Exercise Principles
Shawn Kerger
OVERVIEW
Osteopathic philosophy and practice is truly at its pinnacle when dealing with exercise principles in the athlete. The mechanism at work (or play) as envisioned by Andrew Taylor Still in 1874 is in full stride and can be appreciated for its inherent wisdom and grace. Today, we study and expound on the principles established decades and even centuries ago by thinkers who observed phenomena on a larger scale to explain that which they could not see, but whose intuition told them must be present and thus created new facets and fields of medicine.
The three principles proposed by A. T. Still, which the faculty of the Kirksville College of Osteopathy and Surgery restated in 1953, are as follows (1):
The body is a unit.
Structure and function are reciprocally interrelated.
The body is self-healing.
Such concepts are uniquely suited to exercise and sport. They have tremendous implication in the diagnostic and therapeutic aspects of sports medicine.
More modern applications of these principles are easily seen both in the laboratory as well as on the playing field. Recent trends in exercise have largely focused on the principle of body unification: Pilates, dynamic lumbar stabilization (or core stabilization) training, yoga, and Tai Chi are all variations on this theme. Kinetic chain and sequencing studies in biomechanics laboratories across the country seek to quantify and qualify athletic skill. The human body’s structure-function reciprocity and self-healing nature have been proved by the laws pertaining to its response to external and internal stimuli by structural change for some time now. These principles have advanced beyond the interactions of muscle and bone and currently incorporate neuron complexes, individual cellular structure, and proteomics into their milieu.
In an effort to facilitate freedom of thought and adaptation to clinical scenarios, one should focus on the study of normal functioning and only then on abnormal situations. By not classifying disease states as separate entities but rather as a misguided or an improper expression of a healthy physical state, the practitioner can more easily be of a frame of mind to find the health contained within the athlete.
BONE/JOINT/LIGAMENTOUS STRUCTURES
The skeleton tells a history of the body’s formation: forces of tension and compression mold the shape and density of bone from its mesodermic origin. Tubercles are the result of tensile forces, such as the quadriceps has on the tibial tuberosity and the sartorius on the anterior superior iliac spine. Grooves are created by the growth of bone around a compressive force, such as the bicipital groove, or when tension around a future groove creates opposing ridges, such as in the groove for the radial nerve, which is bordered by the lateral and medial heads of the triceps. Subsequent adaptation to force follows Wolff’s law: Bone is resorbed where force is not present and concentrated in areas of increased force (5).
By virtue of its inherent structure combining type I collagen and the minerals contained within bone matrix, compact bone has tremendous resistance to both compressive and tensile forces. Bone’s resistance to tensile strength along its grain is superior to that of white oak, and its compressive resistance is more than 150% that of granite. Only steel has superior resistance to both compressive and tensile force (2). Much of bone’s strength can be lent to its inherent adaptation of the principles of tensegrity—an architectural system in which structures stabilize themselves by balancing the counteracting forces of compression and tension (3). The chemical structure of hydroxyapatite (the cornerstone of bone formation) is a primary structure in tensegrity—the icosahedron. This principle has vast implications of which we are just recently becoming cognizant, and we will examine some of these later in the chapter.
Tendons and ligaments contain dense, collagenous tissue arranged in a linear fashion and thereby resist motion specifically in one particular plane in order to best serve their function. These fibers do not run independently of each other, but rather intertwine to better spread the force more diffusely across the entire structure, decreasing the chance of failure (4). Ligaments and tendons also serve a feedback function to help with proprioception, that is, the sensation of joint position that is critical to force generation and control.
Joints and their capsules similarly affect proprioception and the accordant neuromuscular adaptations. Although two of the three major types of joints are considered immovable by most anatomists (including the cranial sutures), further studies are demonstrating motion at these joints, albeit extremely small motions (5). With our focus on the athlete, this review will concentrate on the synovial joints, as these provide the greatest degree of motion.
