, Michael GallagherJr.2 and Robert J. Robertson3
(1)
Lock Haven University of Pennsylvania, Lock Haven, PA, USA
(2)
University of Central Arkansas, Conway, AR, USA
(3)
University of Pittsburgh, Pittsburgh, PA, USA
An ergogenic aid is defined as a substance, technique or device that directly improves exercise performance or indirectly removes constraints to exercise performance. Caffeine is one such substance and is a proven ergogenic aid for performance during endurance, load-incremented, short-term high-intensity and resistance exercise modalities. The primary mechanism of action seemingly responsible for caffeine’s ergogenic effect may be due to its antagonism of receptors for the neurotransmitter adenosine. Biochemically blocking adenosine receptors may have widespread effects on the body as a result of an increase in dopamine secretion. Dopamine increases attention, memory, motivation, and feelings of reward. Of importance to the present chapter, appropriate doses of caffeine can affect exercise performance while decreasing perceived exertion. All of these responses to caffeine ingestion can potentially improve exercise performance and PA adherence. In addition, blocking adenosine can decrease the activation of nociceptors, resulting in a blunted pain response to exercise. This chapter presents recent evidence for the ergogenic effects of caffeine during exercise as they pertain to perceived exertion, pain and affective responses. In addition, selected laboratory applications of the perceptual methodologies presented in previous chapters are proposed for use in studying the ergogenic effects of caffeine ingestion during exercise.
15.1 Ergogenic Effect of Caffeine
Studies have shown that caffeine ingestion in amounts ranging from 2 to 10 mg/kg body mass has resulted in significant improvements in endurance, load-incremented, short-term high-intensity and resistance exercise performance (Astorino and Roberson 2010; Doherty and Smith 2004). A meta-analysis by Doherty and Smith (2004) examined the results of 40 different studies that compared the ergogenic properties of caffeine ingestion to a placebo condition. A placebo control design is important because consumption of a caffeine free placebo (i.e., solid or liquid) alone may improve exercise performance (Beedie et al. 2006). Overall, caffeine improved test outcome by 12.3 % when data from all exercise types were pooled, including endurance exercise, graded exercise tests and short-term high intensity exercise. A significantly greater ergogenic effect was found for endurance exercise compared with graded exercise tests and short-term high intensity exercise when reasonably similar caffeine doses were employed. In addition, the greatest improvement in endurance exercise was found for time-to-exhaustion protocols, usually performed at intensities ranging from 75 to 85 % of VO2max/peak as compared with performance for a given time or distance (Doherty and Smith 2004). However, time-to-exhaustion protocols are not similar to the common demands found in sport and therefore, have a low ecological validity compared to time- or distance-trials. In addition, the reliability of time and distance protocols has been found to be greater than time-to-exhaustion tests (Doyle and Martinez 1998; Jeukendrup et al. 1996; Laursen et al. 2003; Marino et al. 2002; Schabort et al. 1998a, 1998b).
A systematic review by Astorino and Roberson (2010) examined the results of 28 investigations that studied the effects of caffeine ingestion on short-term high intensity exercise and resistance exercise performance. Of 17 studies involving short-term high intensity exercise, which included mostly sprinting and power-based performances, 11 found significant improvements with caffeine ingestion. Of 11 studies involving resistance exercise, 6 found significant performance improvements (Astorino and Roberson 2010). Overall, the literature reveals strong agreement that caffeine has an ergogenic effect on endurance exercise. Whereas studies of short-term high intensity exercise and resistance exercise have had mixed results regarding the ergogenic properties of caffeine ingestion.
15.2 Mechanisms for the Ergogenic Effects of Caffeine
15.2.1 Glycogen Sparing
An underlying mechanism for the ergogenic effect of caffeine ingestion on endurance exercise performance involves glycogen sparing. Caffeine increases plasma epinephrine concentration (Arciero et al. 1995; Graham and Spriet 1995; Robertson et al. 1981; Van Soeren et al. 1993) which can result in an increased release of free fatty acids (FFA) from adipose tissue triglycerides. An increase in FFA availability for skeletal muscle metabolism, then, should increase fat use as an energy substrate, sparing muscle glycogen (Costill et al. 1978; Essig et al. 1980; Ivy et al. 1979).
