Chapter 10 From the CORE to the Floor—Interrelationships*
After studying this chapter, the reader will be able to do the following:
During sport activity, the lower extremity moves in a myriad of motion planes with linkage to the body’s core. Speed variations, changes in directions, upper extremity requirements, and opposing player disturbances all challenge the athlete’s postural stability. Restoration of dysfunction in the athlete must be viewed from a total kinetic chain, sport-specific, and core-to-the-floor perspective. Recovery from injury depends on reestablishing mobility and neuromuscular control, often with underlying factors of less than optimal structural alignment. In the spontaneous environment of sport, the body’s core provides muscular stability as the foot and ankle serve as the interface to the grass, court, or track surface. The foot and ankle provide shock absorption, contact balance, and spontaneous propulsion in all motion planes. The body’s core is interdependent with the foot and ankle because it benefits from mobility and stability characteristics from which it can absorb and generate forces required during running and jumping in sport.1 Often in sport rehabilitation, a joint- or muscle-specific treatment approach is taken. Practitioners can miss total kinetic chain interdependencies and lead the athlete through suboptimal rehabilitation. A joint- or muscle-specific treatment focus may place added overuse stress to the area that is painful. A total kinetic chain approach, however, with attention given from above and below the injured area, assists mechanoreceptor stimulation and imparts functional mobility and stability feed to the injured site.
INTERRELATIONSHIPS OF THE CORE, KNEE, ANKLE, AND FOOT
As the athlete moves, responds, accelerates, and decelerates, dependency exists between the stability and power offered by the body’s core and the mobility and kinesthetic directives to the kinetic chain provided by the foot and ankle (Figure 10-1).2 Kibler3 describes the significant role the trunk and scapula play in the tennis athlete. His premise for upper extremity overuse pathophysiology is that altered proximal function places increased burden on the distal joint. Kibler3 describes the kinetic chain linkage in the tennis stroke that allows ground reaction forces and large leg and trunk muscles to generate forces that are summated and passed distally with contributions from each successive link: legs, trunk, shoulder, elbow, and wrist.
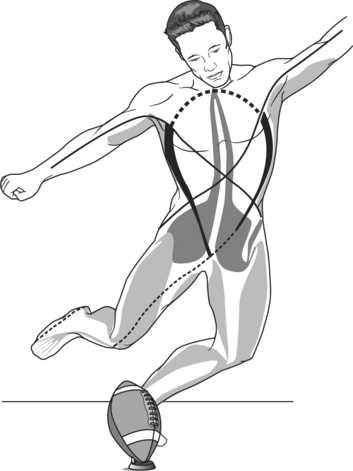
Figure 10-1 From the trunk to the foot, interdependencies exist as the kinetic chain acts and reacts in sport.
Evidence-Based Clinical Application: Core Trunk Influence to the Upper Extremity
Kibler3 writes that during the tennis stroke 51% of the total kinetic energy and 54% of the total force are developed in the leg-hip-trunk linkage. The large forces developed in the proximal links are funneled through the shoulder to the hand and racquet by soft tissue constraint systems of the shoulder. The shoulder relies on its position as a link in the kinetic chain and depends on the generation and transfer of forces from the lower extremity and core to the hand.
Similarly, jumping and jump landing require summated acceleration and deceleration forces between the foot and the core. Nicholas and Marino4 describe this kinetic chain linkage enabling stronger muscles to support weaker movements. They propose that weakness of one segment can disrupt the entire kinetic chain and eventually promote weakness that can migrate throughout the chain. They examined the relationship between proximal thigh strength in athletes with lower extremity pain patterns and related deficiencies to ankle pain.
Similarly, Beckman and Buchanan5 studied the interrelationship between hip muscle recruitment and ankle instability. They describe a neuromuscular response of lowered hip muscle activation thresholds of the gluteus medius in response to a protective steady state ability to respond to ankle inversion.
A strong core aids muscle stabilization, balance, coordination, and kinesthesia. Nicholas and Marino4 reported on the thigh strength of 134 athletes with residual chronic pain patterns after their injury was thought to have been healed. The authors report that their foot and ankle–injured athletes demonstrated significant weakness of the hip abductors and adductors when compared with the uninvolved side through isokinetic testing.
