6 Gait assessment of neurological disorders
Gait assessment in cerebral palsy
Definition, causes and prevalence
Cerebral palsy is now defined as a group of permanent disorders of the development of movement and posture which can be attributed to brain damage to the fetus or infant (Rosenbaum et al., 2007). The condition is different from other forms of brain damage such as stroke or traumatic brain injury because it happens while the brain is still developing and this affects the subsequent neurological and musculoskeletal development of the child. The motor disorders of cerebral palsy are often accompanied by disturbances of sensation, perception, cognition, communication and behaviour, and by epilepsy. Cerebral palsy is the commonest cause of physical disability affecting children in the developed world with a prevalence of around 2 cases for every 1000 live births (Stanley et al., 2000). Figures for other parts of the world are difficult to ascertain but it is generally assumed to be at least as prevalent.
Classification
Gross motor function classification system
The principal means of classifying children with cerebral palsy is with the Gross Motor Function Classification System GMFCS (Palisano et al., 1997 and 2000). This is, essentially a five-point scale reflecting the severity of the condition as it affects motor function. There are different definitions for different age groups but that for 6–12 year olds is the most relevant to gait analysts (Table 6.1). It can be seen that most children who are suitable for gait analysis will be of levels I–III. More recently descriptors for 12–18 year olds have been produced (Palisano et al., 2008). These are similar to those in Table 6.1 but allow for some deterioration in motor ability that occurs in late childhood. While gait analysis is most often used for children with cerebral palsy it is worth remembering that cerebral palsy is a life-long condition, with between 25% and 50% of adults reporting further deterioration in walking ability in early adulthood (Day et al., 2007; Jahnsen et al., 2004; Murphy et al., 1995).
Table 6.1 Gross Motor Function Classification System for children aged 6–12 years
Level I | Children walk indoors and outdoors and climb stairs without limitation. Children perform gross motor skills including running and jumping, but speed, balance and coordination are impaired |
Level II | Children walk indoors and outdoors and climb stairs holding onto a railing but experience limitations walking on uneven surfaces and inclines and walking in crowds or confined spaces and with long distances |
Level III | Children walk indoors and outdoors on a level surface with an assistive mobility device and may climb stairs holding onto a railing. Children may use a wheelchair for mobility when travelling for long distances or outdoors over uneven terrain. |
Level IV | Children use methods of mobility that usually require adult assistance. They may continue to walk for short distances with physical assistance at home but rely on wheeled mobility (pushed by adult or operate a powered chair) outdoors, at school and in the community |
Level V | Physical impairment restricts voluntary control of movement and the ability to maintain anti-gravity head and trunk posture. All areas of motor function are limited. Children have no means of independent mobility and are transported by adults |
Classification by gait pattern
There have been several attempts to classify cerebral palsy on the basis of the gait pattern (Dobson et al., 2007). Some confusion has arisen because several authors have chosen to re-define terms used by previous authors and it is not always clear which classification system is being referred to by which term. Two classifications for spastic hemiplegic gait have been proposed (Hullin et al., 1996; Winters et al., 1987) of which Winters et al. is more commonly used. This is described in Figure 6.1 and Table 6.2. There is some confusion in how this is applied clinically. The original paper refers exclusively to the sagittal plane and is based purely on the gait pattern. It is not at all uncommon to hear clinicians incorporating consideration of the transverse plane (particularly at the hip) and inferences about the nature of the underlying pathology (rather than just the gait pattern) in classifying children. Agreement between clinicians in applying this classification is substantial (Dobson et al., 2006). A more recent population-based study suggested that groups I, II and IV are far more common than group III.
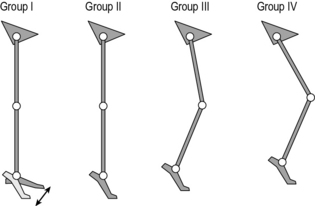
Fig. 6.1 • Common gait patterns in hemiplegic cerebral palsy
(reproduced with permission and copyright © of the British Editorial Society of Bone and Joint Surgery. (Rodda et al., 2004).