In contradiction to this line of thinking, studies have shown that both caffeine ingestion and increased plasma epinephrine do not always result in increased plasma FFA (Arogyasami et al. 1989a; Graham 2001; Graham and Spriet 1995; Winder 1986). In addition, while muscle glycogen sparing following caffeine ingestion has been shown in some studies (Erickson et al. 1987; Essig et al. 1980; Spriet et al. 1992), the findings of most investigations contradict this theory (Arogyasami et al. 1989a, 1989b; Chesley et al. 1995, 1998; Graham et al. 2000; Graham 2001; Greer et al. 2000; Jackman et al. 1996; Laurent et al. 2000; Raguso et al. 1996; Roy et al. 2001). Graham and colleagues (2008) pooled data from multiple studies involving human subjects (Chesley et al. 1998; Erickson et al. 1987; Essig et al. 1980; Graham et al. 2000; Greer et al. 2000; Jackman et al. 1996; Laurent et al. 2000; Spriet et al. 1992) to achieve an overall sample size of 37 subjects from whom muscle glycogen content during exercise was measured. The compiled data found no significant glycogen sparing due to caffeine ingestion. Other studies have found no overall effect of an increase in plasma epinephrine on endurance exercise performance (Graham and Spriet 1995; Kovacs et al. 1998).
Potential reasons for the foregoing conflicting results are that an increase in plasma epinephrine has been shown to increase muscle glycogen breakdown. This action counteracts the effect of increased FFA availability, and increases the production of lactate, a progenitor of muscle fatigue (Arogyasami et al. 1989a; Kovacs et al. 1998; Jackman et al. 1996; Laurent et al. 2000). In addition, although most evidence shows no significant effect of caffeine on carbohydrate or fat metabolism within skeletal muscle, marked interindividual differences have been found. These data indicate that some individuals seem to be responders to caffeine and/or epinephrine resulting in glycogen sparing while most individuals are non–responders (Battram et al. 2007; Chesley et al. 1998; Graham et al. 2008; Martin et al. 2006).
15.2.2 Adenosine Antagonism
The primary mechanism of action of caffeine that results in its ergogenic effects is the blocking of receptors for the neurotransmitter adenosine (Davis et al. 2003; Fredholm et al. 1999). Structurally, adenosine is classified as a purine along with a larger molecule of which it is a component, adenosine triphosphate (ATP) (Marieb and Hoehn 2013). Adenosine alone is a potent inhibitor of neurotransmission in the brain and can have widespread effects throughout both the central and peripheral nervous systems (Marieb and Hoehn 2013). These include decreasing the release of neurotransmitters such as dopamine and decreasing overall brain arousal (Davis et al. 2003; Fredholm et al. 1999). While adenosine increases in skeletal muscle and the blood with muscular contraction (Davis et al. 2003), the ingestion of caffeine can counteract its effects, thereby allowing continued release of dopamine and heightened arousal.
Dopamine is known for its involvement in attention, memory, motivation and reward (Meeusen et al. 2006a, 2006b). By extension, it is likely that dopamine plays an important role in exercise performance. In fact, three studies have shown that pharmacologically inhibiting the reuptake of dopamine results in an increased dopamine concentration and improved endurance exercise performance (Bridge et al. 2003; Roelands et al. 2012; Watson et al. 2005). Therefore, the effect of caffeine on dopamine may allow increased attention and memory, leading to more accurate pacing strategy, as well as increased motivation and feelings of reward, all of which can contribute singularly or collectively to enhance exercise performance capacity (Roelands et al. 2013).
The ergogenic effect of caffeine has been attributed to its ability to blunt perceptual responses to exercise, as measured by RPE and naturally occurring muscle pain. The attenuating effect of caffeine on both RPE and muscle pain may be due to its antagonism of adenosine receptors. First, adenosine antagonism may allow an increased secretion of dopamine (Davis et al. 2003; Fredholm et al. 1999). Dopamine has been found to be inversely related to central fatigue during exercise, possibly because of its ability to counteract the lethargy and tiredness caused by elevated levels of serotonin (Davis and Bailey 1997). Studies have shown that pharmacologically blocking dopamine reuptake improved the intensity of exercise as performance without concomitant increases in the RPE response (Watson et al. 2005; Roelands et al. 2012). In addition, studies of repeated submaximal isometric contractions postulated that caffeine ingestion improved time to fatigue through an attenuation of force sensation, not through alterations in neuromuscular contractile properties (Meyers and Cafarelli 2005; Plaskett and Cafarelli 2001). Therefore, caffeine may delay the onset of fatigue and attenuate the perception of force, blunting the RPE response to exercise.
