, Michael GallagherJr.2 and Robert J. Robertson3
(1)
Lock Haven University of Pennsylvania, Lock Haven, PA, USA
(2)
University of Central Arkansas, Conway, AR, USA
(3)
University of Pittsburgh, Pittsburgh, PA, USA
The achievement of optimal performance during exercise is not a new area of interest among athletes, trainers, and coaches. Many tend to employ external performance enhancing devices or substances, such as improved clothing and footwear, nutritional beverages and high caloric (i.e., energy) shakes. However, those that solely rely on such external mechanisms to improve performance may still be limiting the potential of their athletes to achieve the absolute upper limits of a specific exercise endeavor. Regardless of the type of clothing, footwear, or ergogenic beverage, the athlete’s psychological and/or physiological capacities to attain and maintain maximal loads provides the greatest contribution to reach the upper limits of exercise performance. Information gained from these interrelated psychophysiological mechanisms, known collectively as teleoanticipation, incorporate memory from previously completed exercise along with both centrally generated efferent signals and peripheral afferent signals during exercise in order to minimize fatigue and obtain optimal performance. The assessment of an individual’s just noticeable difference (JND) in perceived exertion may assist in providing appropriate feedback during exercise intensity self-regulation to improve pacing strategy. The perceived exertion JND is a measure of an individual’s perceptual acuity, i.e., the smallest change in exercise intensity (expressed as VO2 or PO) that elicits a noticeable change in perceived exertion. The perceived exertion JND can be applied to the improvement and refinement of teleoanticipation to attain optimal exercise performance. The primary purpose of this laboratory experiment is to examine the use of the perceived exertion JND in a traditional RPE-based exercise prescription. Using the JND as identified in an estimation–production paradigm can in turn provide feedback during the production protocol regarding the accuracy of intensity self-regulation according to a target RPE.
11.1 Background
11.1.1 Pacing Strategy and Teleoanticipation
The study of exercise performance pacing, and associated pacing strategies, arose from research into the mechanisms of fatigue during exercise. It was concluded that an optimal pacing strategy should reduce muscular fatigue by setting performance intensities that optimize both physiological and psychological responses to muscular exertion. It is on this previously established reference point that teleoanticipation plays a critical role in dictating exercise performance in the upcoming and/or ongoing task. Teleoanticipation uses the individual’s cognitive ability to recall the stored information regarding metabolic and biomechanical responses to a previously performed exercise task (Ulmer 1996). This recalled information provides a cognitive reference for periodic adjustment in exercise pace throughout the performance, especially at the outset. Once exercise is underway, afferent signals provide feedback on the “state of the body” to obtain optimal performance for that given task, signaling the need to maintain or adjust performance pace. The closer to optimal performance an athlete is at the beginning of exercise, the better the overall performance for that task throughout the duration of the event.
The teleoanticipation process is analogous to looking at someone to provide a general estimate of their age. The evaluator’s collective memory of individuals, their age, and appearance accrued over the lifespan are used to provide a cognitive estimate of the age of the target individual. Feedback and subsequent storage of this information is obtained through a series of queries aimed at identifying the exact age of the target individual. This process of using stored information provides a more realistic estimate of age for future estimates of individuals possessing the same general characteristics.
Much of the research and support for teleoanticipation as a mechanism for determining a pacing strategy utilized a perceptual estimation–production paradigm. Additionally, performance pacing strategies are partially dependent on the duration of the task and follow one of three possibilities: positive, negative, or even pacing (Abbiss and Laursen 2008). A positive pacing strategy is commonly observed in events lasting less than 2 min in which an athlete initially starts out at an intensity faster than metabolically optimal, but then the speed or PO is systematically decreased with time. A negative pacing strategy employs an increase in performance pace or producing a higher PO as the task progresses. An even pacing strategy occurs when the athlete performs the task at a preset constant speed or PO throughout the duration of the task.
