tissue repair and regeneration. Embryonic stem cells are truly pluripotent cells, meaning that these cells, under the appropriate conditions, may differentiate into any and all cell types of the organism and therefore are capable of complete regeneration of that organism. Embryonic stem cells have seen limited use, primarily as the result of governmental restrictions with challenges regarding control of differentiation and immunogenicity that have not been researched adequately. Induced pluripotent stem cells (iPS) have similar functional capability as embryonic stem cells and arise with low efficiency from reprogramming of partially or fully differentiated cells.444,445 Control of differentiation is a similar concern for iPS, as is increased risk of carcinogenesis.44 Adult stem cells represent progenitor cell populations that have multipotent potential but do not have the plasticity of embryonic stem cells. Postulated properties of all adult stem cells include quiescence, capability of asymmetrical cell division, ability to differentiate into multiple lineages, and ability to undergo significantly more replicative cycles than other cells.274,374
Adult mesenchymal stem cells were originally identified in bone marrow and differentiate into mesenchymal tissues, including bone, tendon, cartilage, fat, or muscle; they are the stem cell of choice for most musculoskeletal applications (Fig. 4-2) (for review65). Other adult mesenchymal stem cell populations include muscle-derived stem cells,342 synovial tissue–derived stem cells,225,316 periosteal-derived stem cells,139 and fat-derived stem cells,454 all of which have shown the potential to differentiate into multiple cell types, particularly of mesodermal origin. At this point, many have hypothesized that all tissues possess stem cells that may be responsible for the turnover, regeneration, and/or repair of that tissue. Recent studies suggest that the blood vessel pericytes and endothelial cells may be the origin of mesenchymal stem cells identified in a variety of tissues.57,72,461 This finding may serve as a possible explanation of the relationship between vascularization and repair and regeneration in tissues throughout the human body. Although stem cells alone serve as a powerful therapeutic, they have been employed in cell- or tissue-based engineering in conjunction with gene therapy to enhance healing of musculoskeletal tissues.
Stem cells serve as vectors for gene therapy when an ex vivo approach is used, but they likely offer additional therapeutic benefit. Gazit et al reported that MSCs transduced with a retrovirus-encoding BMP-2 healed an osseous defect more completely than transduced Chinese hamster ovary (CHO) cells, despite the fact that the CHO cells expressed quantitatively more BMP-2.137 Their results suggest added benefit of MSCs for tissue repair. Moutsatsos et al showed that MSCs expressing BMP-2 produced more bone in a mouse radial defect model than was produced by injection of recombinant protein, thus illustrating the benefits of prolonged gene expression using a gene therapy–based approach in bone repair.303 Choi et al used an ex vivo approach by transducing MSCs with retrovirus–IL-10, which was injected systemically, for treatment of collagen-induced arthritis with efficacy in a murine model, suggesting that MSCs with gene therapy may provide a safer systemic delivery vector than in vivo gene therapy.69
Tissue Engineering
The goal of tissue engineering is to generate functional replacement tissue that can be used to treat defects in cartilage, ligament, tendon, bone, meniscus, blood vessels, muscle, and so forth. Tissue engineering–based approaches are already being employed in clinical practice for cartilage defect repair with methods such as autologous chondrocyte implantation (ACI) and matrix-induced autologous chondrocyte implantation (MACI).173 Tissue engineering is thought to involve three main components: (1) a cell source that may be recruited or isolated and expanded; (2) a scaffold, matrix, or substrate that will form the tissue framework; and (3) various cytokine and growth and differentiation factors, which will improve growth and maintain the phenotype of cells within the construct. The cell source may be cells harvested from the tissue of interest, or cells may be derived from an adult or embryonic stem cell or from precursor populations that are differentiated into the cell type of interest using specific growth and differentiation factors and proper biologic, chemical, and physical conditions. The scaffold or substrate needs to be sufficiently large to span the size of the defect, integrate with the surrounding tissue, have sufficient mechanical properties to protect the cells early following seeding and implantation, provide a bioactive environment for cells seeded in the scaffold to proliferate, and maintain cell phenotype to deposit appropriate matrix that recapitulates the tissue of interest or at least the tissue properties of interest (for review389,411).