Bullock-Saxton et al6 found alterations in electromyographic activity of proximal core muscles in subjects who had sustained a significant ankle sprain. Gribble et al,7 when studying the effect of chronic ankle instability on postural control, proposed that disruptions to a joint may lead to altered neural activity and compensatory muscle recruitment at neighboring joints and corresponding movement patterns. The authors state “the injured athlete may be able to complete a gross motor task, but the method of completion may be altered and less than optimal and/or efficient creating the potential threat of reinjury.”7
Postural stability is defined as the ability to control the body’s center of mass within specific boundaries (stability limits).8 It depends on mobility, stability, and kinesthesia from the core to the floor. Consider jump landing with poor core stability resulting in less than optimal control of postural orientation on landing. Body sway and postural instability require the foot to act as a balance adapter and adjuster. If end range of motion is required, the foot may load beyond its physiological limits and become injured. Hewett et al9 write that an athlete is at risk for injury if the lower extremity is not properly aligned or if the foot is in an unusual position on landing from a jump. The avoidance of excessive valgus or varus forces at the knee on jump landing minimizes the risk of knee injury.10 A total kinetic chain, core-to-the-floor thinking process enables the practitioner to think beyond the area of localized pain to the multifaceted potential causes of mechanical breakdown. When considering patellofemoral joint pain, an understanding of kinetic chain influences is imperative. Post et al11 describe patellofemoral pathogenesis in dynamic foot-to-the hip function terms: “bony malalignment, joint geometry, soft tissue restraint, neuromuscular control and functional demands combine to produce symptoms as a result of abnormally directed loads that exceed the physiologic threshold of the tissues” (Figure 10-2).11
Weakness of hip abductors, extensors, and external rotators is related to the body’s ability to control frontal and transverse plane motion at the knee (Figure 10-3).12 Targeting core hip muscles through strength facilitation has been shown to alleviate patellofemoral pain and change knee mechanics.12,13
Mascal et al12 reports on two patellofemoral joint patients with weakness of hip abductors, extensors, and external rotators as demonstrated by hand-held dynometry testing. A 14-week period of training included a progression from non–weight-bearing exercise, weight-bearing exercise, to functional training. Posttesting demonstrated a greater than 50% increase in gluteus medius force production in a greater than 90% gluteus maximus force production in these cases with objective kinematic improvement in step-down tasks, as well as a significant reduction in visual analog scale pain rating.
Fulkerson14 correlates hip weakness to anterior cruciate ligament injury and patellofemoral pain patterns. He particularly identifies hip external rotators as key stabilizers to dampen the functional internal rotation of the hip during landing mechanics. McConnell15 describes exercise strategies in the treatment of patellofemoral pain with a focus on the gluteus medius as being critical to normal mechanics of the patellofemoral joint. Gluteus medius posterior fiber training aims to decrease excessive closed chain hip internal rotation and its resultant knee valgus vector force.
Ireland et al16 found hip weakness related to patellofemoral joint pain. When comparing patellofemoral pain subjects with normals, a 26% decrease in hip abduction strength and a 36% decrease in external rotation strength were found in the group with patellofemoral joint dysfunction. Leetun et al17 also found a relationship in pain patterns related to hip abduction and hip external rotation weakness. Forty-one injuries were studied in 140 athletes, and core stability measures were found as primary risk factors for injury in basketball and cross-country college athletes.
Concomitant with hip muscle stability, the abdominals provide a critical role in core stabilization for kinetic chain activity. Abdominal, paraspinal, and pelvic muscles integrate upper and lower extremity motions. Core trunk muscles act as synergistic stabilizers4,18,19 as they act as a hub where trunk and ground reactions converge and are modulated.20 Hodges and Richardson21 found that the transverse abdominis is the first muscle that is active during movement of the lower limb following contralateral weight shifting. They provide evidence that the central nervous system initiates contraction of abdominal muscles in a feed-forward manner in advance of lower limb motion, hence the interdependency and linkage between the trunk and lower limb in the control of postural stability.
ABNORMAL MECHANICS OF THE LOWER EXTREMITY LINKAGE SYSTEM
The lower extremity is designed as a locomotor unit providing shock absorption, stability, propulsion, and energy conservation during sport activity.22 The accumulative amount of force generation and dissipation that the kinetic chain responds to is enormous. During running and jumping sports, the lower kinetic chain moves in various forms of airborne-to-landing impacts thousands of times each training and competition session.23 Running 5 km requires an estimated 2500 foot fall with landing forces described as high as 8 times body weight.1 Jump landing forces are reported to be as high as 20 times body weight.24 Simple stairway descent requires shock absorption of as much as 3.7 times body weight.4 Abnormal loading and landing mechanics, when performed repetitively, can place microstress and strain throughout the lower extremity. Inflexibility, hypermobility, neuromuscular weakness, altered kinesthesia, and structural malalignment contribute individually or in combination to abnormal running and jumping mechanics.
