Electrical and Mechanical Bone Growth Stimulation
Michael S. Downey
Wen-Yin Choi Wang
Electrical energy was first described in an effort to achieve bone healing in 1812 when Birch was credited with the healing of a tibial nonunion by “shocks of electric fluid” passed between the fractured ends of the bone (1). Subsequently, sporadic reports of the use of electricity in bone healing were described over the next 150 years. In 1957, the investigation into bone healing with electricity was reinvigorated by the work of Fukada and Yasuda (2), who demonstrated that the mechanical deformation of bone produced electrical potentials. In 1971, Friedenberg et al (3) used direct current (DC) for the management of a nonunion of the medial malleolus. In 1981, Brighton et al (4) reported their results in a multicenter evaluation on the use of constant DC for the management of osseous nonunion. The initial description of a totally implantable DC bone stimulator appears to have been that of Dwyer and Wickham in 1974 (5). In 1981, the first application of a noninvasive method of bone growth stimulation was described by Bassett et al (6), when they applied pulsed electromagnetic fields (PEMFs) in the treatment of a tibial diaphyseal nonunion.
A variety of therapeutic devices are available to provide electrical and mechanical stimulation for the augmentation of bone healing. In addition, the same devices have been studied and/or applied in the management of other clinical problems, including the treatment of avascular necrosis, congenital pseudarthrosis, failed and initial arthrodesis, osteoporosis, chronic refractory tendonitis, osteoarthritis, stress fractures, and acute Charcot osteoarthropathy. Electrical stimulation of nonunited fractures and osteotomies, particularly when applied in a noninvasive manner, is generally reported as being safe. A diagnostic and therapeutic technology assessment by the American Medical Association concluded, “the safety of noninvasive electrical stimulation for the treatment of nonunions has been established” (7).
Numerous retrospective and prospective studies appear to attest to the effectiveness of electrical and ultrasonic stimulation in the management of nonunions and fresh fractures (8,9,10,11,12 and 13). More recently, several systematic review and meta-analyses of randomized trials have further supported the value of electrical and mechanical stimulation in bone healing (14,15,16 and 17). Although these studies collectively support the use of electrical and mechanical stimulation in the healing of nonunions and fresh fractures, further study with large scale, randomized trials is needed to make broad and decisive conclusions regarding these modalities.
BIOPHYSICS OF BONE HEALING
It is well known that changes in the environment or forces applied to bone and joints result in adaptation within these structures. Adaptation of bone in response to environmental factors is explained by Wolff’s law, which states “The law of bone remodeling is that mathematical law according to which observed alterations in the internal architecture and external form of bone occur as a consequence of the change in shape and/or stressing of bone” (18).
The alterations in the radiographic appearance of bone in response to stress and the sequential changes of healing or remodeling of bone following fracture or osteotomy imply that from an engineering viewpoint, bone must possess a control system that is activated during various stages of physiologic function (19). In recent years, researchers studying the structural adaptations of bone to stress and other applied forces have focused on the electromechanical properties of osseous tissue as a means by which these alterations are mediated.
In 1957, Fukada and Yasuda (2) demonstrated that when stress is applied to a bone in such a manner as to cause deformity, electrical potentials are generated within the bone. The appearance of electrical potentials within bone in response to the application of an external force is referred to as a piezoelectric property. The electrical signals generated within bone in response to stress are known as endogenous stress-related potentials (20).
ENDOGENOUS STRESS-RELATED POTENTIALS
Two sources of endogenous stress-related potentials appear to exist within bone—piezoelectric and electrokinetic. The potentials are biphasic, producing current flow in one direction with the application of stress and in the opposite direction when that stress is removed. These potentials are of a negative polarity in areas of compression and a positive polarity in areas of tension. Bone production occurs within the electronegative areas, whereas bone resorption occurs within the electropositive areas (21) (Fig. 89.1).
STEADY-STATE POTENTIALS
In addition to stress-related potentials, bioelectric or steadystate potentials are also recorded from the surface of a living bone even when no stress has been applied and appears to be unaffected by denervation or arterial ligation.