Table 6.2 Classification of gait patterns in hemiplegia*
Group I | Ankle equinus in the swing phase of gait due to underactivity of the ankle dorsiflexors in relation to the plantarflexors |
Group II | Plantarflexion throughout stance and swing from either static or dynamic contracture of the triceps surae. Knee is often forced into slight hyperextension in middle or late stance |
Group III | Findings of type II with reduced range of knee flexion/extension. Reference is now common to sub-types IIIa: reduced knee extension during stance, and IIIb: hyperextension in stance and reduced flexion in swing |
Group IV | Findings of type III with involvement of the hip musculature† |
* Summary by Thompson et al. (2002) of original by Winters et al. (1987).
† In the original paper, in which measurements were restricted to the sagittal plane, this was attributed to flexor and adductor involvement. A three-dimensional analysis would almost certainly have included increased internal rotation
Classifications of gait patterns in spastic diplegia are less clear cut. One example is the classification by Rodda et al. (2004) in which the whole gait pattern is described (Fig. 6.2 and Table 6.3). There is particular confusion with regard to a number of terms that different people have chosen to define differently. Sutherland and Davids (1993), for example defined ‘jump’ as referring to a pattern in which the knee is flexed in early stance but extends rapidly in a pattern reminiscent of jumping. Rodda et al. (2004) used the same term to refer to a posture of flexed knee and plantarflexed ankle in late stance. ‘Crouch’ gait is another term that has been defined differently by a range of authors. Many studies simply use the term to refer to a gait pattern exhibiting knee flexion of no less than a certain value (30° is commonly used) throughout stance. Gage et al. (2009) used a kinetic definition for patterns in which the internal knee moment is extensor throughout stance, whereas Rodda et al. (2004) required ankle dorsiflexon as well as knee flexion.
Table 6.3 Common gait patterns in spastic diplegic cerebral palsy (Rodda et al., 2004)
Group I | The ankle is in equinus. The knee extends fully or goes into mild recurvatum. The hip extends fully and the pelvis is within the normal range or tilted anteriorly |
Group II | The ankle is in equinus, particularly in late stance. The knee and hip are excessively flexed in early stance and then extend to a variable degree in late stance, but never reach full extension. The pelvis is either within the normal range or tilted anteriorly |
Group III | The ankle has a normal range but the knee and hip are excessively flexed throughout stance. The pelvis is normal or tilted anteriorly |
Group IV | The ankle is excessively dorsiflexed throughout stance and the knee and hip are excessively flexed. The pelvis is in the normal range or tilted posteriorly |
Classification by gait pattern does not give the whole picture. The two most commonly used schemes are based on sagittal plane data only (Rodda et al., 2004; Winters et al., 1987). Winters et al. proposed their classification system for people who had had no previous orthopaedic intervention and it is not clear how much value the scheme has for people who have had surgery. Rodda et al. did not discuss how surgery, or other interventions, would affect classification by gait pattern. There is considerable variability within grades and some people clearly have patterns on the boundary between one grade and the next. Indeed two studies which include data that can be used to infer whether natural groupings exist (Dobson, 2007; Rozumalski and Schwartz, 2009) would suggest, on the contrary, that gait patterns vary continuously across a multi-dimensional spectrum and groupings will only ever be a rather gross guide to the general pattern of movement. They can, however, still be useful in giving an overall impression of the person’s gait pattern particularly if full instrumented gait analysis is not available. A full gait analysis is still essential if we want to be specific about how a person is walking and what is causing them to do so in a particular manner.
