, Michael GallagherJr.2 and Robert J. Robertson3
(1)
Lock Haven University of Pennsylvania, Lock Haven, PA, USA
(2)
University of Central Arkansas, Conway, AR, USA
(3)
University of Pittsburgh, Pittsburgh, PA, USA
Keywords
Cardiorespiratory fitnessMaximal oxygen consumption (VO2max)Graded exercise test (GXT)One-repetition maximum (1RM)Maximal HR (HRmax)Prediction modelCross-validationPerceptually-regulated exercise testCardiorespiratory fitness and muscular strength are important components of health-related physical fitness. The criterion measures for cardiorespiratory fitness and dynamic muscular strength are maximal oxygen uptake (VO2max) and one-repetition maximum (1RM), respectively. VO2max testing requires expensive laboratory equipment and expert personnel. Such maximal intensity testing may require physician clearance or supervision for older, sedentary and/or unfit individuals. 1RM testing requires the performance of multiple resistance exercise sets at near-maximal and maximal intensities and may not be safe for many individuals who are unfamiliar with resistance exercise or who have medical contraindications to high intensity exercise. Therefore, administration of maximal fitness test protocols may not be possible, pragmatic or even desirable in health-fitness and clinical settings. As such, submaximal tests have been developed to predict both VO2max and 1RM. It is proposed that the use of submaximal test protocols is both safe and cost-effective. Statistical models to predict VO2max have been based on the positive relation between HR and VO2 during load-incremented exercise. Models to predict 1RM have been based on the inverse relation between weight lifted and repetitions performed to the point of muscular fatigue. However, both VO2max and 1RM can be predicted from submaximal RPE. The use of RPE as a predictor variable in such tests is valid, technically simple, and easily understood by most individuals. In addition, submaximal fitness tests including the measurement of RPE can be used as an assessment of training-induced fitness changes. The primary purpose of this laboratory experiment is to predict VO2max/peak and 1RM from submaximal RPE measured during an estimation protocol exercise test.
8.1 Background
8.1.1 Assessment of Cardiorespiratory Fitness
Cardiorespiratory fitness determines an individual’s ability to perform dynamic exercise of a moderate to vigorous intensity using large muscle groups for a prolonged period. Cardiorespiratory fitness depends on the functional capacity of the cardiovascular and respiratory systems and the oxidative capacity of skeletal muscle (ACSM 2013). The criterion or “gold-standard” assessment of cardiorespiratory fitness is VO2max (i.e., maximal aerobic power), defined as the maximal amount of oxygen an individual can use during dynamic exercise while breathing air at sea level. The assessment of VO2max requires an individual to perform a load-incremented aerobic exercise protocol, or GXT, where pulmonary ventilation (V E) and expired concentrations of VO2 and VCO2 are determined using a respiratory-metabolic measurement system. For most clinically normal individuals, a valid VO2max can only be achieved during upright, weight-bearing, total body exercise such as uphill walking or running on a treadmill. The term VO2peak is used rather than VO2max when the measure is obtained using cycle ergometer exercise and other partial- or non-weight-bearing exercise modalities (e.g., swimming, elliptical ergometer, rowing ergometer, and arm ergometer). However, it is of note that when elite cyclists perform a load-incremented GXT on a cycle ergometer they often demonstrate a higher VO2max than for uphill treadmill exercise. It is important to assess an individual’s VO2max/peak with a mode-specific GXT that matches their exercise experience and the mode of exercise for which the prescription is intended. For most individuals, a treadmill protocol is appropriate.