Considerations for Clinical Development
Since the original musculoskeletal gene therapy trial targeting treatment of RA,96 Evans and colleagues continue to push forward the concept of treating arthritis through intra-articular gene therapy–based approaches (for review98,100,102,104). In fact, an early report of the first phase II clinical trial shows clinical response to IL-1Ra ex vivo gene therapy in two subjects.428 For RA and other chronic joint diseases, the power of gene therapy lies in its potential to achieve sustained intra-articular release of a particular gene of interest, which will provide long-term treatment to the joint with limited systemic side effects at lower cost.104
Achieving a Better Understanding of Intra-articular Gene Therapy
Despite the use of a variety of vectors and transgenes, therapeutic expression of transgenes following intra-articular injection has been limited to approximately 2 to 3 weeks.* Gouze et al asked and attempted to answer the question, “Can transgenes be functionally expressed in the synovium for a sufficient length of time to justify the use of gene-based therapies for chronic joint diseases?”155 In this study, designed to evaluate the limited duration of transgene expression, Gouze et al showed (1) that an immune response to the vector and transgene resulted in a significant decrease in length of transgene expression (with immunologically compromised rats able to maintain expression throughout their life span); (2) that transgene stability was similar between episome and genome-incorporated virus vectors; (3) that a decrease in transgene expression correlated with a decrease in viral genes, suggesting that loss of transgene rather than promoter silencing was limiting expression; and (4) that two separate components to the expression decay curve were present, which corresponded to two cell populations—a transient expression population (synovial cells with an apparent half-life of less than a month) and a sustained expression population (fascia, ligament, tendon, and joint capsule cells).155 With these findings, they then used a lentivirus with no viral proteins coded and containing only rat soluble tumor necrosis factor receptor (sTNFR) transgene to show persistent expression of the transgene, similar to that in nude rats, indicating proof of concept.155 Similar long-term transgene expression was achieved through intramuscular injection of AAV containing the soluble bivalent TNF-α receptor with therapeutically elevated serum protein expression levels lasting longer than a year in a rat inflammatory arthritis model.360 Taken together, to achieve long-term expression, immunologic response to both the vector and the transgene must be limited, and the appropriate target cell must be identified, whose lifetime and ability to express the transgene allow for long-term expression.100 In the case of the knee, collagen-rich tissues within the joint and joint capsule appear to be the best targets for long-term transgene expression.
Translating New Understanding to Practice
At this point, AAV has emerged as the virus of choice for the treatment of arthritis. AAV, particularly serotypes 2 and 5, has proven safe for intra-articular injection with reasonable transduction efficiency, which can be improved by using self-complementary virus.14,210 In addition, cytokines and growth factors are typically small and will fit within the size constraints of the virus. Inducible promoters may allow expression of the transgene only with disease flares and therefore may decrease the risk of side effects. With these newer advances, the possibility of delivering intra-articular gene therapy for the treatment of knee disorder is technically safer and more effective.
Clinical Trials—Arthritis
Over the past 10 years, gene therapy trials in RA and more recently in OA have begun to emerge slowly and with small numbers of patients (for review103,105,106). The first phase I trial for ex vivo IL-1Ra gene therapy was completed by Evans et al at the University of Pittsburgh with nine patients.100 Although the purpose was to prove safety, evidence of efficacy was also noted in some patients within the time frame of the study. A second phase I trial was performed by Roessler et al at the University of Michigan, using in vivo gene therapy with HSV-tk (thymidine kinase), which was used in combination with ganciclovir to achieve pharmacogenetic synovectomy similar to that seen in the animal model.362 The single patient treated was noted to receive therapeutic benefit, but the study was ended because of poor enrollment in light of the death of a patient in another gene therapy study, which resulted in significant public apprehension of gene therapy as a therapeutic modality. A small phase II trial was performed by Wehling et al, who also used an ex vivo approach with IL-1Ra in Germany on two patients and provided evidence of some efficacy.428 Phase I and phase II clinical trials have been performed by Mease et al using in vivo intra-articular gene therapy with TNFR/Fc (protein product is the same as Etanercept) in inflamed knees.289 The phase I study was done at viral concentrations that were an order of magnitude lower than those necessary to see an experimental effect with RA.289 The trial continued and is now completed with no report of results. In addition to trials for RA, two OA clinical trials are under way in South Korea using TGF-β1; both are using an ex vivo intra-articular approach. To date, no results have been reported from these trials. Given the fact that animal models have shown that intra-articular overexpression of TGF-β1 results in fibrosis and osteophytosis, the results of these trials may improve our understanding of OA.107
Meniscus
Meniscus injury is the most common intra-articular injury to the knee,28,223 and meniscal tear due to trauma, sports-related injury, or degeneration is the leading indication for arthroscopic surgery.287 The menisci consist of crescent-shaped fibrocartilage, which sits between and adjacent to the contact surfaces of the femur and the tibia of the knee and is responsible for load distribution,34,230,368,369 shock absorption,230,420 and some joint stability, particularly in the absence of ACL,8 and may play a role in surface lubrication, nutrition, and proprioception (for review287,352). Although it was initially the standard of care, meniscectomy is now known to cause articular cartilage damage227,238 and to increase the risk of OA.353 At the same time, treatment of meniscal lesions is associated with early improvement of symptoms and may protect the meniscus and the chondral surface from further injury. Therefore, treatment for meniscal pathology now focuses on conservative removal of degenerated tissue (partial meniscectomy) and primary repair to injury within vascularized meniscus tissue (for review286). A major anatomic obstacle of meniscal repair mirrors the difficulty experienced with repair of articular cartilage defects: Although injury to the peripheral vascular zone can heal, the inner two thirds of the meniscus is avascular and thus cannot adequately support a regenerative or reparative process.261 Newer allograft and meniscal replacement approaches are being used to treat irreparable meniscal lesions, with good early and medium-term results70,267; however, these procedures are technically demanding and carry with them incumbent dangers of allograft use, including disease transmission, immunogenicity, and graft failure.267 Additional techniques such as gene therapy and tissue engineering may offer alternatives or assistance in repair.