Impairments
Spasticity
The term ‘spasticity’ means different things to different people. Most clinicians working with people with cerebral palsy1 tend to use it to refer to a very specific ‘velocity-dependent increase in tonic stretch reflexes, with exaggerated tendon jerks resulting from hyperexcitability of the stretch reflex’ (Lance, 1980). In other words if a tendon is stretched rapidly then its muscle will become active. Depending on the severity of the spasticity this might be for a short period and the muscle may thus show phasic, but inappropriate activity throughout the gait cycle, or for a longer period in which case the muscle may be inappropriately active throughout the gait cycle. This stretch reflex is present in all of us but in most people is suppressed by signals originating in the brain and passing down the spinal column (descending control). In people with cerebral palsy the brain damage reduces the capacity to send such signals and the reflexes are effectively out of control. Thus, although it is common to hear reference to ‘spastic muscles’, spasticity is really a property of the nervous system.
In cerebral palsy spasticity commonly affects some muscles more than others. In particular the bi- and multiarticular muscles appear to be susceptible (Gage et al., 2009). Thus spasticity is commonly found in the gastrocnemius, hamstrings, rectus femoris and psoas muscles. In more severely affected children, spasticity of the monoarticular hip adductors is a particular issue.
Muscle contracture
The mechanisms by which contractures develop are not fully understood. In relation to children with cerebral palsy it is quite common to hear contractures talked about as a failure of normal growth, where the bone keeps on growing but the muscle does not. This cannot be the full picture, as some children, particularly those with hemiplegia, can develop contractures over a short period of time which are too severe to be explained simply by failure of growth. Contractures are also common in many adults who have spasticity (in conditions such as stroke). Another common belief is that contractures are a result of immobilisation, which is supported by some early clinical and animal work suggesting that this reduces the length of the muscle fibres (Shortland et al., 2002). Again though, there are many other conditions in which immobilisation of muscles is much more complete than in cerebral palsy, but such severe contractures do not develop. More recent work suggests that a reduction in muscle belly length through shortening of the aponeuroses is much more significant than any reduction in fibre length (Shortland et al., 2002), but no mechanism has been proposed for this. In summary, the development of contractures is probably related to failure of growth, immobilisation and the consequences of spasticity but the relative contributions of these factors remain unknown.
Weakness
It is only relatively recently that the effect of muscle weakness on the gait of people with cerebral palsy has been fully acknowledged. Wiley and Damiano (1998) surveyed the strength of children with cerebral palsy and demonstrated that muscle weakness is also important with the gluteal muscles with the plantarflexors also being particularly weak with respect to those of age-matched able-bodied children. Muscle weakness can arise either because of the anatomy and physiology of the muscles or through reduced neural activity to stimulate contraction. Despite cerebral palsy being an essentially neurological problem there have been few investigations to establish the relative proportions of the muscular and neurological components of weakness.
The weakness has generally been attributed to a muscle’s reduced physiological cross-sectional area attributed to short muscle belly length and may therefore be strongly related to the development of contracture in both spastic hemiplegia and diplegia (Elder et al., 2003; Fry et al., 2007). The clinical picture does not always agree with this, with some muscles appearing to be quite weak without evidence of contracture. It is also known, however, that there is more extra-cellular material of lower quality than in normal muscle. Recent evidence suggests that these changes are quite different from those that arise simply through either chronic stimulation or disuse (Foran et al., 2005) but, as with the development of contracture, the underlying mechanisms are not understood. During movement there is a further factor in that contractures or specific walking patterns may result in muscle fibres functioning away from their optimal length to generate contractile force. The clinical picture appears to be that the muscles get weaker with age with respect to those of children without cerebral palsy. A particular issue arises in late childhood and early adolescent when weight increases rapidly and relative weakness becomes significant.
Clinical management
Spasticity management
The focus of management in early childhood is generally on trying to reduce spasticity and a range of options are available. Although there are some anecdotal reports of responses to stem cell implants there is little scientific evidence so far that anything can be done to repair the original brain damage. Botulinum toxin is a chemical that disrupts the neuromuscular junction and thus prevents the neural input to a muscle activating it. If injected into the muscle it is selectively absorbed by these junctions and is an excellent way of suppressing activity in specific muscles. The direct effect of the toxin wears off over a period of between 3 and 9 months (Eames et al., 1999). This can be useful as it is possible to test the effects of injections without the risk of doing permanent harm but does mean that, if successful, injections need to be repeated. In children with cerebral palsy who can walk, the gastrocnemius muscles are the most commonly injected (Baker et al., 2002; Eames et al., 1999). In severe spasticity it may be more effective simply to release specific muscles surgically rather than perform repeat injections.