VO2max and VO2peak tests allow determination of the VT. The VT is an important physiological marker for aerobic training intensity. At test intensities above the VT, an individual can no longer achieve steady state metabolic energy production. HR and/or RPE can be measured throughout a GXT. The HR or RPE values corresponding to specific physiological intensities (e.g., %VO2max or %VT) can then be used to prescribe exercise intensity. However, the ability to calculate target HR or RPE ranges based on VO2 responses to an exercise test comes with inherent costs and risks. First, the measurement and interpretation of respiratory-metabolic responses are time-consuming procedures that require expensive laboratory equipment and expert personnel that are not available in many clinics and fitness facilities. Maximal exercise testing often requires physician clearance and test supervision, especially when older, sedentary and/or unfit individuals are evaluated. This imposes additional financial and testing burden on the client and testing facility. Therefore, it is often not possible, practical or even desirable to perform a maximal test with respiratory-metabolic measurement to establish an individual’s cardiorespiratory fitness prior to undertaking an aerobic exercise program.
8.1.2 Assessment of Dynamic Muscular Strength
Muscular strength reflects the ability of a muscle or muscle group to exert force. Muscular strength can be assessed statically, involving isometric muscular action with no change in muscle length or joint angle, or dynamically, involving concentric and/or eccentric muscular contractions and changes in muscle length. Since assessments of static muscular strength are only specific to the joint angle used in testing, dynamic muscular strength is considered more ecologically valid. The criterion or “gold-standard” assessment of dynamic muscular strength is the 1RM test. The 1RM is used to establish the maximal amount of force an individual can exert during one repetition of single maximal effort using a defined muscle or muscle group. The lift must be performed in a controlled manner through the full range of motion with proper technique (ACSM 2013). This test requires that an individual perform multiple resistance exercise sets at near-maximal and maximal intensities using a progressive protocol. Unlike VO2max testing, most clinics and fitness facilities have ample resistance exercise equipment and educated personnel to assess 1RM strength. The primary concern for an individual who is undergoing a 1RM baseline test prior to beginning a resistance exercise program is safety. Many individuals who are beginning a resistance exercise program, including children and adolescents, have little to no experience with resistance exercise. In particular, they lack instruction on proper exercise technique. For these individuals, performing a 1RM test is not practical and may result in musculoskeletal injury. Even a multiple-RM test, where the goal is to perform a set number of repetitions ending at maximal intensity (e.g., 5RM or 10RM), may not be safe for many individuals. If a 1RM or multiple-RM test is employed, it may be best to guide the individual through a brief orientation and practice period that employs the exercise protocol prior to maximal testing.
8.1.3 Submaximal Tests to Predict Maximal Aerobic Power and Muscular Strength
Due to the methodological and safety limitations of measuring maximal aerobic power (i.e., VO2max) and muscular strength (i.e., 1RM), researchers have designed submaximal exercise tests from which maximal values can be predicted. The methods used to predict VO2max and 1RM are based on the relations between the criterion variable and predictor variable(s). For aerobic exercise, VO2 serves as the criterion variable since VO2max/peak is the unknown that is estimated by prediction models. For resistance exercise, weight lifted serves as the criterion variable since 1RM is the unknown that is estimated by prediction models. The predictor variables are physiological and/or physical markers that rise concurrently with increases in exercise intensity.
Traditional prediction models for aerobic exercise are based on the strong positive correlation between VO2 and HR that has been consistently demonstrated in the literature. The model of VO2 max using submaximal HR is dependent on the relative accuracy of age-predicted maximal HR (APMHR) equations (e.g., APMHR = 220 − age) and the positive relation between VO2 and HR as measured during a load-incremented protocol. The prediction can be presented graphically and/or determined using linear regression analysis. For a graphic determination, VO2 and HR data points from multiple submaximal exercise intensities are plotted on separate axes. HR, the predictor variable, is on the x-axis. VO2, the criterion variable, is on the y-axis. A line of best fit that describes the relation between the two variables is drawn by visual determination. The point where the line intercepts APMHR is extended laterally to the VO2 axis, identifying predicted VO2max. Using a linear regression model a line of best fit is calculated that describes the relation between HR and VO2 where these variables are expected to change as a function of increasing exercise intensity. This calculation yields an equation, VO2max = s(APMHR) + i. In this equation, s is the slope of the line and i is the y-intercept. One solves for VO2max by entering APMHR into the prediction equation.