11.1.2 Mechanisms of Different Pacing Strategies
The three commonly employed pacing strategies may be dependent upon the availability, type and magnitude of metabolic and biomechanical feedback. Tasks that are short-duration may not last long enough to provide sufficient time for the afferent signals to be processed and thereby relying more on the stored memories of that task. These events indicate positive pacing strategies, i.e., a sprinter “bursts” out of the blocks at high speed and the speed tends to slow down towards the end of the sprint. Additionally, the decline in substrate availability of ATP, phosphocreatine, and intramuscular glycogen regulate the rate of muscular fatigue for exercise tasks lasting between 1 and 30 min. Conversely, increases in core body temperature appear to contribute greatly to the onset and progression of muscular fatigue in exercise tasks lasting between 20 and 120 min. Likewise, a decline in intramuscular carbohydrate as an energy source contributes greatly to muscular fatigue in tasks lasting greater than 90 min (de Koning et al. 2011).
11.1.3 Pacing Strategies and RPE
As such, one marker of the integration of the psychological “exercise template” (St Clair Gibson et al. 2005) and physiologic feedback involving energy substrate evaluation is the overall rating of perceived exertion (RPE). An even pacing strategy, as measured by speed, power output, and/or RPE, is thought to provide an optimal performance level throughout the competitive event. Such a pace can forestall the onset of muscular fatigue by using stored information regarding previously performed pace and resulting metabolic and biomechanical feedback that occurred during a similar previously performed task. Therefore, the exercise duration and the pre-rehearsed “exercise template” that guides pace using in-task sensory feedback is continually updated with each subsequent repetition. The resulting pacing strategy for a competitive event is a composite of preset signals from a teleoanticipation sensory feedback system that is continually updated based on the effectiveness of the in-task competitive strategy (Hettinga et al. 2011).
Undifferentiated RPE (overall or whole-body) has been employed in many studies examining pacing strategies as linked to underlying teleoanticipation mechanisms. These studies used match–mismatch exercise paradigms or pharmacological interventions to selectively block afferent signals arising from active muscles and joints during exercise (Amann et al. 2009; Roelands et al. 2009, 2013). Match–mismatch exercise paradigms involve participants that are informed they would perform a predetermined number of trials at the same intensity and duration (Baden et al. 2005) or distance (Albertus et al. 2005). In reality, the paradigm was designed to deceive the participants into believing they exercised at different durations or distances. This was done by masking the participants to correct visual or verbal feedback regarding the performance.
Baden et al. (2005) had participants complete three trials at the same intensity and duration, in random order: (1) a 20 min run, (2) a 10 min run, but then with 1 min remaining subjects were instructed to run another 10 min (20 min total), and (3) a run of an unspecified duration, but subjects were instructed to stop at 20 min. Albertus et al. (2005) had participants complete four separate 20-km exercise time trials. However, the distance feedback was presented as: (1) correct, (2) under-reported, (3) over-reported, and (4) randomly between 25 and 250 m for each 1-km split. Regardless of the distance feedback, participants completed the 20-km cycle ergometer time trial in similar times. Albertus et al. (2005) suggested that factors other than distance feedback may have been responsible for pacing strategies among the four trials. They further speculated that the anticipatory response, indicative of the predetermined “exercise template,” may have been more critical in setting the pace than the performance feedback during exercise. The support for teleoanticipation was evident in the last kilometer of each time trial in which the participants were still capable of increasing the power output suggesting that the participants utilized a “hardwired” motor-sensory mechanism to prevent the development of premature fatigue and subsequent loss of power or speed.
The results reported by Baden et al. (2005) and Albertus et al. (2005) suggest that perceived exertion is influenced by physiological changes during exercise. Therefore, this theory holds that prior to the exercise performance the anticipated intensity of the perceived level of exertion that will be experienced in-task is preset but subsequently fine-tuned on a moment-to-moment basis during exercise to ensure optimal performance. Lambert et al. (2005) summarizes the potential underlying mechanisms of teleoanticipation: “mechanoreceptor and metaboreceptor stimulation of the exercise reflex, perhaps initiated at the onset of exercise by mechanical feedback, and adjusted by chemoreceptor feedback according to the continuing energy demands of the muscle” (Lambert et al. 2005).