Steady-state potentials are significantly electronegative at the site of growing bone, as exemplified by physeal plates. In addition, strongly electronegative bioelectric potentials may be recorded throughout a fractured or osteotomized bone, with the peak of electronegativity recorded at the site of bone healing (22,23).
CELLULAR AND BIOCHEMICAL RESPONSE TO ELECTRICAL AND SONIC STIMULATION
Although stress-related and steady-state electrical potentials are very small, the discovery of these processes resulted in the conclusion that Wolff’s law was in effect mediated by electrical impulses. Clinically, the hypothesis that the exogenous application of electronegative currents may stimulate osteogenesis is the basis upon which bone growth stimulation is employed.
The effects of electric and electromagnetic fields on osteogenesis at the site of delayed unions and nonunions have been reported. A variety of methods to develop an exogenous electrical potential have been used, including constant DC (24,25), pulsed DC (26,27), PEMF stimulation also known as inductive coupling (28), capacitively coupled electrical fields (29,30), and combined magnetic fields (CMFs) (31). Although all of these devices administer exogenous electrical stimulation by different techniques, all have been associated with the healing of nonunions at a rate comparable with that of bone grafting (32,33).
A variety of mechanisms by which endogenous and exogenous electrical potentials stimulate bone healing have been proposed. The biochemical effects of PEMFs, reviewed by Cheng (34), include significantly increased DNA synthesis by chondroblasts, increased collagen synthesis, an increased rate of amino acid transport functions across cellular membranes, enhanced H-noradrenaline release from nerve cell lines, and reduced cellular cAMP in mouse osteoblast cultures. An increase in mRNA expression of multiple bone morphogenic proteins (BMPs), namely, BMP-2 and BMP-4, is key in mediating osteoblastic proliferation (35). These responses to PEMFs indicate accelerated metabolic activity, implying the potential for rapid healing of connective tissue. In addition, increased proteoglycan synthesis (36) in bone matrix, increased calcification of bone matrix, and increased proliferation of both osseous and cartilaginous cells have been noted in response to electrical stimulation (22).
In vitro studies aimed at the elucidation of biochemical pathways showed that initial transduction of capacitive coupling signals is at the level of the bone cell membrane, allowing for influx of calcium from the entire (essentially infinite) extracellular matrix, while inductive coupling and combined electromagnetic fields acted within or upon intracellular calcium stores. In all three instances, the final pathway is the same, leading to an increase in cytosolic calcium, and an increase in activated calmodulin, which leads to cell proliferation (37).
Ultrasound is cyclic acoustic pressure waves generated at frequencies above the human limit of hearing (roughly 20 kHz). It is a form of mechanical energy that can result in biochemical events at the cellular level and may promote bone formation in a manner comparable with the bone responses to mechanical stress postulated by Wolff’s law (12). In 1983, Duarte (38) was the first to report acceleration in the healing of fresh fractures with a low-intensity ultrasound device in animal studies. Numerous studies in other animal models and human clinical studies have confirmed the effectiveness of low-intensity ultrasound in the management of fresh fractures. One proposed mechanism is that ultrasound increases the number of degranulated mast cells at the site of injury. These degranulated mast cells cause an amplified cascade of chemical mediators, including histamine, leading to the quicker influx of inflammatory cells that initiate the bone reparative process. Vascular endothelial growth factor (VEGF) is also released, causing increased angiogenesis. Chondrogenesis and osteogenesis proceed via an increased uptake of calcium, increase in growth factors, and BMPs (39). Several studies have shown that this mechanical force modulates bone formation both in vitro and in vivo (12). The differential absorption of ultrasound may create a gradient of mechanical stain in healing bone callus that stimulates periosteal bone formation (40).
At least 30 known or probable factors have been described to explain the cellular and mechanical responses of bone to electrical stimulation and ultrasound (41). Several fundamental questions regarding the characteristics of exogenously administered electrical or electromagnetic energy and the concept of targeted biologic response remain to be investigated. In normal bone growth or repair, endogenous electrical potentials serve to autoregulate osseous metabolic processes, with changes in the physical and electrochemical environment reflected by changes in potential within the bone. This is analogous to a rheostat light switch, which may be adjusted to various levels between on and off. Whether a similar variability in an exogenously administered electrical field is necessary remains unclear. It is possible, for example, that the electrical characteristics of a recent nonunion, chronic nonunion, infected nonunion, nonunion with internal fixation, and their varying qualitative and quantitative circumstances demand greater selectivity in the characteristics of the applied fields and their interactions with endogenous bone potentials.