Second, adenosine antagonism may decrease the activation of nociceptors, i.e., pain receptors. Adenosine is one of many chemicals that can activate nociceptors in both the central and peripheral nervous systems (Sawynok and Liu 2003). Its increase during exercise naturally produces muscle pain, similar to hydrogen ions, bradykinin, and substance P, among others (O’Connor and Cook 1999). However, caffeine does not have a known effect on these chemicals. Therefore, caffeine may delay the onset of naturally occurring muscle pain and blunt the pain response to exercise as the intensity and/or duration of the performance increases. Improvements in exercise test performance and concomitant attenuation in the RPE and/or pain response, both owing to caffeine ingestion, have been shown during endurance (time-to-exhaustion and time-trials) (Backhouse et al. 2011; Cole et al. 1996; Demura et al. 2007; Doherty and Smith 2005; Gliottoni and Motl 2008; Hadjicharalambous et al. 2006; Ivy et al. 1979; Jenkins et al. 2008; Laurence et al. 2012; Motl et al. 2006; O’Connor et al. 2004) and resistance exercise (Bellar et al. 2011; Green et al. 2007; Hudson et al. 2008).
15.3 Effect of Caffeine on RPE During Exercise
A meta-analysis by Doherty and Smith (2005) tested the results of 21 studies that compared RPE between caffeine ingestion and placebo conditions during constant load exercise or following exhaustive exercise. The constant-load exercise protocols in these investigations generally required performance at intensities between 50 and 80 % VO2max/peak. For constant load exercise, RPE was significantly lower in the caffeine than placebo condition by an average of 5.6 %. This corresponded to an average increase of 11.2 % in exercise test performance. Regression analysis revealed that the mean exercise RPE explained 29 % of the variance in the difference in performance between caffeine and placebo conditions. Therefore, it seems that caffeine blunts the RPE response during constant load exercise, allowing individuals to exercise for a longer period of time before subjective fatigue becomes intolerable (Doherty and Smith 2005). This is in agreement with investigations that have studied the effect of caffeine during exercise performed at a constant RPE, i.e., using a perceptual production protocol, rather than a constant workload. Investigations by Ivy et al. (1979) and Cole et al. (1996) found that subjects chose to exercise at higher intensities after ingesting caffeine compared to a placebo condition, yet subjects were instructed to self-regulate exercise intensity at the same target RPE for both exercise conditions. Another interesting finding of the meta-analysis conducted by Doherty and Smith (2005) was that RPE did not differ between caffeine and placebo conditions following exhaustive exercise. This perceptual response at the end of exhaustive exercise is intuitive, given that the individuals were tasked to perform the exercise to the point of complete exhaustion necessitating exercise termination. By definition, such exhaustive exercise should result in a maximal or at least near maximal RPE.
More recent investigations have continued to support the relation between caffeine and reduced perceptions of fatigue during both endurance and resistance exercise (Backhouse et al. 2011; Demura et al. 2007; Green et al. 2007; Hadjicharalambous et al. 2006; Hudson et al. 2008; Laurence et al. 2012). Demura and colleagues (2007) studied the effect of 6 mg/kg caffeine ingestion on physiological variables and RPE during 60 min of submaximal endurance cycling at 60 % VO2peak. The only difference between the caffeine and placebo conditions was a significantly lower RPE at a given submaximal cycle PO as a result of caffeine ingestion (Demura et al. 2007).
Hadjicharalambous and colleagues (2006) studied the effect of 7–7.5 mg/kg caffeine ingestion on differentiated RPE (legs and chest/breathing) and performance during both constant load (73 % VO2max) and incremental exercise after consumption of a high fat meal in endurance trained men. The purpose of the high fat meal was to remove the potential ergogenic effect of an increase in FFA, cited earlier as a possible result of caffeine supplementation. Elevated FFA concentration produces glycogen sparing and subsequently increases exercise performance. However, it is important to note that such a response may only be seen in certain individuals (Battram et al. 2007; Chesley et al. 1998; Graham et al. 2008; Martin et al. 2006). In the investigation by Hadjicharalambous et al. (2006), results demonstrated a significantly lower RPE for the legs during both exercise tests and a significantly lower RPE for the chest/breathing during incremental exercise in the caffeine condition only. However, performance was not improved by caffeine supplementation (Hadjicharalambous et al. 2006).
