CHAPTER 23 Electrical stimulation
Electrical stimulation is a physical wound care modality that uses the transfer of electrical current to tissues to support wound healing. Devices used for wound healing applications consist of a source of electrical current, a minimum of two wires or leads, and their corresponding electrodes (Figure 23-1). One lead is connected to the negative jack of the device and is the source of negatively charged electrons in an electrical circuit; this is referred to as the cathode. The other is connected to a positive jack on the device that serves as an electron depository for the flow of electrons in the electrical circuit; this is called the anode. Electrodes are attached to the patient end of the wires and placed on either the patient’s wound bed or the adjacent skin. When the device is operating, a unidirectional current flows through this circuit, causing positively charged ions (Na+, K+, H+) in the tissues and positively charged cells (activated neutrophils and fibroblasts) to migrate toward the cathode; negatively charged ions (Cl−, HCO3−P− and negatively charged cells (epidermal, neutrophils, and macrophages) migrate toward the anode. To understand the basis of electrical stimulation as an adjunctive therapy for wounds, it is important to understand several terms. Table 23-1 provides definitions of relevant terms.
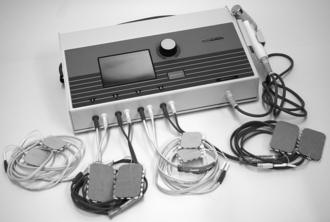
FIGURE 23-1 Example of a high-voltage pulsed current electrical stimulation device.
(Courtesy Rich-Mar Corporation, Inola, Okla., USA.)
TABLE 23-1 Terms and Definitions for Electrical Stimulation
Terms | Definitions |
---|---|
Alternating current | Uninterrupted, bidirectional current flow |
Amperage | Measure of rate of flow of current; expressed as amperes (A), milliamperes (mA), or microamperes (μA) |
Amplitude | Maximum (peak) excursion of voltage or current pulse |
Charge | Property of matter determined by proportion of electrons (negatively charged particles) contained by the matter; substance may be neutral, positively charged, or negatively charged; measured in units of coulombs (C) or microcoulombs (μC) |
Current | Rate of flow of charged particles (ions or electrons); measured in units of amperes (A) or milliamperes (mA) |
Direct current | Uninterrupted, unidirectional current flow |
Frequency | Number of pulses delivered per unit of time; also termed pulse rate; frequency is the reciprocal of cycle time; usually measured as pulses per second (pps) or hertz (Hz); 0.1 Hz is on for 10 seconds, and a pulse of 1,000 Hz is on for 1 ms |
Interpulse interval | Time between pulses when no voltage is applied and no current is flowing |
Polarity | Property of possessing two oppositely charged electrodes in an electrical circuit (positive and negative); negative electrode (cathode) provides electrons in a circuit; positive electrode (anode) serves as depository to which electrons flow |
Pulse duration | Time during which current is flowing |
Voltage | Measure of force of flow of electrons through a conductor (wound tissue) between two or more electrodes; created by difference of charges between two electrodes (one with excess in relation to the other); electrodes are polarized in comparison to each other (negative electrode and positive electrode) |
Waveform | Graphic representation of current flow; may be monophasic (current that deviates from isoelectric zero line in one direction and then returns to baseline) or biphasic (current that deviates above and below isoelectric zero line); may be symmetrical or asymmetrical |
Effects of electrical stimulation
In an effort to examine the entire body of evidence on electrical stimulation and chronic wound healing, Gardner et al (1999) conducted a meta-analysis of 15 studies that assessed the efficacy of electrical stimulation. Studies included in the meta-analysis were limited to randomized controlled trials (n = 9) and nonrandom controlled trials (n = 6). Meta-analysis procedures were applied to a total of 24 electrical stimulation samples (n = 591 wounds) and 15 control samples wounds (n = 212). The calculated average rate of healing for the electrical stimulation samples was 22% per week compared to 9% for the control samples. The net effect of electrical stimulation therapy was 13% per week, a 144% increase over the control rate. These data provide substantive evidence that electrical stimulation can produce substantial improvement in the healing of chronic wounds. The physiologic effects of electrical stimulation that contribute to its wound healing efficacy include galvanotaxic, stimulatory, antibacterial, blood flow, and tissue oxygenation.