Foot and Ankle Influences to the Kinetic Chain (Bottom Up)
Mobility and structure of the foot and ankle greatly influence proximal segments of the lower extremity during shock absorption and propulsion requirements.25 The foot and ankle act as the first unloaders to ground reaction forces protecting the entire kinetic chain from shock impact. Subtalar and midtarsal mobility enable triplanar pronation and supination, providing the kinetic chain with shock absorption and torque conversion of the femoral and tibial rotation at the foot–ground interface.26 The foot is uniquely designed with 26 bones and more than 30 articulations, each with 6 degrees of freedom of movement to serve as the body’s interface with the ground.25 During jump landing the foot acts to decrease the downward velocity of the center of mass, thereby attenuating impact force.2 The foot responds to ground reaction forces when jump landing from a plantarflexed position and absorbs landing forces by dorsiflexing at the ankle and pronating at the foot. Bobbert describes the angular velocity of foot dorsiflexion during jump landing as ranging from 578 degrees per second to 1025 degrees per second with varying heights of 20 cm and 60 cm, respectively.27 Valiant and Cavanagh28 reported that basketball players who land flat-footed versus the typical toe heel pattern had increased vertical impact forces and peak landing pressures on landing.
Dananberg and Guiliano30 write that the rocker effect of ankle dorsiflexion, calcaneus bone curvature, and hallux extension while walking and running serve to absorb landing forces and propel the body forward in the sagittal plane (Figure 10-4). Motion restrictions with sagittal plane ankle dorsiflexion or hallux extension, or both, will alter gait mechanics, shock absorption, and propulsion. Restoration of restricted foot and ankle motion in the athlete relates to improved shock absorption and propulsion throughout the kinetic chain. Functional retraining through dynamic mobilization and weight-bearing exercise purportedly stimulates a neuromuscular response.31–33 Mulligan33 writes that when motion is gained in weight bearing, it is better retained in function than non–weight-bearing techniques (Figures 10-5 through 10-10). Kaufman et al34 describe insufficient ankle dorsiflexion as a primary risk factor for injury among 449 Navy Seal candidates. They relate the 149 injuries to a prescreening assessment profile of flexibility and foot mechanics when following the Navy Seal candidates over 25 weeks of intensive training. Ankle dorsiflexion restriction, abnormal pronation, and supination foot mechanics, as well as hypermobility of ankle inversion, were all found to be risk factors for this Navy Seal group (Figures 10-11 through 10-12).
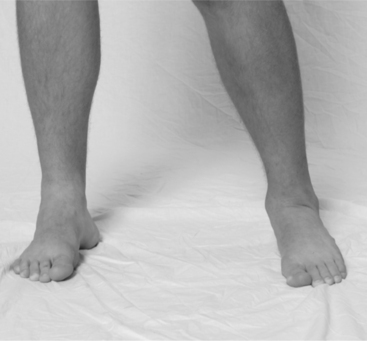
Figure 10-10 Supination with external rotation right leg. Pronation with internal rotation left leg.
Evidence-Based Clinical Application: Sensory Influences of the Foot on the Lower Kinetic Chain
Sensory deprivation of the feet has been shown to significantly alter lower extremity kinetic chain response and postural reflexes. O’Connell29 reported on the postural reflex response of four subjects ejected from a swing under the conditions of controlled jumps, blindfolded jumps, and anesthetized feet (20 minutes with feet suspended in ice water). During the controlled drops each subject landed and achieved an effortless erect posture. When visually deprived (subject blindfolded), three of the subjects responded with greater knee flexion than during the controlled ejections. However, with subjects blindfolded and feet chilled in ice water, no subject was able to regain erect posture as their postural reflex and postural stability was completely impaired. The necessity for feedback from sensory organs of the feet to coordinate a positive supporting reaction of the kinetic chain was demonstrated.
Evidence-Based Clinical Application: Static Stretching versus Dynamic Functional Stretching
Improving gastrosoleus flexibility is particularly difficult due to the shortening influence of the muscle tendon unit during sleeping hours when the foot is in end range of plantar flexion. Youdas et al35 reported on the effects of a 6-week program of static calf musculature stretching with 101 adults. A 6-week once-per-day static stretching regimen for up to 2 minutes was not sufficient to increase active dorsiflexion range of motion in this group.