Synovial joints generally have reciprocating surfaces, such as the cup-and-ball arrangement seen in the hip, and the bony structure strongly dictates the function of the joint (although the reverse situation might exist quite frequently during development, and certainly exists during adult life, as is demonstrated in osteoarthritic knees). The shallower the articular arrangement, the more reliant the joint is on soft tissue stabilization to control the expanded range of motion (ROM) available. An articular capsule filled with synovial fluid sandwiched between articular cartilages, usually hyaline, surrounds the joint.
Proprioceptive and pain output from the joint comes from the capsule, the surrounding ligaments, and the periosteum—not from the articular cartilage. It is this capsule and the joint’s surrounding ligaments that respond with pain to a shear force, or more commonly, distension. This is why arthritic pain worsens with incoming storms or cold weather—the hydrostatic pressure inside the joint changes at a slower rate than the barometric pressure outside the joint (Fig. 10.1A and B).
The maintenance of intra-articular fluids is critical for several reasons: nourishment of the joint surfaces, cellular transport, lubrication, and cushioning. The synovial fluid carries with it not just the cells for maintenance of the joint, but also metabolic nutrients and waste products. Particulate matter, however, must be phagocytized. The very structure of synovial fluid (mostly hyaluronic acid) allows for cushioning and lubrication of the joint, and as one ages, this fluid decreases in volume and viscosity. This degeneration leads to a greater likelihood of chondral injury with a concomitant decrease in healing response. Hyaluronic acid supplements, both oral and injectable, have shown to help with osteoarthritic pain, but they do not seem to restore the articular cartilage.
Arthrokinetic responses are neuromuscular responses to joint position and output, and are a delicate and interwoven web of data processed by the central nervous system (CNS). These responses are separate from stretch reflexes, although some of the same nervous pathways are used. The four nerve types responsible for the afferent information from the joint are the following (6):
Globular (static and dynamic mechanoreceptors)
Conical (dynamic mechanoreceptors)
Fusiform (mechanoreceptor)
Plexus (nociceptor)
These impulses are conducted to the spinal cord and interface with the data from the muscles, tendons, and ligaments serving not just the same joint but also the surrounding joints. This integration allows for a snapshot of the body’s position for the CNS, on which it can act appropriately. When a capsule or ligament becomes stretched beyond its programmed length, or when motion occurs too quickly, two responses occur:
Inhibitory signals are sent to the agonist muscle responsible for loading the joint in the plane in question.
Stimulatory signals are sent to the antagonist musculature.
Thus, if the anterior hip capsule should be stretched more than its programming allows, the gluteal muscles will receive an inhibitory signal to reduce the forces acting on the capsule, while the hip flexor muscles will be stimulated. This is the case in both healthy and dysfunctional states, with the difference being when this process is activated.
Flexibility is the ability to move through a physiologic ROM. There are two types of stretches that can increase flexibility: elastic (temporary) and plastic (more permanent, but can still regress unless ROM is maintained). Joint ROM is primarily reduced due to connective tissue (muscle, ligament, tendon, or capsule). Muscles adapt their length more readily than their encasing fascia and attaching tendons, unless dysfunctional inputs to the muscle prevent adaptation. Therefore, most efforts to improve flexibility focus on plastic deformation of the connective tissues, and well-established techniques exist in the repertoire. Consideration of dysfunctional input to muscles will increase the success rate of practitioners dealing with recalcitrant or recurrent ROM problems in athletes.
MUSCLE
Similar to bone, muscle adapts its physical structure to the demands being placed on it. These structural changes occur at a macroscopic level with hypertrophy and increased tonicity of an exercised muscle, and also, to a limited degree, microscopically with regard to fiber type. The latter change reflects the type of demand repetitively placed on a muscle. It has been well documented that hypertrophy occurs in animal models to a much greater degree with
chronic loading of fibers than with the intermittent loading consistent with human exercise (7). Although initially classified by Engel (8) in 1962 as being one of two types of fibers, muscle fibers currently can be classified into one of seven fiber types, using pH sensitivity of myofibrillar adenosine triphosphatase histochemistry techniques (9). All muscles contain various percentages of fiber types, even within contralateral limbs of the same organism.
chronic loading of fibers than with the intermittent loading consistent with human exercise (7). Although initially classified by Engel (8) in 1962 as being one of two types of fibers, muscle fibers currently can be classified into one of seven fiber types, using pH sensitivity of myofibrillar adenosine triphosphatase histochemistry techniques (9). All muscles contain various percentages of fiber types, even within contralateral limbs of the same organism.