The underlying physiologic effects of electrical stimulation are mediated by the endogenous bioelectric system in the human body and its response to positive and negative polarity. Several investigators have demonstrated the existence of transepithelial potentials on the skin surface (Barker et al, 1982; Illingsworth and Baker, 1980). These transepithelial potentials arise from Na channels on the mucosal surface of the skin that allow Na to diffuse from the area surrounding epidermal cells to the inside of the cells. As a result of the movement of Na from the skin surface to the interior of epidermal cells, the exterior of the skin maintains a variable level of negative electrical charge (Foulds and Barker, 1983). Jaffe and Vanable (1984) demonstrated that when the epidermis is injured, current flows as ions are transmitted through the tissue fluid between the damaged regions of the epidermis. This “current of injury” has a positive polarity, whereas the adjacent intact skin retains its negative polarity, producing a unidirectional force sufficient to attract reparative cells to the wound bed during the inflammatory and proliferative phases of healing (Vanable, 1989). Once reepithelialization has closed the wound, the current of injury disappears, suggesting that it does not play a role in the remodeling phase of healing. It is important to note that the flow of current out of the wound is blocked if the wound bed is allowed to desiccate and form a scab (Alvarez et al, 1983).
Galvanotaxic effects
Positively and negatively charged cells are attracted toward an electric field of opposite polarity, a process termed galvanotaxis. Multiple in vitro studies have established that cells essential to tissue repair will migrate toward the anode or cathode created by an electric field within a tissue culture (Bourguignon and Bourguignon, 1987; Eberhardt et al, 1986; Orida and Feldman, 1982; Stromberg, 1988). Preliminary evidence from in vivo studies has demonstrated that electrical stimulation creates a similar galvanotaxic response. Eberhardt et al (1986) showed that treating wounds with electrical stimulation for 30 minutes increased the relative number of neutrophils in the wound exudate compared to control wounds. Using the pig model, Mertz et al (1993) treated experimentally induced wounds with two 30-minute sessions of monophasic pulsed current (PC) using varying polarity. They found that wounds initially treated with negative polarity (day 0) followed by positive polarity on days 1 to 7 showed 20% greater epithelialization compared to wounds that received only one type of polarity. In the wounds treated by alternating polarity daily (negative one day, positive the next), epithelialization was limited by 45%. Although the influence of polarity on cell migration in human wounds remains to be elucidated more completely, these findings suggest that bioelectric signals play a role in facilitating the phases of healing.
Stimulatory effects on cells
Basic science studies have shown that electrical current has a stimulatory effect on fibroblasts. Using the pig model, Cruz et al (1989) demonstrated the presence of significantly more fibroblasts in burn wounds treated with electrical stimulation than in controls. Alvarez et al (1983), also using the pig model, documented more fibroblasts and increased collagen synthesis in partial-thickness wounds, thus substantiating previous findings. Castillo et al (1995) found significant increases in collagen density with burn wounds in the rat model. The stimulatory effect of electrical current on fibroblasts, reported by Bourguignon and Bourguignon (1987), was observed when fibroblasts in culture increased DNA and protein (including collagen) synthesis in response to electrical stimulation. This effect was most noticeable near the negative electrode.