Functional gastrosoleus stretching exercises include dynamic movement and neuromuscular training into the direction of desired motion. Ryerson and Levit31 describe movement reeducation training to stimulate firing patterns of the foot and ankle with weight shift and foot posturing strategies for neuromuscular reeducation.
Closed kinetic chain pronation defined as calcaneal eversion, talar adduction, and talar plantar flexion links to tibial rotation and knee flexion and thereby directly influences the knee and patellofemoral joints.26 Hreljac et al36 write that foot pronation during stance provides dissipation of stress protecting the lower extremity by attenuating high-impact forces. When comparing injury-free runners with runners with a history of lower extremity pain patterns, they found that the injury-free group pronated more rapidly during the stance phase of running. They postulated that this may have assured foot stability before push off. Excessive, inadequate, or mistimed pronation and supination relate to deficiency in the lower extremities’ ability to absorb shock, provide ground reaction stability, and propel the lower extremity forward. The repetitive nature of abnormal foot mechanics can cascade into a myriad of lower extremity injury patterns including iliotibial band syndrome, patellofemoral dysfunction, stress fracture, ankle instability, posterior tibialis tendon dysfunction, shin splints, Achilles’ tendonitis, and plantar fasciitis.37–47 Williams and McClay48 studied injury history patterns and lower extremity mechanics in a group of 20 runners classified as high-arched pes cavus feet and 20 runners with low-arched planus feet (Figure 10-13). Low-arched runners had significantly more medial, soft tissue, and knee injuries, while high-arched pes cavus foot types sustained more lateral, bony, and foot injuries. The authors describe that the injury patterns correlated planus foot types with greater rearfoot motion and higher velocities stressing more medial and soft tissue structures. Conversely, high-arched cavus foot types run with stiffer gait patterns and higher vertical load rates, sustaining more shock-related problems, such as stress fractures.
During running, maximum pronation occurs at approximately 45% of the total stance time when measured by rearfoot calcaneal eversion angle.49 Similarly, maximum knee flexion occurs also at approximately 45% of stance, followed by kinetic chain propulsion with knee extension. Subtalar joint pronation is linked to tibial internal rotation and knee flexion.11 The complementary relationship between knee flexion and pronation provides kinetic chain loading and shock absorption. Knee extension and concomitant lower leg external rotation and supination provides propulsion stability. Buchbinder et al50 theorized that excessive pronation causes prolonged lower extremity internal rotation in late stance phase when it would normally undergo external rotation. Disruption of this normal timing relationship may result in tissue overload and injury patterns throughout the lower kinetic chain.1 The relationship of excessive pronation to patellofemoral mechanics is well accepted.51 McClay and Manal52 describe the increased valgus position of the tibial femoral joint related to excessive foot pronation. Powers et al13 report on an alignment profile of excessive foot pronation; excessive heel angle; and an associated increased, laterally directed resultant quadriceps and patellar tendon forces in the frontal plane. They describe the lateral resultant force production with increased contact forces and contact pressures on the lateral aspect of the patellofemoral joint.
The cavovarus-type foot strikes the ground in an inverted position, and rearfoot eversion motion is typically limited, diminishing the shock-absorbing capacity of the subtalar joint. This excessively supinating foot type commonly presents with a plantarflexed first metatarsal. Laterally directed overload can occur with resultant ankle instability, Jones fracture of the fifth metatarsal, metatarsalgia, peroneal tendon pathologies, and sesamoiditis.43 Linkage to excessive external rotation of the tibia may result in varus strain at the knee joint and iliotibial band friction syndrome.53
Core Influences to the Kinetic Chain (Top Down)
Proximal core weakness at the trunk, pelvis, and hip has significant ramifications for the athlete’s ability for shock absorption and postural stability. The gluteus medius, upper gluteus maximus, and posterior tensor fascia lata stabilize the pelvis in the frontal plane during rapid transfer of the body weight onto the loading leg when running (Figure 10-14).22 These muscles provide an active lateral stabilization of the pelvis over the hip. Muscle stabilization is required because the base of the body vector shifts to the supporting foot while controlling the center of gravity. Weight transfer while running produces a large medial torque at the hip that causes the unsupported side of the pelvis to drop, hence the stabilization requirement of hip muscles firing at approximately 35% of maximal muscle tension even with simple walking. Internal rotation of the limb in the transverse plane also must be controlled during the loading response. The lower extremity is neurophysiologically wired for concomitant knee flexion, tibial and femoral internal rotation, and foot pronation when loading from airborne to landing postures. Muscular stability provides boundary to this knee flexion, hip internal rotation, and hip adduction pattern. Excessive internal rotation and adduction of the femur lead to potentially injurious transverse and frontal plane motions. Hip internal rotation is decelerated by the external rotational effects of the gluteus maximus muscle action.