Finally, while many human clinical trials have tested and shown the effectiveness of bone growth stimulation, there is still significant research being done to determine the specific mechanisms of action and ideal delivery systems. Many of these studies are being performed in vitro or in animal models, and as
invaluable as these studies may be, it is important to recognize that in vivo conditions include many other factors, including intracellular and extracellular interactions.
invaluable as these studies may be, it is important to recognize that in vivo conditions include many other factors, including intracellular and extracellular interactions.
INDICATIONS
NONUNION AND DELAYED UNION
The primary indication for electric- or electromagneticinduced bone stimulation is delayed union or nonunion following fracture, osteotomy, attempted arthrodesis, or infection. Electrical stimulation has been used as the primary modality for treatment in up to 40% of patients with nonunions (42). The use of electric or electromagnetic stimulation on bone may, and should usually, be used as a secondary modality in combination with other measures to enhance healing, including cast or brace immobilization, internal or external fixation, bone grafting, and débridement and concurrent management of infection in bone or soft tissue.
No universal agreement exists as to the precise definition of a nonunion. A general understanding is a situation in which bone has failed to heal and all outward clinical and radiographic evidence of reparative processes has ceased. Previously, the U.S. Food and Drug Administration (FDA) defined a nonunion as being established when a minimum of 9 months had elapsed since injury and the fracture site showed no visibly progressive signs of healing for a minimum of 3 months. After this original definition was released, many clinicians argued that the 9-month time period represented an arbitrary cutoff point that did not reflect the complicated variables present in many fractures, for example, degree of soft tissue damage, alignment of the bone fragments, vascularity, and quality of the underlying bone stock. Other proposed definitions evolved defining a nonunion as being present when the fracture was 3 to 6 months from original healing or simply when serial x-rays fail to show any further healing. Later, the FDA broadened its definition, stating a nonunion is considered to be established when the fracture site shows no visible, progressive signs of healing. Certainly, a clinician’s judgment plays a role in this determination as well. In severe trauma with extensive soft tissue, vascular, and neural injury, there is a higher prevalence of nonunion or delayed union. In these cases, patients should be followed closely with a high level of suspicion for the development of a nonunion (43). Delayed union generally refers to a decelerating of the bone healing process, as identified on serial radiographs. (In contrast, serial radiographs of a nonunion show no evidence of healing.) When lumped together, delayed union and nonunion are sometimes referred to as “ununited fractures.”
The precise differentiation of a delayed union from nonunion may be even more difficult to define clinically and radiographically. Asymptomatic cartilaginous or even fibrous union may occur within a bone that demonstrates the radiographic characteristics of nonunion. Under these circumstances, a reduction in pain or other signs and symptoms that are extraosseous in nature may be used to determine the presence of a radiographically occult union.
Many clinicians have advocated the use of electrical stimulation of bone “as soon as tardy union of bone is identified” (11). Their argument is that the early institution of electrical stimulation of a delayed union will reduce the disability time, as well as the incidence of nonunion (30,44).
A benchmark study of PEMF stimulation in humans was first performed on congenital or acquired pseudarthroses that were previously resistant to surgical treatment. PEMF therapy was used as a last attempt at salvage prior to amputation. The success rate was 73% and 76%, respectively (45). Patients who had failed multiple surgeries healed their acquired pseudarthroses with adjunctive PEMF treatment and long-term assessment showed that the union achieved remained intact. In the less fortunate cases of congenital pseudarthroses, patients would heal with PEMF stimulation but refractured elsewhere (27,46).
A study comparing treatment of tibial nonunions with capacitive coupling, DC, and bone grafting all showed similar rates of healing when there were no other risk factors involved (i.e., duration, atrophic nonunion, comminuted fracture, infection) (33). Bone growth stimulation may be a reasonable option prior to returning to the operating room to surgically repair the nonunion.