These fiber types respond to functional demands, hormonal signals, and neural input, but most of the muscle’s fiber type composition is due to genetic control (9). In completely artificial circumstances—60 days of continuous (24 hours/day) low-frequency stimulation of a rabbit tibialis anterior muscle (10), for example—muscles can undergo complete fiber type changes. Most current research suggests that fiber type change in response to more reasonable types of exercise is not as significant as was anticipated years ago. For an unknown reason, both strength and endurance types of exercise result in the change of fast-twitch type IIB fibers to type IIA fibers (9). Therefore, if change in fiber type does not account for performance improvement with a certain form of exercise—what does? Hypertrophy of fiber types appropriate to the exercise, neurologic adaptations, skill development, metabolic changes, and other pathways are probably the answer. Muscle responds to and, to a small degree, can change its fiber composition, but generally adapts its structure within the existing fiber type and relies on other mechanisms of the body to assist with physical demands.
When considering muscular firing patterns in normal activities and in sports and their associated kinetic chains, origin-and-insertion thinking becomes obsolete. If a picture is worth a thousand words, a three-dimensional anatomic appreciation is certainly worth a million. Muscle action has classically been defined as concentric, eccentric, and isometric, with the defining characteristic of each being shortening, lengthening, or maintaining the same length of a muscle while under load, respectively. Recently, the concept of “econcentric” contractibility has been proposed as the way a muscle (or even portions of a muscle) may function in any of the three classic phases of action at one joint or multiple joints simultaneously (11). Such a term is used to more accurately define function in a variety of tasks, especially with regard to the kinetic chain. The kinetic chain is described as the sequencing of individual body segments and joints to accomplish a task (12). It generally functions from a base of support proximally and then proceeds distally, but this depends entirely on the task at hand: A bench press would follow the aforementioned path, but a push-up reverses the mechanics even though the muscles engaged are very similar, if not identical.
A helpful way of conceptualizing muscular action and function (and subsequently, dysfunction) is to categorize muscles by their method of functioning, as described early on by Rood’s concept of stabilizer and mobilizer movements (13) and subsequently expanded on by Janda (14) and Sahrmann (15). This concept extends beyond simple contracture and relaxation, and more toward the characteristics exhibited during muscular activity. Tonic muscles are those maintaining a low level of tone nearly all the time, functioning primarily as postural muscles and using more fibers of an oxidative nature to avoid fatigue. Examples of tonic muscles are iliopsoas, rectus femoris, quadratus lumborum, scalenes, gastrocnemius, pectoralis major, and the biceps. Phasic muscles exhibit quicker, shorter bursts of activity with phases of rest in between and more often use the glycolytic pathway fibers. Examples of these muscles are vastus medialis and lateralis, gluteal muscle complex, midthoracic erector spinae, tibialis anterior and peroneal muscles, rhomboids, lower trapezius, and triceps.