Powers et al13 describe altered lower extremity kinematics of the femur and tibia in the transverse and frontal planes and its effects on patellofemoral joint mechanics. They describe bottom up and top down influences to the patellofemoral joint and its movement mechanics. Excessive frontal plane valgus and transverse plane tibial and/or femoral rotation increase the Q-angle as the patella displaces medially relative to anterior superior iliac spine. In contrast, misbalance and altered mechanics could result in excessive knee varus load placed in the frontal plane. Excessive knee varus or valgus loading necessitates a kinetic chain response to control the center of gravity in space. Therefore excessive ankle, knee, or hip muscle activation may be required, creating a potentially overstressed joint environment. Geraci54 describes a relationship between muscle weakness patterns of the hip and lower extremity injury patterns. Muscle imbalance patterns are described in which the gluteus medius, maximus, and abdominals can have neural reflexive inhibition resulting in functional weakness. In contrast, the antagonist hip adductors, hip flexors, and erector spinae are related to tendencies for shortening and tightening restrictions. Janda55 describes neuromuscular imbalances in which pain and dysfunction in a motion segment can initiate weakness in phasic muscles and tightness in postural muscles. Sale56 describes a concept of neural adaptation in which focused strength facilitation exercises may cause changes within the neuromuscular system that enable an individual to better coordinate the activation of muscle groups, thereby affecting a greater net force even in the absence within the muscles themselves. Facilitation techniques involve stretching the antagonist of the inhibited weak gluteus medius and gluteus maximus (Figure 10-15). Geraci54 describes a neurological release to the inhibition through functional retraining. The recruitment of mechanoreceptor input through functional closed kinetic chain exercise patterns includes patterns of postural muscle flexibility and phasic muscle dynamic strength recruitment (Figure 10-16).
STRUCTURAL MALALIGNMENT OF THE HIP, KNEE, AND TIBIA
Faulty mechanics during running, jump propulsion, and landing may be predisposed by skeletal malalignment. Post et al11 write that normal bony alignment allows weight transfer to be balanced in a manner tolerated by the core and lower extremities. Conversely, malalignment can cause the transfer of body weight to be unbalanced and asymmetric and potentially cause overload somewhere along the kinetic chain.11 Lower extremity malalignment, compensations, and potential pain patterns occur from the pelvis down and from the foot up in the kinetic chain. Skeletal malalignment includes static deformities present in individual bone segments (i.e., tibial torsion), as well as joint deformities (i.e., genu valgus).57
The most common types of malalignment are axial and rotational. Axial alignment refers to the longitudinal relationships of the limb segments.58 Axial alignment is described in reference to the angle made by the segments in relation to a straight line. Deviation toward the midline would relate to the term valgus. Deviation away from the midline is termed varus. Rotational alignment refers to the twisting of the limb around its longitudinal axis.58 Joint deformities, such as genu valgus, may increase in magnitude when assessed from a static non–weight-bearing position in contrast to more functional positions, such as unilateral limb weight bearing due to ground reaction forces coupled with foot pronation.57
Normal characteristics at the hip include an inwardly rotated relationship between the femoral neck relative to the femoral condyles of the femur in the transverse plane called femoral anteversion. Normal femoral anteversion ranges from 13 to 18 degrees in adults.11,57,59,60 Measurement of hip anteversion can be performed in the prone position by recording the angle of the lower leg from the vertical when the greater trochanter is placed in its most lateral and prominent position.11,57,61,62 Excessive femoral anteversion influences the knee joint medially, giving rise to a cascade of biomechanical stresses and compensations (Figure 10-17).11 An inwardly rotated knee creates excessive laterally directed shear forces resulting in increased stress in the medial patellofemoral ligaments and increased lateral patellofemoral compressive forces.11 The trochlea is pushed medially and forward against the patella, creating resultant excessive posterolateral pull on the patella. Lee et al63 describe that excess of femoral internal rotation of 30 degrees increases contact pressures on the lateral facets of the retropatellar surface. Riegger-Krugh and Keysor64 report possible femoral anteversion compensations in the kinetic chain as including excessive tibial external torsion, excessive pelvic external rotation, and excessive ipsilateral lumbar spine rotation. Predisposition for altered mechanics as described by Powers et al13 is set up by excessive femoral anteversion. Clinically, the requirement for strong muscular stability from the hip and pelvis to limit knee stress is amplified.