Gene therapy used for repair and tissue engineering of the meniscus has only begun to be realized. Work by Arnoczky et al showed improved but limited healing of the avascular portion of the meniscus with placement of a fibrous clot scaffold, which investigators proposed was secondary to growth and chemotactic factors present within the clot.21 Based on this work, Goto et al tested the feasibility of gene therapy as a means of achieving prolonged growth factor release for meniscus repair using both a retrovirus and AdV constructs with a lacZ reporter gene in in vitro and in vivo systems.150 Although this study showed stable transduction of a cell population with meniscus explants in vitro, the area of infected cells was limited to the site of injection; the ex vivo approach, which used scaffolds for in vivo repair, showed localized expression within the scaffold at the site of the defect for longer than 6 weeks in a canine model, suggesting possible feasibility of the method to enhance repair.150 Similarly, Madry et al used an AAV-LacZ construct to test the effectiveness of in vivo gene therapy applied directly to the site of injury for meniscal repair within the avascular region and found transgene expression in cells at the surface and near the site of the defect.270 Using a tissue engineering construct of bone marrow stem cells embedded in an alginate scaffold to treat full-thickness meniscal defects within the avascular portion of the anterior horn in goats, Zhang et al tested the improved healing capability with ex vivo transfection with IGF-1 compared with the construct without IGF-1.458 In this study, investigators were able to show increased proteoglycan content with IGF-1 gene therapy; however, proteoglycan content did not reach the level of the native tissue.458
Gene therapy has also been used to improve vascularization, which may enhance repair potential. Hidaka et al, using AdHGF-transfected bovine meniscal cells implanted in a polyglycolic acid (PGA) scaffold, showed improved neovascularization in HGF-expressing tissue–engineered meniscal graft and compared no virus versus virus expressing a reporter gene when implanted into a subcutaneous pouch of nude mice.176 To test the potential benefits of gene therapy for meniscal allograft transplantation in vivo, Martinek infected an explanted meniscus with MLV-LacZ construct, which subsequently was used as an allograft for reimplantation and was evaluated at 2 and 8 weeks.280 The meniscal allografts showed some persistence in expression, particularly at the meniscosynovial junction—a site that may be of importance in revascularization and repopulation of the allograft.280 Additional in vivo work is necessary to evaluate possible gene therapy strategies to promote meniscal healing, with in vitro work focusing on appropriate gene targets and better tissue engineering approaches.
In vitro work on the meniscus remains limited but has added to our understanding of meniscus biology and gene therapy approaches. One of the earliest in vitro studies on meniscal cells in monolayer retrovirally transduced with TGF-β1 showed improved matrix synthesis.149 In in vitro explant studies, Steinert et al used a tissue engineering approach to meniscal repair and showed that AdTGF-β–infected bovine meniscus cells implanted in a collagen-gag matrix and placed into a defect created within the meniscus explants showed improved healing compared with the construct expressing a reporter gene.385 Also in a human meniscal explant model, Cucchiarini et al showed that AAV-FGF2 applied to explants of meniscus after a created defect decreased the size of the defect over time and showed enhanced cell proliferation and fibrocartilage matrix deposition.74 Growth factors such as IGF-1 and -2,426,427 FGF-2,426,427 PDGF,38,248,382 TGF-β,149,248 HGF,38,176 and BMP-738,248 have been proposed as proanabolic cytokines for meniscus. These works suggest a possible role for gene therapy in meniscal repair by expanding the region of vascularization for repair and by using tissue engineering constructs.
Tendon
The extensor mechanism of the knee is of prime importance for knee function and is composed of the quadriceps muscle group, the quadriceps tendon, the patella, the patellar tendon (or ligament), and the tibial tubercle (for review193,283). Although mild degenerative tendinopathies of the knee are relatively common (“jumper’s knee”), tendon rupture is rare, with patellar tendon rupture occurring more often in patients younger than age 40 and quadriceps tendon rupture more frequently occurring in older patients with comorbid medical conditions. Patellar or quadriceps tendinitis or tendinosis is a common overuse injury that is frequently seen in jumping athletes; it typically responds to conservative care consisting of rest, nonsteroidal anti-inflammatory drugs (NSAIDs), and physical therapy, but in more severe cases may require surgical débridement.9,421 Tendon rupture occurs most often at the osteotendinous junction at the superior and inferior borders of the patella for the quadriceps and patellar tendons, respectively. Complete extensor tendon rupture may occur secondary to degenerative tendinopathy or more rarely with trauma, and requires surgical repair to ensure apposition and mechanical stabilization of the tendon ends for proper healing, with healing occurring in a similar fashion to that seen in most vascular tissues.283 Early surgical repair of the tendon is advocated, and excellent results have been recorded 55% to 90% of the time.226,367,378 The most common complications of tendon repair are loss of quadriceps muscle strength, decreased repair tissue strength resulting in rerupture, and decreased flexion (knee range of motion [ROM]), which may require further intervention.193 Attempts at delayed tendon repair are riddled with failure secondary to muscle and tendon degeneration with scarring and retraction of the tendon ends, and at times requiring salvage procedures such as Achilles allograft to reestablish extensor function. Tissue engineering and gene therapy approaches may offer better treatment optimization of tendon repair, particularly in cases of delayed tendon repair, for which outcomes remain suboptimal.