Strengthening
Now that the importance of weakness in cerebral palsy has been acknowledged there has been considerable research into physiotherapy programmes to actively strengthen muscles (Damiano and Abel, 1998; Damiano et al., 1995, 2002; Dodd and Taylor, 2005; Dodd et al., 2002, 2003). These generally used the principles of progressive resistive strength training, which have now been demonstrated to result in increases in muscle strength in children with cerebral palsy of up to 25% (which is consistent with their use in many other conditions). Such programmes are now being used more and more routinely.
Gait analysis
Data capture
Most clinical services focus on obtaining good-quality kinematic and kinetic data based on the measured positions of retro-reflective markers using techniques documented elsewhere in this book. The conventional gait model (CGM) (Baker and Rodda, 2003; Davis et al., 1991; Kadaba et al., 1990; Ounpuu et al., 1996) is by far the best documented and most common approach (see Chapter 4). Other models are used but it is questionable whether they have yet been sufficiently well validated in clinical practice to satisfy the strict demands of clinical governance. Many centres will also capture EMG data either concurrently with full kinematic data or with a reduced marker set.
Clinical examination
In addition to the gait analysis, a full clinical examination is also completed. The range of movement of various joints is assessed which helps identify muscle and joint contractures. Muscle testing is generally carried out, grading muscles on the conventional five-point scale (Kendall and Kendall, 1949) and this is often accompanied by a simple assessment of the degree of selectivity with which the child can control muscle activation. The Tardieu test (Boyd and Graham, 1999) is used to measure spasticity and the modified Ashworth scale (Bohannon and Smith, 1987), while often referred to as a measure of spasticity, should probably be regarded as a measure of resting tone. Measures of bony malalignment such as femoral anteversion and tibial torsion are also recorded. An example of the results of such a clinical examination are included in Figure 6.3. The results of the clinical examination give additional information which aids the process of interpreting the gait analysis data to elucidate exactly which impairments are most affecting the gait pattern.
Interpretation
Orientation
The first thing the clinician must do is obtain a general impression of the child and how they are walking. This will require a knowledge of the diagnosis (assumed here to be cerebral palsy), motor type, GMFCS classification and topographical distribution. Scales indicating the child’s general level of functions such as the Functional Assessment Questionnaire (Novacheck et al., 2000) or the Functional Mobility Scale (Harvey et al., 2007) may also be useful. It is also important to have an overview of the child’s medical and surgical history and of the precise reason they have been referred for gait analysis. The final part of orientation is to look at the video to get an overall impression of the gait pattern. During the assessment the child or family should be asked whether the walking pattern adopted during the analysis is representative of the way they usually walk.
Mark-up
The next stage is to look at the data and identify gait features. These are regions of the traces that are different from reference data from a population that has no neuromusculoskeletal impairments. Many gait analysts do this in their head but it can be extremely useful to actually annotate the graphs to mark-up these features (Figure 6.4), using the symbols that are listed in Table 6.4.