Femoral retroversion is defined as excessive external rotation of the femoral neck relative to the femoral condyles in the transverse plane. Excessive external rotation of the femur magnifies retropatellar articular surface stress along the medial facet.65 Compensations include excessive tibial internal rotation and pelvic internal rotation with contralateral lumbar spine rotation.64 Physical examination of excessive femoral anteversion and retroversion demonstrates distinctive range of motion differences. An excessively anteverted hip will demonstrate an excess of hip internal rotation and limitation of external rotation.66,67 Conversely, a retroverted hip will demonstrate excessive hip external rotation and limitation of hip internal rotation.
Genu valgus is defined as the angle formed at the knee between the femur and tibia in which the knee angulates toward the midline with the tibia angulating away from the midline.58 Frontal plane knee valgus posturing in weight bearing is considered normal at approximately 6 degrees.57,68 Excessive knee valgus correlates with excessive pronation as gravity influences the lower limb toward the midline in weight bearing.64 Radiographically and clinically, this can be measured by the angle bisecting the midline position of the distal femur with the midline position of the proximal tibia.57 Excessive valgus results in disproportionate compressive forces laterally and distractive tensile forces medially. Conversely, knee varus posturing produces compressive forces medially and increased tensile forces laterally.57 Genu varum is defined as the angle formed by the femur at the knee directing away from the midline and the tibia angling back toward the midline.58 This contact stress asymmetry leads to structural failure, such as arthrosis and chondromalacia.11
Excessive tibial varum is a common congenital osseous deformity in which the distal one third of the leg is angled medially in the frontal plane (Figure 10-18).40,69 The varum angle occurs within the shaft of the tibia as opposed to the angulation occurring at the joint in the genu varum deformity condition. Measurements of tibial varum are described in three positions: double-limb stance with subtalar joint in the resting position, double-limb stance with the subtalar joint in neutral, and single-limb stance with the subtalar joint in the resting position.70 Lohmann recorded tibial vara measurement in uninjured subjects and found the single-limb stance measurement to be the largest at 6.35 degrees and double-limb stance with the subtalar joint in neutral to be the smallest at 4.62 degrees relative to these three test positions. Tomaro40 found a significant difference in the amount of tibial varum between the involved and uninvolved extremities in 20 patients with various lower extremity overuse injuries. He found an increased tibial varum measurement on the side of overuse symptoms with measurements at 5.3 degrees compared with 4 degrees on the uninvolved extremity. Increased tibial varum is related to compensatory excessive subtalar joint pronation because this malalignment tends to elevate the medial foot from the support surface, requiring increased pronation to achieve foot flat. Ross and Schuster41 describe a concept of total varus imbalance that includes the summation of tibial varum, rearfoot varus, and forefoot varus measurements. Tibial varum was measured in stance, while rearfoot and forefoot varus were measured non–weight bearing. A preseason screening examination of 63 runners was then correlated to the summation of varum measurements. A low injury rate was described with individuals of less than 8 degrees of total varus, and a high injury rate was found in runners with more than 18 degrees of total varum summation.
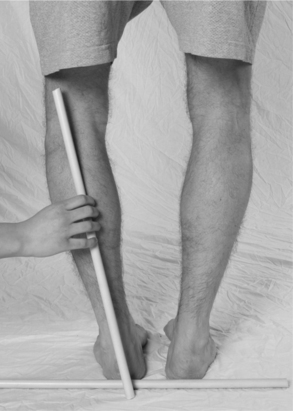
Figure 10-18 Excessive tibial varum in double-limb stance. Distal one third of the tibia is angled medially in the frontal plane.