Tendon is a hypovascular tissue composed primarily of water (60% to 70%); most of the tendon dry weight is composed of collagen type I (90%), which is organized into microfibrils, fibrils, and fascicles surrounded by an endotenon sheath containing blood vessels, lymphatics, and nerves. The tendon fascicles are further arranged in parallel to and are surrounded by another loose connective tissue layer, referred to as the epitenon and paratenon sheath, with the entire complex making up the tendon (for review178,184). The tenocytes are aligned parallel to the collagen fibril bundles and are responsible for turnover and for maintenance of the collagen bundles. Tendinitis is associated with inflammatory changes to the surrounding tendon sheath; tendinosis refers to degeneration of the tendon tissue itself and is characterized by disorganized collagen fibrils and increased tenocyte apoptosis, associated with increased risk of tendon failure.9,421,443 Tendon rupture is thought to heal with an initial hemorrhage and inflammatory phase involving cell migration to the site of injury and removal of tissue debris, followed by fibroblast infiltration, deposition of granulation tissue rich in collagen type III to form callus, and finally, remodeling of the collagen-rich scar tissue.183,184,193 Because of the higher proportion of collagen type III, the strength of the tissue repair remains weaker than surrounding tissue, increasing the risk of rerupture.273 Further, adhesion formation can prevent normal tendon function and therefore joint motion. Gene therapy may serve as a means of treating tendinopathy or encouraging stronger repair by enhancing collagen I production during scar formation.
Tendon basic science research has identified a number of progrowth and differentiation factors that may serve as useful targets in modulating tendon repair. Growth of tenocytes in vitro has been shown to be affected by such diverse mediators as IGF-1, PDGF, TGF-β, epidermal growth factor (EGF), and bFGF.64,86,273,422–424 Differentiation factors GDF-5 (BMP-14), GDF-6 (BMP-13), and GDF-7 (BMP-12) have been associated with ectopic tendon formation.433 Analogously, null mutations in GDF-6 are associated with decreased collagen and glycosaminoglycan content within the tendons,296 and GDF-5−/− mice show delayed tendon healing,67 while GDF-7 deficiency results in no significant biochemical abnormalities but is associated with an increase in GDF-5 expression.294,295 Given the apparent role of GDF-5, GDF-6, and GDF-7 in tendon formation and healing, GDF-5 and GDF-7 have been used to improve repair in an Achilles tendon defect model.22,124–126 The previous discoveries have provided the rationale behind several applications of gene therapy for tendon. To date, experimental gene therapy work has focused on improving tendon healing associated with complete tendon rupture using patellar, digital flexor, and Achilles tendon defect models.
Early studies characterized the ability of viral and nonviral vectors to infect and express transgene reporters in tendon. Using direct injection of a hemagglutinating virus of Japan (HVJ)-liposome vector into a patellar tendon laceration in rats, Nakamura et al showed low-level expression as high as 7% on day 7 of the virus-carrying transgene, LacZ, which infected both fibrocytic and macrocytic cells within the region of repair, and later provided evidence of low-level expression throughout the tendon.315 Similarly, Lou et al used AdV containing the LacZ gene injected into the tendon sheath of toe flexor tendons of chickens and showed that cell expression of the transgene was greater in sheath cells than in tenocytes and was greatest locally at the site of injection.258 Gerich et al used both an in vivo approach with AdLacZ and an ex vivo approach with BAG-LacZ–infected allograft fibroblasts injected into an intact rabbit patellar tendon to show superior results with the ex vivo approach, however with some evidence of an early inflammatory response.140 To enhance transduction of tendon cells by direct in vivo transduction, Ozkan et al used an injection of HVJ-liposomes containing the LacZ transgene into the femoral artery ipsilateral to a patellar tendon defect and showed gene transfer to the perivascular cells within the tendon and sheath, with as many as 12% of cells expressing the reporter gene at 3 days post injection; however, no other tissues were evaluated.327 Wolfe et al used an intra-articular and direct patellar tendon injection to deliver the herpes virus carrying nerve growth factor (NGF) and were able to show long-term transgene expression in tendon and ligament cells, which resulted in elevated systemic levels of the transgene.431 Jayankura et al used an in vivo nonviral plasmid transfer approach enhanced by electroporation to deliver LacZ transgene to Achilles tendon in rat and mice and patellar tendon in rabbits, with approximately 1.5% of cells staining positive.197 To improve localization and transduction efficiency, Dai et al soaked gelatin sponges in AdLacZ and placed the construct in a surgical defect in a rat Achilles tendon with improved transduction; 6.7% of cells were positive compared with local in vivo injection, with which 2.4% of cells were positive with the same quantity of Ad-LacZ.78 Overall, in vivo injection of virus or plasmid at the site of injury or into the tendon sheath overall showed limited transduction efficiency, with tendon sheath cells more readily infected than tendon cells and some evidence of long-term expression within the tendon cells.