Although HR is relatively easy to measure during aerobic exercise and most clinics and fitness facilities have appropriate technology and/or personnel, APMHR is not always the most accurate prediction of actual HRmax. The primary prediction equations used in the health-fitness setting are: APMHR = 220 − age for males; APMHR = 226 − age for females. These equations are based on large sample data with standard deviations of ±11 b·min−1 (Londeree and Moeschberger 1982). A standard deviation can be defined as the average amount by which the scores in a distribution differ from the mean. Therefore, based on the above APMHR equation for men and women and the reported standard deviation for the derived value, the average 20-year-old will have a HRmax between 189 and 211 b·min−1, but could even have a HRmax below 189 b·min−1 or above 211 b·min−1. As such, APMHR can have a considerable amount of error for an individual resulting in even greater error for predicted VO2max. A target HR range for a prescribed training program that is based on a VO2max prediction model using APMHR, could then be either below or above that which provides an optimal overload stimulus for the individual. A target HR range set too low may not provide the individual with an appropriate overload stimulus to achieve health-fitness benefits. A target HR range set too high may not be tolerable for the individual, causing early termination of an exercise session and eventually leading to dropout from the exercise program.
The use of equations that employ APMHR to predict VO2max/peak as a basis for exercise prescription is not appropriate for many individuals with certain clinical conditions. Individuals taking beta-blocker medication to control hypertension exhibit a blunted HR response, especially during aerobic exercise. In such conditions, HRmax would be much lower than that estimated by the APMHR equation. Individuals with pulmonary limitations to exercise, such as chronic obstructive pulmonary disease (COPD) or cystic fibrosis, often terminate exercise because of dyspnea, or shortness of breath, and cannot reach maximal/peak HR levels similar to clinically normal individuals of the same age. Also, individuals with peripheral artery disease (PAD) experience claudication pain in active limbs, even at submaximal exercise intensities. In these patients, exercise is prescribed based on tolerable levels of perceived pain rather than a target HR range. For any clinical conditions where there may be an increased risk for adverse events during exercise, it is important to obtain physician clearance prior to participation in exercise testing or an exercise program. Often, exercise testing with physician supervision may be indicated.
The only way to ensure accuracy when prescribing aerobic exercise based on the expected positive relation between HR and VO2 is to actually measure HRmax and VO2max. This necessitates that the GXT terminates at maximal intensity. However, a maximal GXT may not be possible due to the lack of respiratory-metabolic instrumentation or trained testing personnel. In addition, a maximal GXT may not be appropriate because of time constraints when administering the test protocol and the possible error induced when developing a prediction based on APMHR. VO2 has shown strong correlations with RPE, a fact well-established in experiments demonstrating the concurrent validity of RPE scales. As such, submaximal RPE expressed as a function of either HR or VO2 can be used to predict VO2max/peak. Numerous investigations have shown the validity of RPE-based exercise tests to predict VO2max/peak (Davies et al. 2008; Eston et al. 2005, 2006, 2008, 2012; Faulkner et al. 2007; Faulkner and Eston 2007; Morris et al. 2009, 2010).
Prior to the initiation of a resistance training program for an untrained individual, the administration of 1RM testing may not be indicated because of safety concerns. The statistical basis for traditional models to predict 1RM is the strong inverse relation between weight lifted per repetition and the number of repetitions performed until fatigue. As weight increases, the number of repetitions that can be performed until fatigue decreases, ultimately resulting in the 1RM value. The linear relation between submaximal weight lifted and repetitions performed can be analyzed to predict the amount of weight lifted for 1RM. Weight lifted has shown strong correlations with RPE, a fact well-established in experiments demonstrating the concurrent validity of RPE scales. As such, the relation between resistance and RPE allows the prediction of 1RM. A number of investigations have shown the validity of RPE-based exercise tests to predict 1RM (Eston and Evans 2009; Gearhart et al. 2008; Robertson et al. 2008).