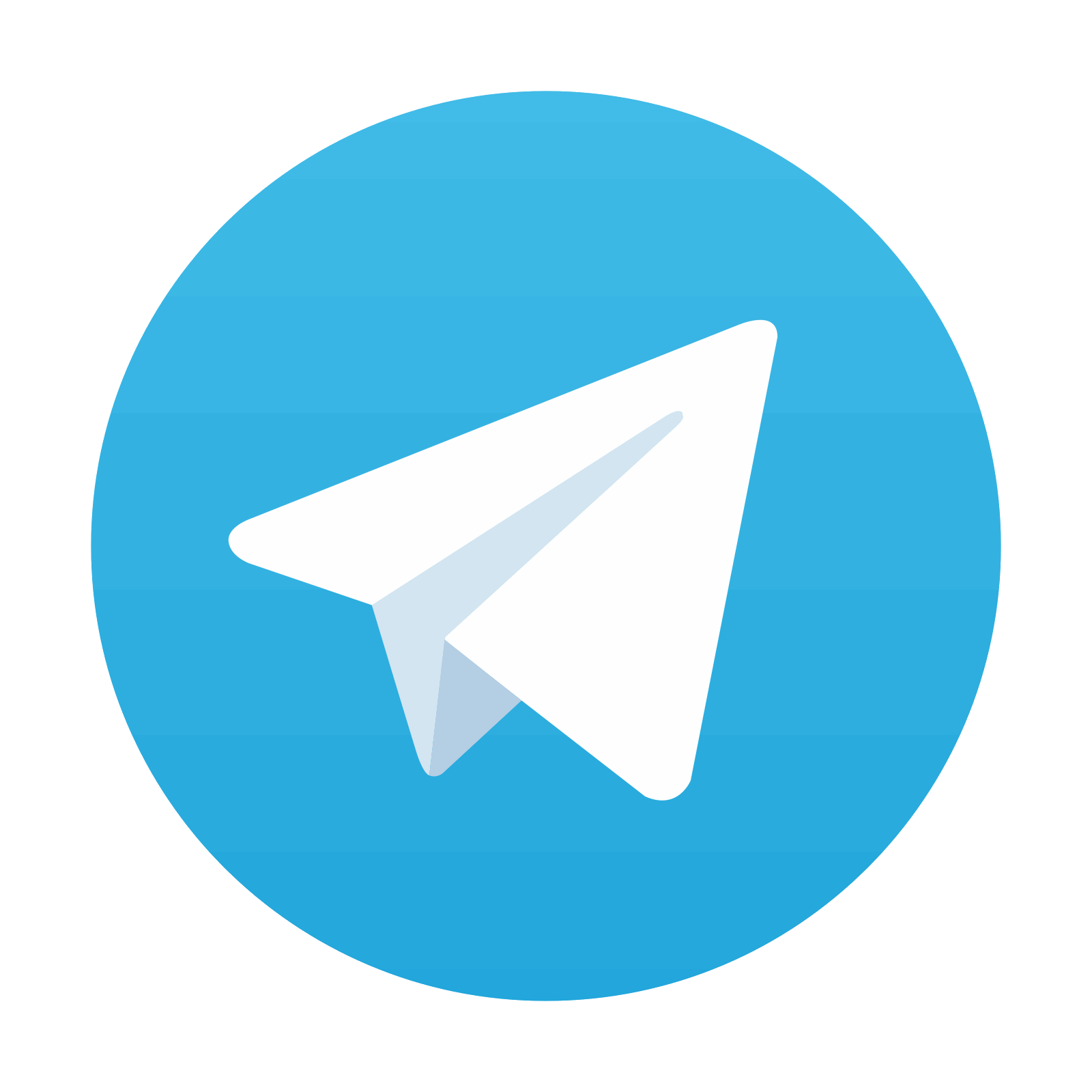
Stay updated, free articles. Join our Telegram channel

Full access? Get Clinical Tree
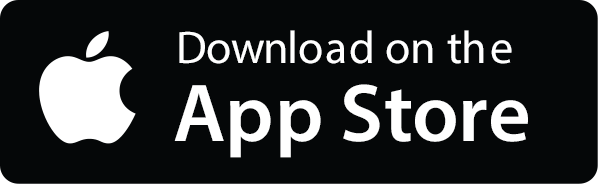
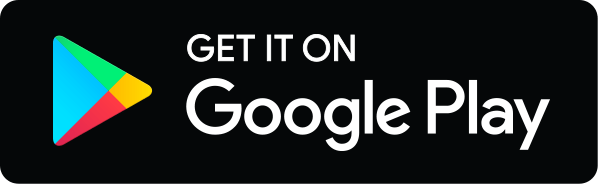