Given that tenocytes and sheath cells can be transduced and transfected at low levels by a variety of different means, the next question was whether delivery of growth and differentiation factors using gene therapy can augment healing. An early study by Nakamura delivering PDGF-B by gene transfer using HVJ-liposomes into patellar tendon resulted in improved early angiogenesis and collagen deposition at 4 weeks, with no significant difference by 8 weeks, but this may translate to improved healing time.314 More recently, Hou et al explored the use of TGF-β1 ex vivo gene therapy with bone marrow mesenchymal stem cells (BMSCs) compared with BMSCs alone for Achilles tendon healing and found that TGF-β1 promoted healing, including increased collagen I deposition with concomitant increase in tensile strength and elastic modulus at all time points between 1 and 8 weeks.189 A follow-up study by the same group explored the use of both TGF-β1 and VEGF-expressing BMSCs to promote healing in a rabbit Achilles tendon defect model. This study showed no benefit of VEGF compared with BMSCs alone and no added benefit with the combination of TGF-β1 and VEGF compared with TGF-β1 alone, as measured by collagen type I or type III production and by tensile strength or elastic modulus at 8 weeks.188 As IGF-1 has been shown to increase cell proliferation and matrix deposition in ligament and tendon, Schnabel et al tested whether AdIGF-1–treated MSCs were better able to repair collagenase-induced equine FDS tendon lesions; improved histologic scores with MSC treatment and very modest improvements in the mean tensile modulus of AdIGF-1–treated MSCs compared with control MSCs showed a trend toward significance.363 Given that bFGF was shown to accelerate wound closure in vitro, Tang et al used AAV2-bFGF applied locally to enhance healing of a flexor tendon injury model in leghorn chickens with increased ultimate strength at 2, 4, and 8 weeks, without an associated increase in adhesions.392 Lou et al overexpressed focal adhesion kinase, an enzyme associated with integrin signaling, using Ad-pp125FAK, and showed increased adhesion formation with healing in a chicken flexor tendon model, making it a poor candidate for gene therapy in tendons.260 Finally, Ricchetti et al used lentivirus expressing IL-10 to reduce inflammation and promote wound healing in a murine patellar tendon injury model, which showed increased tensile strength at 6 weeks post injury compared with no treatment.346 Overall, these results indicate a possible role for anti-inflammatory cytokines and growth factors to assist in tendon healing.
In addition to the growth and anti-inflammatory factors discussed previously, the TGF-β superfamily members, GDF5-7, involved in tendon formation and repair have been explored and shown to provide some efficacy in promoting tendon healing. Given the improved healing identified with GDF-7, Lou et al used AdBMP-12 (GDF-7) therapy to enhance healing, with a twofold increase in tensile strength noted 4 weeks after treatment in a chicken tendon laceration model.259 Similarly, Majewski et al used ex vivo AdBMP-12 (GDF-7) therapy of a muscle autograft to enhance healing in a rat Achilles tendon laceration model; normal tendon strength was reached as early as 2 weeks with BMP-12 therapy compared with control, which achieved normal stiffness by 8 weeks.276 To evaluate ectopic tendon formation, Helm et al injected AdBMP-13 (GDF-6) or AdLacZ into the thigh of nude mice and showed a significant increase in ectopic tendon formation with GDF-6 compared with AdLacZ over the 100-day period of study.171 In a transected Achilles tendon rat model, Rickert et al showed that treatment with AdGDF-5 (BMP-14) significantly increased tendon thickness, with a trend toward increased strength at 8 weeks and with maximum GDF-5 (BMP-14) expression measured at 4 weeks.347 It is interesting to note that they also noted small cartilage and bone nodules localized in many of the tendons. In a similar study, Bolt et al used AdBMP-14 (GDF-5) gene therapy in a tendon laceration model to show that AdBMP-14 (GDF-5) treatment increased granulation tissue, cell proliferation, and early scar formation with a 70% increase in tensile strength at 2 weeks that was statistically significant.46 In a combination approach, Hoffmann et al used a gene therapy approach to transduce the MSC cell line (C3H10T1/2) with BMP-2 and a modified Smad8, a transcription regulator and downstream signaling molecule of the TGF-β/BMP receptor, and showed improved tendon repair by magnetic resonance imaging (MRI) in a partial Achilles defect model.182 Basile et al evaluated the improved healing potential of freeze-dried tendon allografts loaded with rAAV-GDF-5 compared with rAAV-LacZ reporter tendons and showed more rapid remodeling with the addition of GDF-5 gene therapy and statistically significantly improved flexion, indicating a decrease in adhesions in a mouse flexor tendon model at 14 and at 28 days.36 These findings suggest that GDF-5, GDF-6, GDF-7, and TGF-β1 enhance tendon repair in small-animal tendon laceration models using both an in vivo and an ex vivo approach. GDF-5, -6, and -7 are likely the primary candidates for modulating tendon healing in vivo with other TGF-β family members; PDGF and other growth factors may serve as secondary targets.