Ultrasound has also been successful in the treatment of nonunions, with success rates of ranging from 85% to 100% in multiple, smaller studies. In long-term follow-up, the healed areas remained intact. Not only was time to union significantly shorter in the studies, there was also better maintenance of fracture reduction (12,13,47).
BONE GRAFTING
Electrical stimulation may augment the normal incorporation of bone grafts (48), and a synergistic effect of bone grafting and electrical stimulation has been reported (49,50 and 51). Bone grafting, in combination with electrical stimulation, is typically employed if malunion or osseous defects exist, inasmuch as electrical stimulation will not correct osseous malalignment or loss of bone substance (Fig. 89.2).
Bone grafts that may be augmented with electrical bone stimulation include autografts, allografts, and many of the bone substitute grafts that include porous hydroxyapatite.
INFECTION
Electrical stimulation has been used with success for the management of infected nonunions (3,52). Appropriate stabilization, as well as appropriate efforts to eradicate the microorganisms, must be concurrently employed as necessary. Three problems exist that need to be addressed in managing an infected delayed union or nonunion, and these include the infection itself, the osseous instability, and the hindrance of osteogenesis. Management of the infection and the creation of osseous stability, along with the use of a bone stimulator for enhanced osteogenesis, create a more optimized environment for healing an infected nonunion.
In addition to the augmentation of osteogenesis, electrical stimulation may accelerate the resolution of infection by other means. Electrical stimulation has been noted to result in reduction, change in character, and resolution of drainage at the site of an infected nonunion (11). Bone grafting combined with electrical stimulation has been demonstrated to successfully resolve the signs and symptoms associated with infected nonunions (49,53,54). If an infection is present or suspected, a noninvasive PEMF or CMF device may be preferable to an implantable one. Use of PEMF therapy has been shown to increase lymphocytic response in vitro and in vivo, regulating inflammatory response (55,56,57 and 58).
ARTHRODESIS
A recent prospective study utilizing PEMF stimulation in a patient population that included primary and revisional arthrodesis, showed an increased rate of radiographic union (59). Whether or not a bone stimulator is indicated in a primary fusion remains debatable, but in all subgroups studied, the time to radiographic union was significantly decreased.
Patients who have reduced healing potential, such as those in an immunocompromised state due to comorbidities and/or steroid usage, and those with impaired vascularity due to peripheral vascular disease or tobacco use, could benefit from an adjunctive device. Nicotine abuse has been shown to cause a decreased rate of bone healing, in addition to complications during healing. In assessing the healing of distal radial and tibial fractures in nonsmoking and smoking patients who either used a low-intensity, pulsed ultrasonic device or a placebo device, there were no statistical differences found in healing time between smokers and nonsmokers who used the active ultrasound device (60).
Arthrodesis procedures are common salvage procedures used in Charcot deformity, arthritic conditions, and failed nonarthrodesis surgeries. Unfortunately, many of the patients undergoing arthrodesis are high-risk patients with past medical histories that prove a challenge for bone fusion. Some conditions include diabetes mellitus, rheumatoid arthritis or connective tissue disorders, peripheral vascular disease, obesity, smoking, immunosuppressive therapy, and alcoholism. In these instances, it may be beneficial to primarily use an implantable bone stimulator during the initial arthrodesis surgery (61).
REVISIONAL (FAILED) ARTHRODESIS
Electrical stimulation may be used to resolve symptoms associated with failed arthrodesis procedures, with a reported success rate of approximately 85% (62,63). The most important aspect in the use of electrical stimulation for failed arthrodesis is to identify the delayed union or nonunion and to take appropriate steps to reinitiate the reparative process. If the arthrodesis site is failing, this must be identified in such a way as to classify it as a delayed union, partial union, or complete nonunion site. Identifying where any fibrocartilaginous nonunion has occurred is equally important since surgical reduction and revision may be necessary prior to using electrical bone stimulation. Electrical bone stimulation can be either a primary treatment or a secondary
adjunctive treatment for a failed arthrodesis of the foot or ankle. The delayed union or nonunion must be hypertrophic (i.e., not an atrophic nonunion), and capable of potential healing, for electrical bone stimulation to be considered alone. If the delayed union or nonunion is atrophic and/or not capable of healing (e.g., due to the gap distance present), then surgical treatment must be performed, and if desired, combined with invasive or noninvasive electrical bone growth stimulation (Fig. 89.3). In a small case series, using an implantable bone stimulator as an adjunct to rigid external fixation in revisional arthrodesis procedures led to fusion in all 10 patients studied. While the success of fusion cannot be attributed solely to the use of bone growth stimulation, the study also showed that no patients had complications or problems arising from the implantable stimulator (64).