11.1.4 Just Noticeable Difference in Perceived Exertion
One method to optimize use of the “exercise template” containing the memory of preferred pacing strategies is to determine the just noticeable difference (JND). The JND reflects the acuity of human sensory processes. The concept of identifying a JND between isomorphic sensations of different intensities was explored by classic psychophysicists E. H. Weber and G. T. Fechner. These pioneers in experimental psychology sought to quantify the smallest detectable changes in physical stimuli that humans could perceive, i.e., the difference threshold, or JND (Noble and Robertson 1996; Wozniak 1999). Identifying the perceived exertion JND in an individual may improve the “exercise template” that guides pacing strategies, ensuring faster attainment of optimal performance. JND procedures identify physiological boundaries that define the intensity of an individual’s perceived exertion above and below a given exercise intensity. These boundaries are indicative of an individual’s perceptual acuity in the presence of changes in exercise intensity. Subsequent practice trials with feedback based on this JND range may allow an individual to more accurately self-regulate exercise intensity to achieve optimal performance strategies.
To measure the JND for a given type of sensation, a standard stimulus must first be determined. The standard stimulus represents a single point within the entire detectable range of stimuli. This point normally occurs somewhere between the stimulus threshold and terminal threshold, i.e., the lowest and highest perceived stimuli possible for that individual, respectively. The JND is then determined for that specific standard stimulus and is expressed as the smallest amount of change in the intensity of the stimulus that is required for that change to be perceived (Buckworth et al. 2013). The JND is determined for intensities that are both greater and less than the standard stimulus. It cannot be assumed that the JND above a standard stimulus is the same size as the JND below the same standard stimulus. In addition, it cannot be assumed that the JND determined for one standard stimulus is the same for other types of stimuli as perceived by any given individual.
11.1.5 Perceived Exertion JND Methodology
The JND for sensations such as hearing or touch can be measured easily using classic methods that involve multiple presentations of stimuli that are compared to the standard stimulus, each referred to as a comparative stimulus. For example, using the Method of Constant Stimuli, after presentation of the standard stimulus, a number of comparative stimuli are presented in random order and each one is presented multiple times. For each comparative stimulus the subject is asked whether it is similar to or different from the standard. The JND is calculated as the comparative stimulus that is perceived as being different from the standard 50 % of the time. The logic underlying their measurement proposes that the JND is not an absolute; rather it falls within a certain perceptual response range at any given time (Noble and Robertson 1996).
Classic psychophysical methods are not appropriate for determination of a perceived exertion JND. For senses such as hearing or touch, multiple comparative stimulus intensities can be presented without any residual or cumulative consequences that may change the individual’s overall perception of that sense. However, multiple presentations of various intensities of exercise may increase RPE, lead to fatigue, and hinder the ability to measure the perceived exertion JND (Haile et al. 2013). Therefore, methods unique to the determination of the perceived exertion JND have been designed. A recent investigation determined a perceived exertion JND for cycle ergometer exercise in young adults (Haile et al. 2013). The individual PO corresponding to an OMNI RPE of 5 derived from an estimation trial served as the SS. This RPE was chosen because it was previously shown to correspond to the lower limit of the perceptual zone encompassing the response-normalized RPE at the ventilatory threshold. The perceived exertion JND above (JND-A) and below (JND-B) the standard stimulus was determined using four separate 5-min cycle ergometer exercise bouts with rest periods between bouts. Exercise bouts 1 and 3 presented the standard PO. In exercise bouts 2 and 4, subjects were allowed to adjust the PO to produce the smallest detectable change in perceived exertion, either greater than the standard (for JND-A) or less than the standard (for JND-B). VO2 was measured during each bout. The change in VO2 between each JND bout and the previously presented standard stimulus bout was used as the aerobic metabolic analog to define the perceived exertion JND. In addition, physical units (i.e., PO) describing the JND were also determined (Haile et al. 2013).