Laurence and colleagues (2012) studied the effects of 6 mg/kg caffeine ingestion on maximal 30-min cycling performance, RPE and RER in sedentary men. Performance (i.e., total work) was significantly greater after caffeine ingestion compared to the placebo condition. RPE and RER were similar between trials across time-points. The improved performance and similar RPE indicate that consequent to caffeine ingestion, an increase in work rate was not accompanied by a corresponding increase in RPE. The men were able to accomplish a higher intensity while reporting the same level of perceived exertion. In addition, by measuring RER, the investigators were able to examine potential changes in energy substrate utilization subsequent to caffeine ingestion. The similar RER between the two work rates may indicate that the relative contribution of carbohydrate and fat as fuel for exercising muscle was similar between experimental conditions even though the work rates were different (Laurence et al. 2012).
Green and colleagues (2007) studied the effects of 6 mg/kg caffeine ingestion on resistance exercise performance (bench press and leg press) and differentiated RPE (specific to active muscles) in men and women. Caffeine ingestion resulted in significantly greater performance for leg press exercise as indicated by an increased number of repetitions to failure at a 10RM resistance. The size of this ergogenic effect was similar to that reported by Laurence et al. (2012) for a 30-min cycling performance. The increased performance for leg press exercise was not accompanied by an increase in RPE, indicating a delay in fatigue induced by caffeine. However, these results were not found for bench press exercise (Green et al. 2007).
Two investigations studied the effects 5 and 10 mg/kg caffeine ingestion on moderate intensity cycling performance (60 % VO2peak) and leg muscle pain in subjects who reported low habitual levels of caffeine consumption. One investigation employed males as subjects (O’Connor et al. 2004), and the other employed females (Motl et al. 2006). Both investigations revealed significant decreases in leg muscle pain ratings during exercise after caffeine ingestion (5 and 10 mg/kg) compared to placebo measurements, without any changes in such physiological variables as BP, HR, and VO2. In addition there was no statistically significant difference in leg muscle pain between the 5 and 10 mg/kg caffeine conditions. In the study by O’Connor et al. (2004), each male subject rated perceived pain lower during the 10 mg/kg condition compared to the 5 mg/kg condition, yet the overall group mean difference was not statistically significant. These results indicate there may be a dose–response relation between caffeine ingestion and the attenuation of exercise-induced muscle pain in males. Another investigation compared ingestion of 5 mg/kg of caffeine to a placebo condition during high intensity cycling (80 % VO2peak) in women. Leg muscle pain was significantly lower during the caffeine than placebo conditions (Gliottoni and Motl 2008). The size of this caffeine-induced ergogenic effect was similar to that reported for cycle performance at 60 % VO2peak intensity (Motl et al. 2006; O’Connor et al. 2004).
15.4 Effect of Caffeine on RPE and Pain During Exercise
A number of investigations studied both RPE and pain responses to exercise following caffeine ingestion (Astorino et al. 2011; Hudson et al. 2008; Jenkins et al. 2008). Jenkins and colleagues (2008) examined the effects of 1, 2 and 3 mg/kg caffeine on cycling performance, RPE (measured for the overall body, legs and chest/breathing) and muscle pain in trained men. The exercise trial involved 15 min of cycling at 80 % VO2peak followed by 4 min of active recovery. The initial phase of the protocol was followed by a second phase whereby exercise progressed sequentially to maximal performance in a 15-min time trial that simulated the end of a race (Jenkins et al. 2008). Cycling performance was improved following ingestion of 2 and 3 mg/kg caffeine compared to placebo with no effects on RPE (differentiated and undifferentiated) or muscle pain (Jenkins et al. 2008). Hudson and colleagues (2008) studied the effects of 6 mg/kg caffeine on light resistance training performance (leg extension and arm curl), RPE and active muscle pain perception. Caffeine ingestion resulted in significantly greater performance compared to a placebo condition without increases in RPE or pain (Hudson et al. 2008). Astorino and colleagues (2011) studied the effects of 2 and 5 mg/kg caffeine ingestion during high intensity isokinetic knee extension and flexion exercise. Although 5 mg/kg caffeine resulted in significant improvements of muscle function (i.e., peak and average torque, total work, PO), neither RPE nor pain responses were affected by supplementation (Astorino et al. 2011). The results of all three studies indicate blunted perceptual responses (both RPE and pain) with increased work rates and performance as a result of caffeine ingestion (Astorino et al. 2011; Hudson et al. 2008; Jenkins et al. 2008).