adjunctive treatment for a failed arthrodesis of the foot or ankle. The delayed union or nonunion must be hypertrophic (i.e., not an atrophic nonunion), and capable of potential healing, for electrical bone stimulation to be considered alone. If the delayed union or nonunion is atrophic and/or not capable of healing (e.g., due to the gap distance present), then surgical treatment must be performed, and if desired, combined with invasive or noninvasive electrical bone growth stimulation (Fig. 89.3). In a small case series, using an implantable bone stimulator as an adjunct to rigid external fixation in revisional arthrodesis procedures led to fusion in all 10 patients studied. While the success of fusion cannot be attributed solely to the use of bone growth stimulation, the study also showed that no patients had complications or problems arising from the implantable stimulator (64).
CHARCOT JOINT DISEASE
Electrical stimulation has been reported as effective in the management of neuropathic (Charcot) joint disease associated with diabetes mellitus or alcoholic neuropathy (41,65,66,67 and 68). Traditionally, acute Charcot osteoarthropathy is treated with rigid immobilization and edema reduction measures. These modalities may potentially be enhanced with the use of bone growth stimulation and used as an adjunct in this way; bone growth stimulation may result in a more expedient reduction of joint inflammation and edema and earlier foot stability (69). Hanft et al (66) performed a study with patients using CMF stimulation in patients with acute Charcot arthropathy, and consolidation occurred 12.8 weeks sooner in the group receiving bone growth stimulation than in the control group.
Charcot joint disease is characterized by progressive bone resorption and increased osteoclastic activity, with both processes reduced by electrical stimulation of bone because of a decrease in the cellular response to parathyroid hormone (70). In addition, improvement in ulcer healing rates and sensation related to electrical field-triggered alterations in neural regeneration and axon transport rates have been reported (71,72 and 73).
Low-intensity ultrasound has also been proposed as an adjunctive treatment for patients with acute Charcot osteoarthropathy based on its positive supportive role in healing a limited number of reported cases (74).
AVASCULAR NECROSIS
A variety of osteonecroses may affect the foot and ankle, including posttraumatic or nontraumatic avascular necrosis of the talus, second metatarsal head (Freiberg disease), navicular (Köhler disease), or dysvascular change after any fracture or osteotomy. Electrical stimulation of bone has been shown to increase angiogenesis while promoting osteogenesis. In addition, electrical bone growth stimulation diminishes the osteoclastic resorption of bone associated with osteonecrosis, which is responsible for the bone collapse and fragmentation.
Electrical stimulation has been shown to be an effective, conservative alternative to arthroplasty for the management of avascular necrosis of the hip. A significantly higher percentage of success was found in patients treated with PEMF stimulation rather than surgical decompression. PEMF therapy, by decreasing bone resorption and therefore maintaining bone mass, was more successful in preventing collapse of the femoral head and preventing further progression of the condition (75,76,77,78 and 79). Its use may be helpful following distal first metatarsal surgery with suspected avascular necrosis of the capital fragment, thus potentially avoiding the development of overt collapse and deformity, at which point resection arthroplasty or arthrodesis may be necessary.