Blood flow and tissue oxygen effects
Accumulating evidence indicates that electrical stimulation exerts a positive influence on blood flow and localized tissue oxygen. Hecker et al (1985) showed that negative polarity increased blood flow in the upper extremity as measured by plethysmography. In a sample of patients diagnosed with Raynaud disease and diabetic polyneuropathy, Kaada (1982) demonstrated that application of distant, low-frequency transcutaneous electrical stimulation (TENS) produced pronounced and prolonged cutaneous vasodilation. Using skin temperature as a measurement of peripheral vasodilation, Kaada found a rise in the temperature of ischemic extremities from 71.6°F to 75.2°F (22°C–24°C), to 87.8°F to 93.2°F (31°C–34°C). The latency from stimulus onset to the abrupt rise in temperature averaged 15 to 30 minutes with a duration of response ranging from 4 to 6 hours. Kaada (1983) subsequently reported successful treatment of 10 patients with 19 leg ulcers, previously resistant to treatment, by applying a TENS device to the web space between the first and second metacarpals of the ipsilateral wrist. Using the burst mode, Kaada delivered 15 to 30 mA of pulsed direct current by the cathode for 30 to 34 minutes three times per day. He proposed that the remote application of electrical stimulation enhanced microcirculation in the tissues of the ipsilateral lower extremity, as demonstrated by the increase in toe temperature and ulcer healing. Based on findings from subsequent basic research, Kaada suggested that the improvement in tissue microcirculation was the result of activation of a central serotonergic link that inhibits sympathetic vasoconstriction (Kaada and Helle, 1984; Kaada et al, 1984).
Additional evidence of increased blood flow in wounds treated with electrical stimulation is provided by reports of increasing capillary density following implementation of this therapy. Fifteen venous leg ulcers, previously resistant to healing, were treated for 30 minutes daily for an average of 38 days (Junger et al, 1997). Using light microscopy to measure capillary density, they found densities increased from a prestimulation baseline of 8.05 capillaries/mm2 to 11.55 capillaries/mm2 following stimulation (p < .039). Transcutaneous oxygen in the periwound skin was noted to increase from 13.5 to 24.7 mm Hg.
Although research on the effects of electrical stimulation on wound oxygenation has been minimal, preliminary studies demonstrate that cutaneous oxygen can be improved with electrical stimulation. In separate studies, investigators showed that application of electrical stimulation improved cutaneous oxygen in the lower extremity of elderly patients, subjects with diabetes, and patients with spinal cord injuries (Gagnier et al, 1988; Peters et al, 1998). These works provide indirect evidence of the potential for electrical stimulation to increase wound oxygenation.
Antibacterial effects
Evidence indicates that electrical stimulation has bacteriostatic and bactericidal effects on microorganisms that are known to infect chronic wounds (Daeschlein, 2007). In a study of 20 patients with burn wounds that had been unresponsive to conventional therapy for 3 months to 2 years, Fakhri and Amin (1987) showed a quantitatively lower level of organisms after treatment for 10-minute intervals twice weekly. This decrease in bacterial count was accompanied by epithelialization of the wound margins within 3 days of beginning electrical stimulation. Although the mechanism underlying the bactericidal or bacteriostatic effects remain unclear, the galvanotaxic effect on macrophages and neutrophils has been implicated (Eberhardt et al, 1986; Orida and Feldman, 1982). These studies suggest that the anodal attraction of neutrophils to tissue with high bacterial levels may be a primary mode of action, rather than destruction of pathogens by electrolysis or elevation of tissue pH. However, these studies used direct current, which is not as commonly used in wound management as the PC type (see section on types of electrical stimulation). It appears that the voltage required to produce an antibacterial effect with PC would create profound muscle contractions and therefore would not be applicable in clinical practice (Guffey and Asmussen, 1989; Kincaid and Lavoie, 1989; Szuminsky et al, 1994).