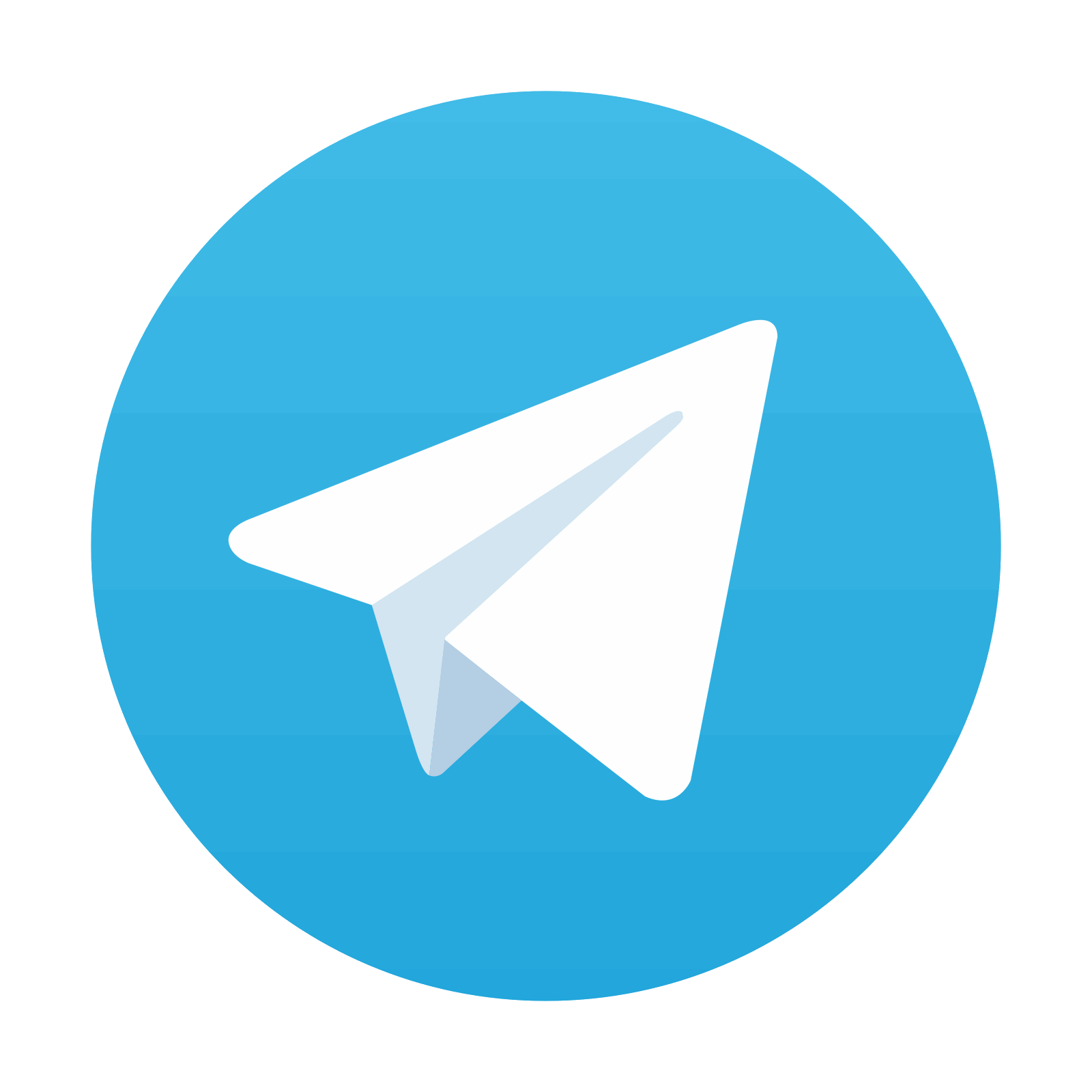
Stay updated, free articles. Join our Telegram channel

Full access? Get Clinical Tree
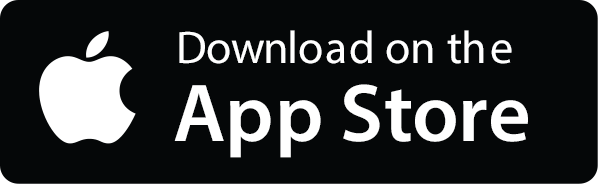
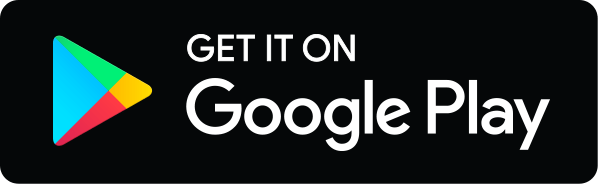