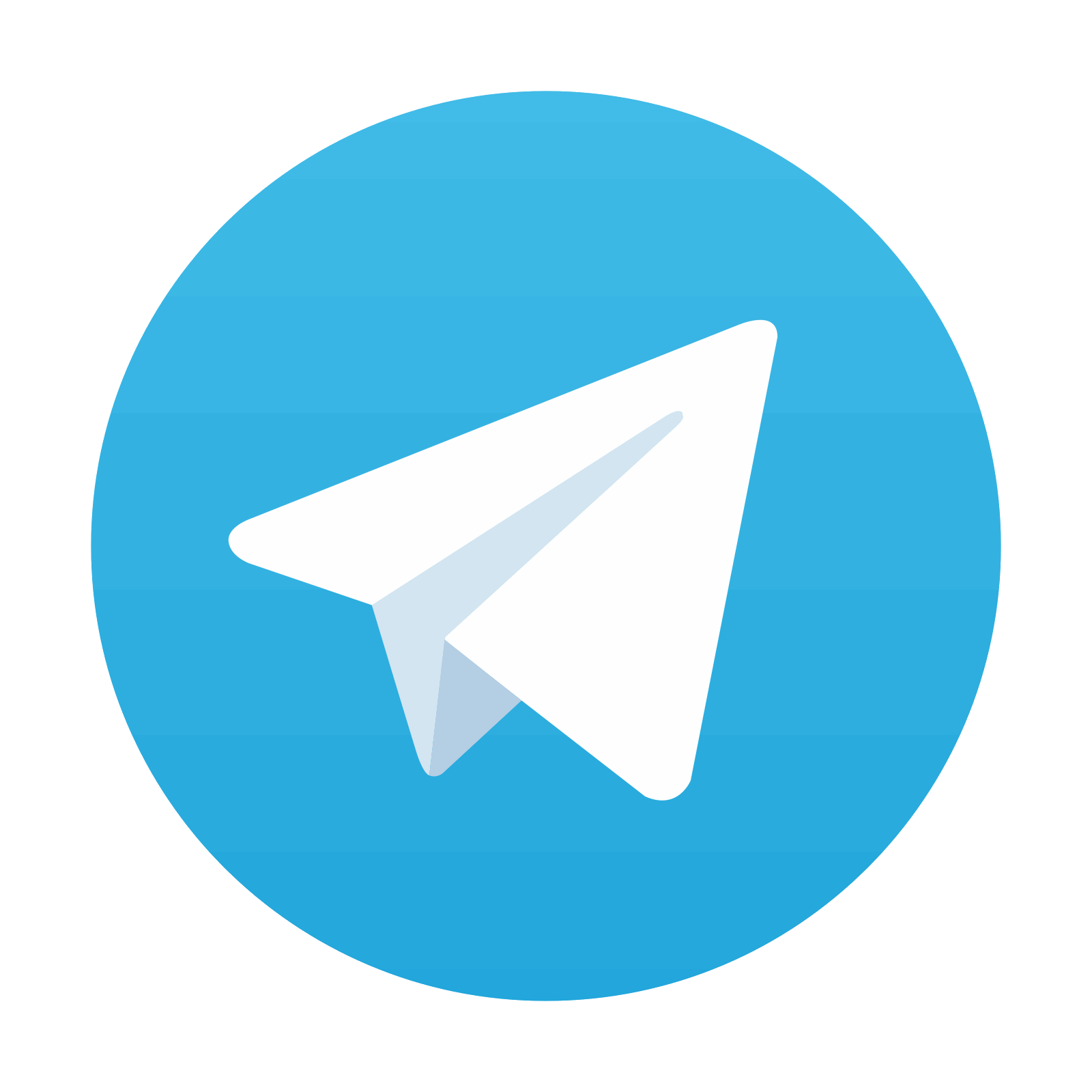
Stay updated, free articles. Join our Telegram channel

Full access? Get Clinical Tree
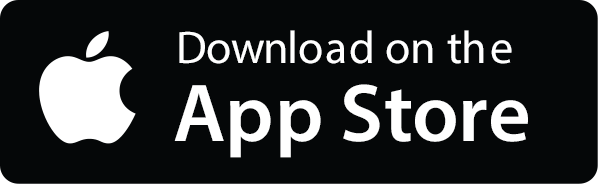
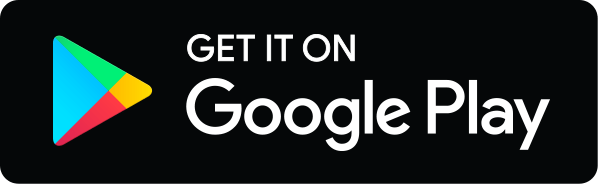