Ligament
Four major ligaments are responsible for stabilizing the articulation of the femur with the tibia at the knee joint: two intra-articular ligaments, ACL and PCL, and two extra-articular ligaments, the medial collateral ligament (MCL) and the lateral collateral ligament (LCL).366 The MCL is the primary restraint to valgus stress and is the most commonly injured ligament of the knee; however, most MCL injuries heal without requiring surgical intervention, albeit mechanically weaker than before injury, particularly if a gap is present between the torn ligament ends, or if injury occurred near the bone–ligament interface (for review130,298). The mechanism of healing for the MCL and all extra-articular ligaments is thought to be similar to that of tendon rupture, with a multistage process involving hemorrhage, inflammation, and early repair, followed by remodeling. The ACL is the most common intra-articular ligament injury and is responsible for anterior translation and rotational stability. In contrast to the MCL, ACL tears fail to repair without surgical intervention, with autograft ligament reconstruction serving as the most effective treatment (for review168). Given the fundamental differences in healing and in treatment strategies between extra-articular and intra-articular ligament injuries, the use of gene therapy will need to focus on augmenting repair or enhancing reconstruction, depending on the location and healing potential of the injured ligament.
Intra-articular ligaments heal poorly and require reconstruction to restore anterior-posterior translational and rotational stability, which is important in high-performance athletics and may prevent further knee injury and possibly long-term joint failure. It is estimated that as many as 100,000 ACL reconstructions are performed in the United States yearly.18 Although primary ACL repair by suture has been attempted,390 the ACL has poor de novo healing capacity, and surgical repair gave way to reconstruction with either autograft tissue, in particular hamstring or patellar tendon or allograft, typically Achilles or patellar tendon.110,169 All reconstruction procedures offer some disadvantages, including donor site morbidity in autografts, poor ligamentization, mismatch in biomechanical properties between native ACL and donor tissue, and possible disease transmission, limited availability, and immunogenicity of the allograft.429 In addition, in a recent meta-analysis of eight studies comparing allograft with autograft ACL reconstruction, autograft failure ranged from 0% to 8% in recent literature while allograft failure ranged from 0% to 12%, with one study reporting a 45% failure rate when failure was defined as any of the following: revision ACL reconstruction, traumatic graft rupture, or a combination of clinical instability findings (positive Lachman, pivot-shift, and >5 mm translation by arthrometer).58 Although technical error accounts for a majority of primary ACL reconstruction failures,141 failure of graft bony incorporation and “ligamentization” contributes to failure.11,198 The tendon–bone interface is considered the weakest site of the repair, especially early in the healing period,80,351 and patients are asked to refrain from pivoting activities for at least 3 to 9 months to prevent pull-out of the graft.231 Bone–patellar tendon–bone autografts have been a popular choice for ACL reconstruction because of the rigid osseous fixation and more rapid healing, but are known to have greater donor site morbidity, particularly in the older population.158,357 Ligamentization is the process of revitalization of the allograft or autograft with conversion from a more tendinous tissue to a ligament that contains increased type III collagen and proteoglycan, decreased collagen content and cross-linking, and increased fibroblast metabolic activity.12 The process of ligamentization progresses through avascular necrosis, revascularization with cell infiltration and repopulation, and collagen matrix remodeling, which takes months to years to complete.20,91 Technical considerations such as graft placement and tensioning are known to play a role in the remodeling process, making the relationship between anatomy and biomechanics and the biology of repair complex and requiring further consideration.290 Animal studies are beginning to explore the use of a scaffold to assist in primary ACL repair in an attempt to overcome some of the limitations associated with healing that led to the abandonment of repair for reconstruction.10 Despite our limited understanding of healing and failure associated with primary ACL repair, a gene therapy–based approach to improved healing may provide additional options for biologic repair of the native or reconstructed ligament.