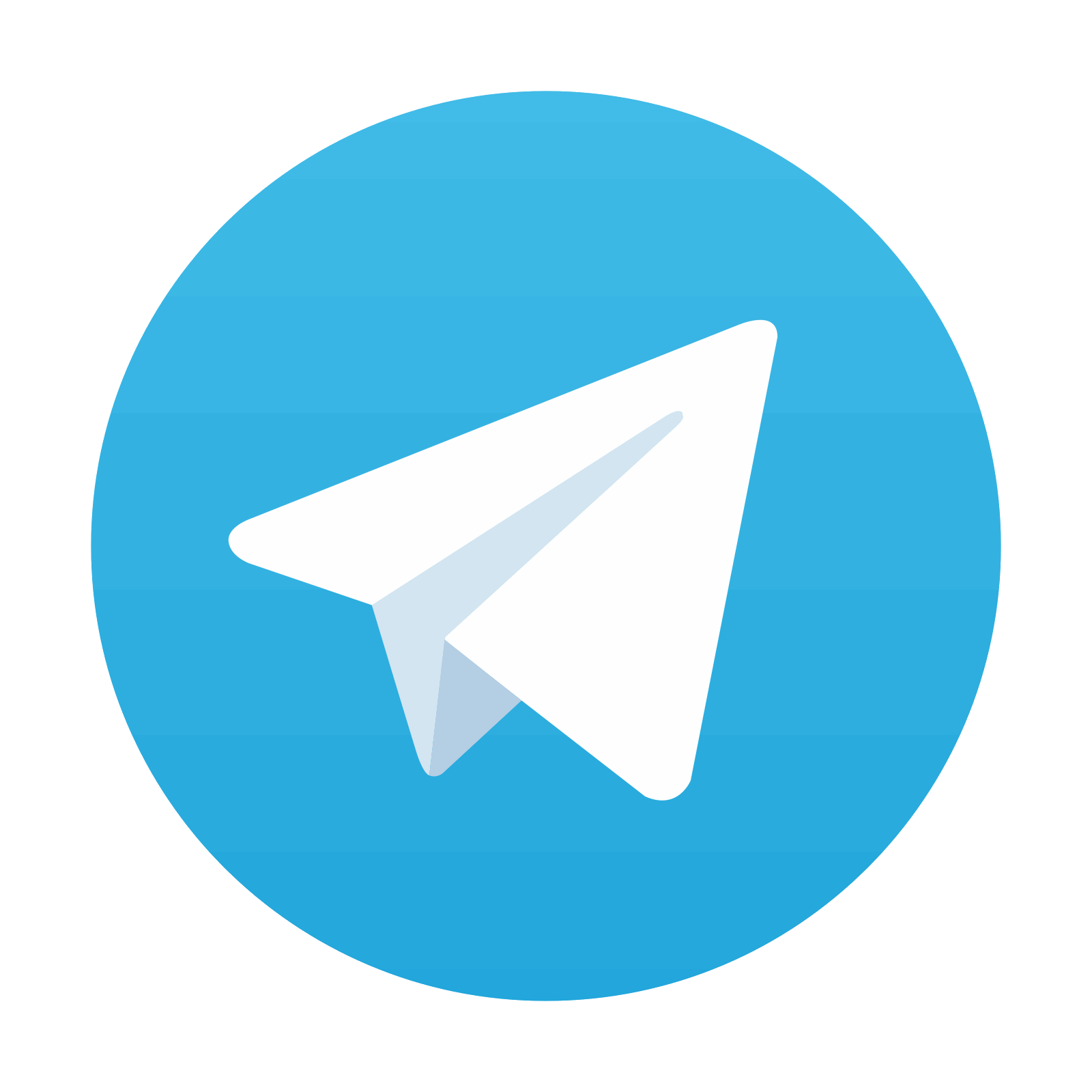
Stay updated, free articles. Join our Telegram channel

Full access? Get Clinical Tree
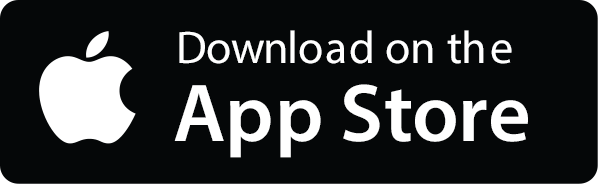
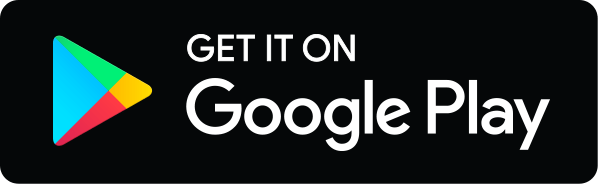