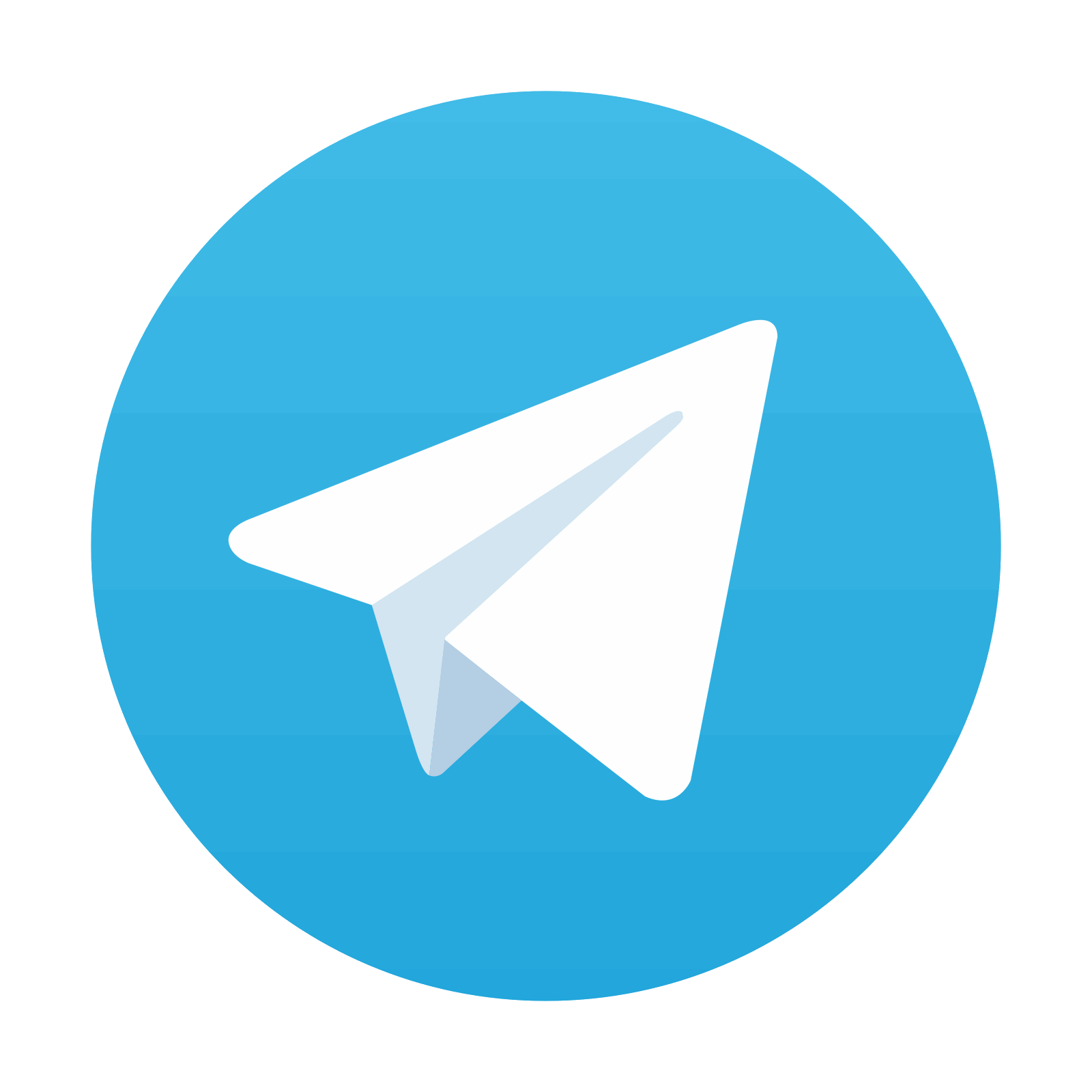
Stay updated, free articles. Join our Telegram channel

Full access? Get Clinical Tree
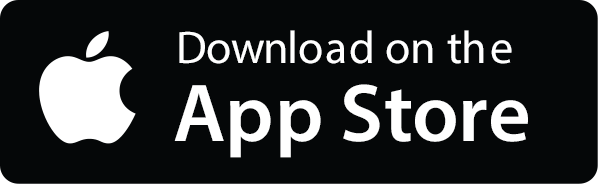
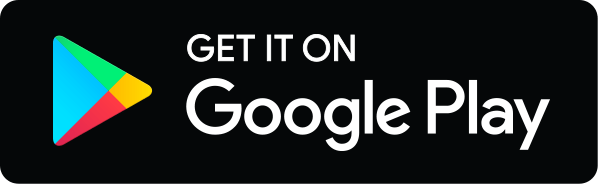