Intra-articular Ligaments
The feasibility of gene therapy in ligament and tendon fibroblasts was first tested by Gerich et al, who showed that AdLacZ can be used to transduce cells in vitro derived from the ACL, PCL, MCL, patellar tendon, and semitendinosus tendon.140 Given the ability to transduce cells, Hildebrand et al used both an in vivo approach with AdLacZ injected into the ligament or site of injury, and ex vivo gene therapy with retrovirus-lacZ–treated ligament cells injected at the site of injury, and showed that in vivo gene therapy with AdLacZ was more effective than the ex vivo approach, with transgene expression still present in the ACL after 6 weeks.177 Similarly, Menetrey et al used an in vivo AdLacZ gene therapy approach and showed transgene expression as far as 42 days post injection with cells throughout the ACL expressing the transgene, while in the ex vivo approach with AdLacZ to both fibroblasts and myoblasts, injection of cells into the ACL led to expression of cells surrounding the ligament for 3 weeks and myotubes within the ligament expressing the transgene for approximately 6 weeks, respectively.291 In vivo gene therapy and to a lesser extent ex vivo gene therapy to the ACL resulted in transgene expression for as long as 6 weeks.
The next step was to use gene therapy to improve tendon–bone integration and/or ligamentization and repair in native and allograft tissue. To test the efficacy of gene therapy with BMP-2, a growth and differentiation factor associated with improved ossification and tendon–bone integration267 and with improved tendon–bone integration in ACL reconstruction with a semitendinosus graft, Martinek et al used AdBMP-2 gene therapy ex vivo to tendon grafts that were used for ACL reconstruction in rabbits.279 The AdBMP-2–treated autograft tendons showed improved histologic tendon–bone integration and statistically significant improvement in stiffness and load to failure, with failure occurring within the graft rather than at the tendon–bone interface, the failure mechanism seen in untreated grafts.279 To test the possible role of gene therapy in enhancing ligamentization, Li et al attempted to improve early revascularization and healing of the Achilles allograft in a rabbit ACL model through the transfer of PDGF-B, using an ex vivo gene therapy approach with MSCs, and showed improved early collagen type III deposition and increased cellularity at 3 weeks and expression of the transgene for longer than 12 weeks.247 However, no evidence was shown to support improved vascularity or improved strength of construct.247 In an attempt to improve primary repair of the ACL, scaffolds to bridge the ruptured ligament ends have begun to be used, as well as various approaches to enhance production or release of growth factors to improve healing.309,310 One approach is gene therapy ex vivo or in vivo delivered via the scaffold. Pascher et al used an in vitro model with cut ligament explants to test whether the combination of scaffold and gene transfer of AdTGF-β1 improved cell accumulation within the hydrogel and deposition of collagen type III with transgene expression.331 Subsequently, Steinert et al used an AdIGF-1–laden collagen hydrogel scaffold to improve primary repair of ACL in an in vitro ACL explant model. This study indicated that IGF-1 and AdIGF-1 can enhance cell accumulation and collagen deposition (as measured by 14C-proline) in hydrogels bridging ACL-injured explants in vitro.384 Although no gene therapy–based approach has been implemented in vivo, a platelet-rich plasma collagen scaffold–based approach has been used in animal models to show improved healing in primary ACL repair,123,307,308 suggesting a possible future for gene therapy in changing treatment approaches for ACL injuries.
Extra-articular Ligaments
Unlike the ACL, the MCL possesses intrinsic healing capacity, and the standard of care for grade I and grade II MCL injuries is nonsurgical with hinged knee bracing, early ROM and strengthening, and return to sports dependent on improvement of pain, but treatment for grade III remains controversial (for review298). MCL and extra-articular ligaments heal similarly to tendon with hemorrhage and inflammation followed by cellular infiltration, collagen deposition, and finally remodeling. Although lower-grade tears heal primarily with collagen I deposition, more severe tears are associated with collagen type III–rich scar tissue, which is biochemically and compositionally inferior to the uninjured ligament (for review434). MCL scar tissue in a rabbit injury model was measured to be 30% to 50% of normal values with increased creep, particularly early in repair.128,129,400,401 Biomechanical changes are associated with changes in composition, including changes in collagen I/collagen III ratios,38 increased collagen type V,319 and changes in water and proteoglycan content.129 High-grade MCL tears with bony avulsion or persistent laxity therefore require surgical intervention with primary repair or reconstruction with autograft or allograft tendon.298 Treatment of high-grade tears may benefit from biologic therapies such as gene therapy expression of growth factors to improve rate of repair and quality of repair tissue.
Gene therapy to extra-articular ligaments will focus on improving biologic repair and will target similar genes to tendon, particularly genes encoding growth and differentiation factors, which may be delivered locally using an in vivo or ex vivo approach. Early work proved that MCL ligament cells may be transduced in vitro to express reporter genes.140 Expression of transgenes within MCL cells using an AdLacZ in vivo and an AdLacZ or BAG-LacZ ex vivo approach indicated greater and longer-term expression using an in vivo approach and little difference between intact and injured ligaments.177,291 Gene therapy to extra-articular ligaments has been limited, with a study by Nakamura et al targeting inhibition of decorin, a small leucine-rich proteoglycan responsible for regulating collagen fibril diameter and binding to TGF-β and modulating its signaling. Nakamura et al used HVJ-liposome to deliver decorin antisense technology administered by direct injection, multiple direct injections, and femoral artery intra-arterial injection to downregulate decorin expression and observed improved transfection by intra-arterial injection with decorin antisense therapy, resulting in larger collagen fibrils and an 85% increase in scar strength, which was significantly stronger than in the control.313 Because of the similarity between tendon and ligament, much of the ongoing gene therapy work for tendon may be applicable to extra-articular ligament healing.