11.1.6 Evidence for Perceptual Acuity
Perceptual acuity varies greatly between individuals for all human senses, including perceived exertion. For cycle ergometer exercise in young adults, the average perceived exertion JND-A was 5.90 %VO2peak with a standard deviation of 4.09 %VO2peak. This corresponded to an average change in PO required for detection of 8.4 W. The average JND-B was 8.33 %VO2peak with a standard deviation of 6.01 %VO2peak, corresponding to an average change required for detection of 15.6 W (Haile et al. 2013). This indicates that some individuals had comparatively fine perceptual acuity and sensed very small changes in the exertional level associated with changes in exercise intensity. Other individuals required more substantial changes in intensity before a noticeable difference in perceived exertion was detected. In addition, JND-A was significantly smaller than JND-B indicating that, on average, perceptual acuity was more precise just above an OMNI RPE 5 compared to just below an OMNI RPE 5. This indicates that the perceived exertion JND may change with exercise intensity or target RPE’s. Further research is needed to investigate the relation between the perceived exertion JND and exercise intensity, as well as the effects of fitness and PA levels. These potential differences in the perceived exertion JND, whether between exercise intensities in a single individual or between separate individuals of differing fitness or PA characteristics, may influence or even predict individual pacing strategy. Comparatively larger and smaller JND values may explain the appearance of positive and/or negative pacing strategies even when steady state pacing strategies may be considered the most optimal.
11.1.7 Perceived Exertion JND and Exercise Intensity Self-Regulation
A measurement of the perceived exertion JND can be used as an ideographic assessment of exercise intensity self-regulation error. A classic estimation–production paradigm analyzes prescription congruence by comparing physiological values that correspond to a specific RPE between estimation and production trials. This method does not take individual perceptual acuity into consideration. For example, VO2 values could be found significantly different between trials by a statistical analysis. However, an individual with less perceptual acuity and hence a greater perceived exertion JND would not be able to perceive them as different.
Therefore, the addition of the JND method to examine prescription congruence between estimation and production protocols can improve the validity of exercise intensity self-regulation error assessment. The VO2 values corresponding to JND-A and JND-B define a physiological range spanning an exercise intensity that corresponds to the target RPE for an exercise prescription. The VO2 range analogous to the perceived exertion JND identifies the limits of perceptual acuity of an individual for that exercise intensity. By definition, when an individual produces the target RPE, if VO2 values fall within the VO2 range for that mode specific JND then that individual is accurately self-regulating exercise intensity. Exercise intensity self-regulation error would be defined as physiological values that fall outside of the VO2 range corresponding to that perceptual JND when attempting to produce a prescribed target RPE.
The perceived exertion JND measures an individual’s perceptual acuity during physical exercise. The JND identifies those with fine and broad perceptual acuity corresponding to the same relative exercise intensity. In addition, determination of the perceived exertion JND allows precise feedback regarding accuracy of intensity self-regulation during production protocols. This feedback can provide an individual with the knowledge of whether or not exercise intensity self-regulation error is present, i.e., if the individual’s VO2 is within or outside of the analogous JND range. In addition, this feedback would provide the individual with information about which direction to adjust exercise intensity to correct self-regulation error. This additional step in the traditional estimation–production paradigm may be crucial for some individuals to accurately self-regulate exercise intensity using target RPE’s. Incorporating JND into exercise or fitness testing and training may provide a better-matched “exercise template” from which the individual may begin exercise and achieve an optimal pace to promote health-fitness gains. The improved “template” of the stored memory for the upcoming exercise may allow the individual’s body to efficiently and effectively reach the metabolic requirements for optimal exercise performance.
11.1.8 Case Study
11.1.8.1 Client Information
A 30-year-old female school teacher comes to your exercise physiology laboratory for follow-up exercise testing. Previously she performed maximal and submaximal exercise testing under your supervision upon entering an exercise program as part of her employer’s faculty health promotion program. The exercise testing involved both estimation and production procedures to test her ability to accurately self-regulate exercise intensity at a target RPE corresponding to the VT. The data from her production trial revealed that she seemed to have difficulty self-regulating exercise intensity. In this instance, she was demonstrating a significant amount of exercise intensity self-regulation error, as indicated by VO2 values quite dissimilar to that corresponding to the VT achieved during a maximal exercise test. Further examination of her ability to self-regulate exercise intensity is recommended to better allow her to achieve her goals for the exercise conditioning.