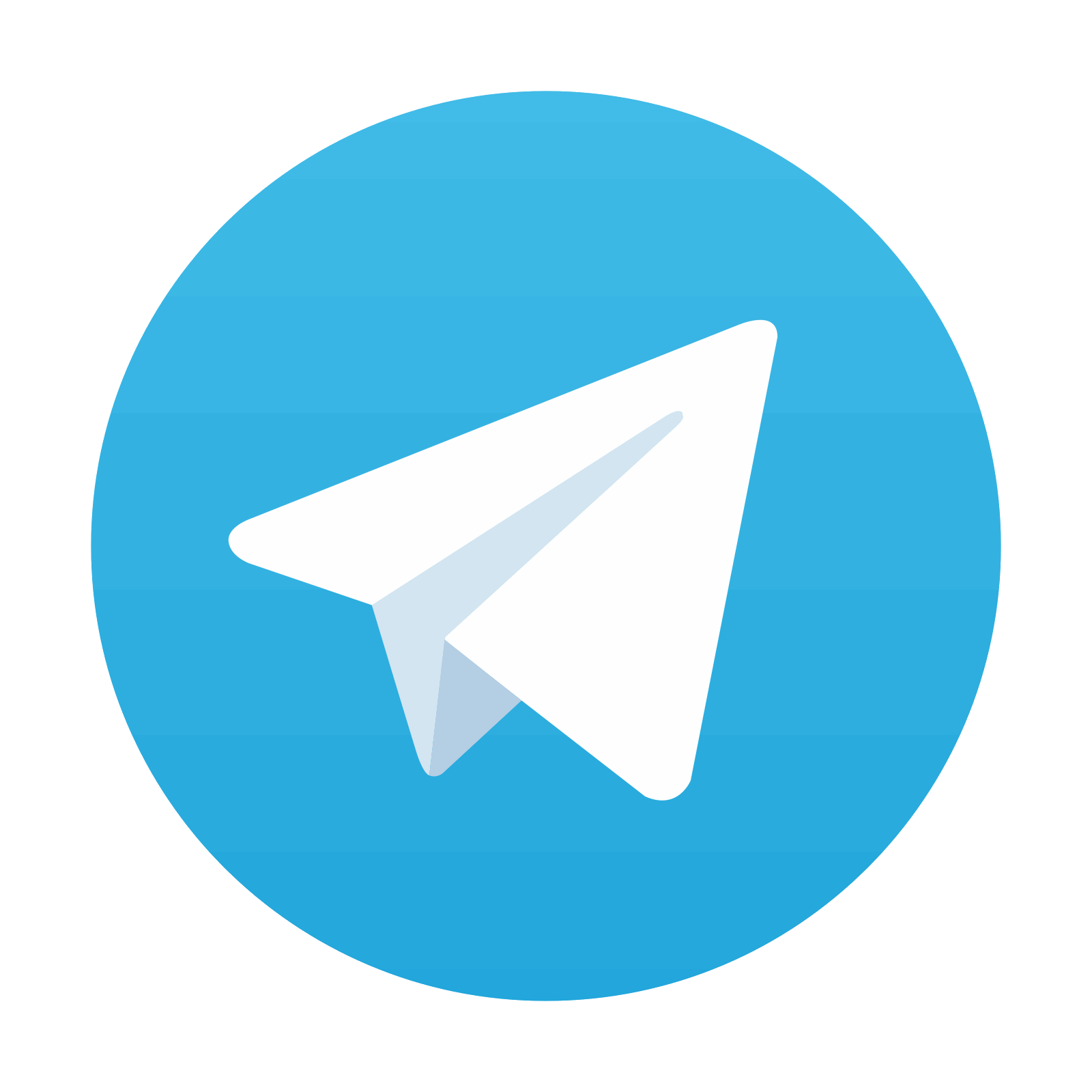
Stay updated, free articles. Join our Telegram channel

Full access? Get Clinical Tree
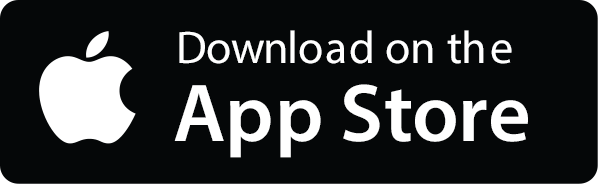
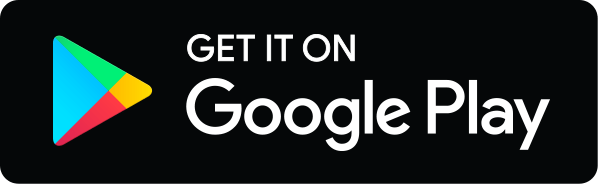