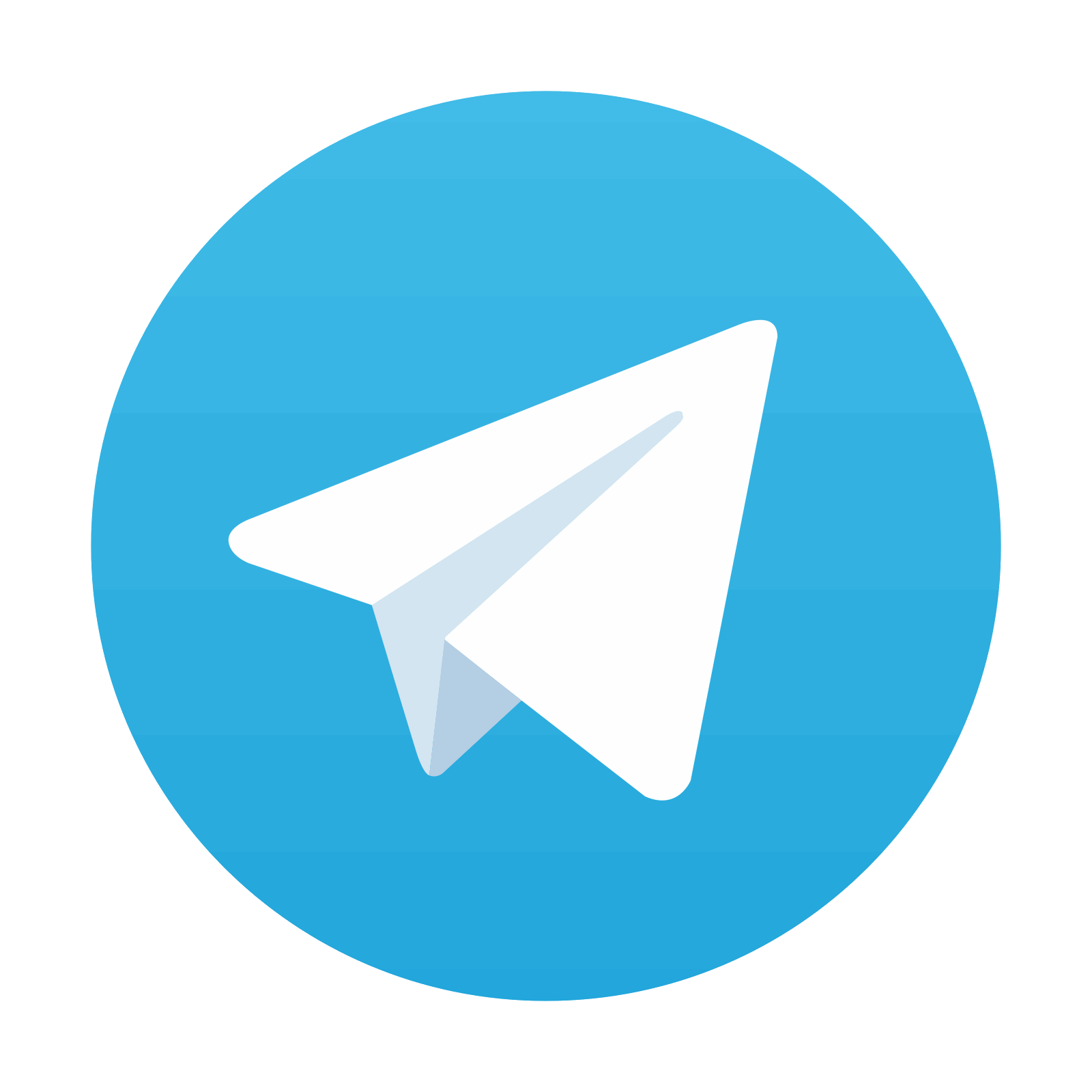
Stay updated, free articles. Join our Telegram channel

Full access? Get Clinical Tree
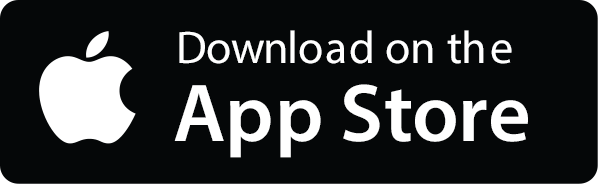
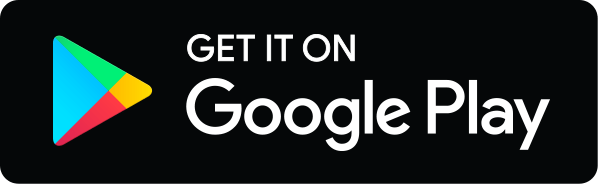