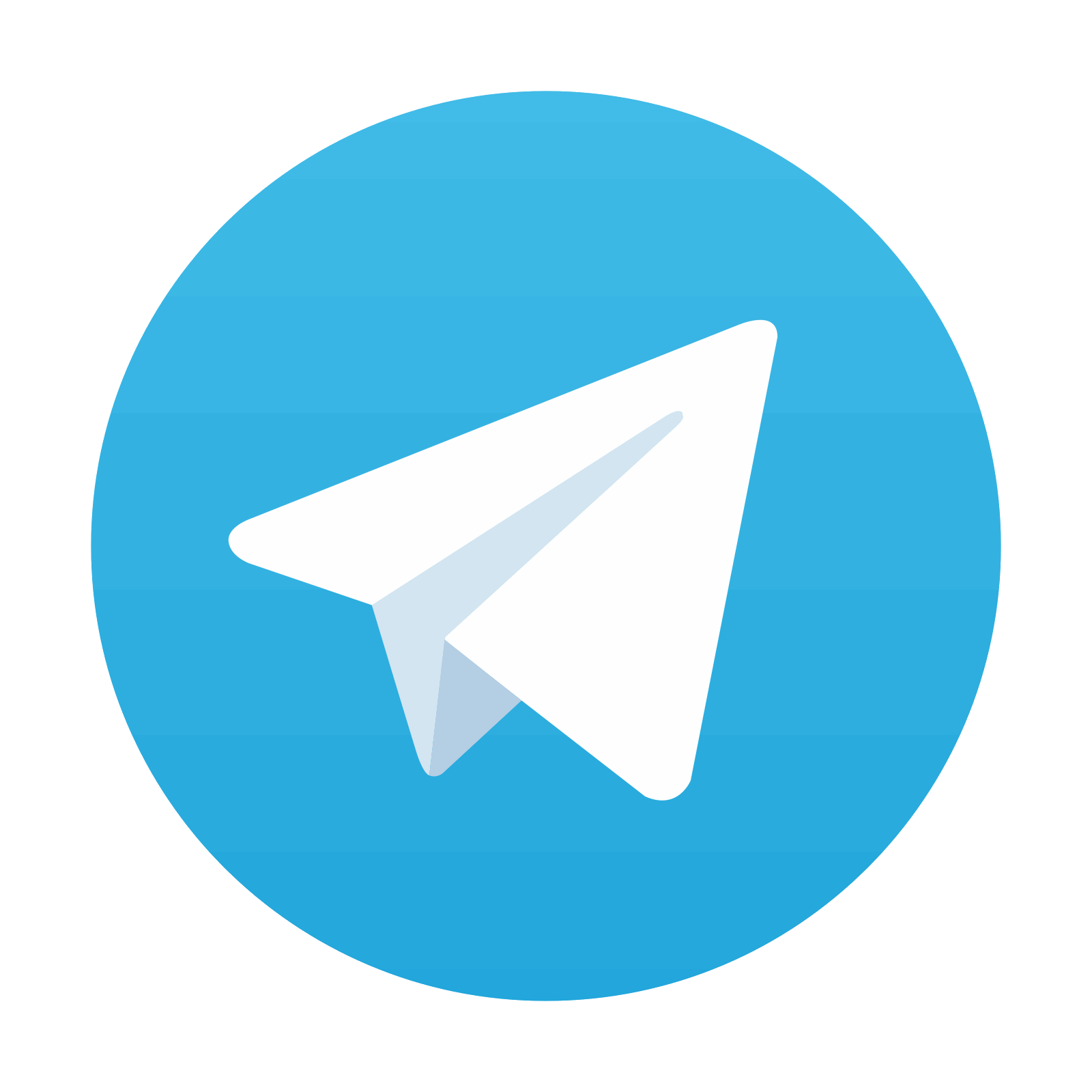
Stay updated, free articles. Join our Telegram channel

Full access? Get Clinical Tree
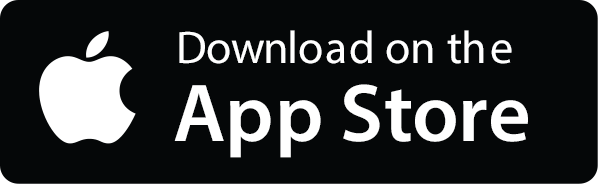
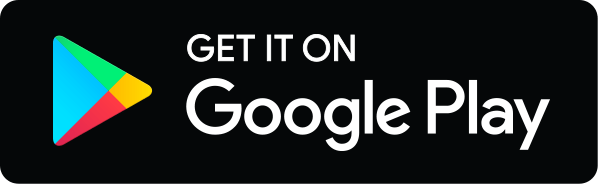