8.1.4 RPE-Based Models to Predict VO2max and 1RM
The development of RPE-based models to predict VO2max and 1RM follow the same design as models based on HR and weight lifted, respectively. Prediction of VO2max using RPE as the predictor variable is based on the positive relation between VO2 and RPE that occurs during load-incremented aerobic exercise. Likewise, prediction of 1RM using RPE as the predictor variable is based on the positive relation between weight lifted and RPE that occurs during load-incremented resistance exercise. These relations and their predictive properties can be presented graphically and/or determined using linear regression analysis. For a graphic prediction procedure, VO2 (or weight lifted) and RPE data points measured at multiple submaximal exercise intensities are plotted on separate axes. A line of best fit that describes the relation between the two variables is drawn by visual determination. When using a HR-based model to predict maximal aerobic power, the point where the line intercepts APMHR is extended laterally to the VO2 axis, identifying predicted VO2max. However, when using a category rating scale, the RPE used to predict VO2max or 1RM is a fixed value, i.e., the maximal RPE (RPEmax) on the category scale. The use of such a fixed upper rating category facilitates the prediction of the corresponding VO2max/1RM value when employing a graphic procedure.
Statistical prediction models that employ RPE as a predictor variable are based on Borg’s Range Model. According to Borg’s Range Model, when an individual reaches maximal intensity of the perceptual response range, they should report the highest numerical category, i.e., an RPEmax of 10 using the OMNI Scale, or 20 using the Borg Scale. Therefore, for visual determination of VO2max using the line of best fit derived from an RPE-based model, the point where the line of best fit intercepts RPEmax is extended laterally to the VO2 axis, identifying predicted VO2max. For visual determination of 1RM using submaximal RPE responses, the point where the line of best fit intercepts RPEmax is extended laterally to the y-axis to identify predicted 1RM. Using linear regression, the average positive relation as depicted by the line of best fit is calculated using submaximal VO2 (or weight lifted) and RPE responses to a load-incremented protocol. For aerobic exercise, this calculation yields an equation, VO2max = s(RPEmax) + i, where the predictor variable is RPE and the criterion variable is VO2. For resistance exercise, 1RM is predicted using the same linear regression model. In these linear regression equations, s is the slope of the line of best fit and i is the y-intercept. Then, one solves for VO2max or 1RM by entering RPEmax into the prediction equation.
8.1.5 Evaluating the Accuracy of RPE-Based Prediction Models
The accuracy of both HR- and RPE-based statistical models to predict VO2max can be evaluated in a laboratory setting where standardized testing instrumentation and control and experimental conditions are available. Using a statistical regression procedure where multiple measurements are required, the subject performs three different submaximal exercise intensities. On a treadmill, the intensities are progressively increased by changing speed and/or grade. On a cycle ergometer, the intensities are progressively increased by incremental changes in PO. The intensities on both a treadmill and cycle ergometer are presented in a load-incremented format. However, there are also less frequently employed protocols that present the different intensities in random order. HR and RPE are measured near the end of each 2–3-min exercise stage.
Using a computer program such as Microsoft Excel, plots of VO2 expressed as a function of HR and as a function of RPE are developed so the relation between variables can be depicted both graphically and using statistical linear regression analysis. VO2max can be predicted by extrapolating submaximal responses to intercept at APMHR or RPEmax using the graphic procedure. In addition, the computer program employs the linear regression equation to determine the line of best fit. The slope and intercept of this regression line can be used to predict VO2max as previously described. Alternatively, some computer programs, such as the Statistical Package for the Social Sciences (SPSS), use a linear regression equation to determine the line of best fit without inclusion of the graphic procedure. In order to compare measured VO2max with that predicted using both the HR and RPE models, the subject should perform an entire GXT terminating at maximal intensity.
For aerobic exercise, both undifferentiated RPE (RPE-O) and the dominant differentiated RPE (i.e., RPE-Legs) have been used in models to predict VO2max/peak (Faulkner and Eston 2007). In some instances, the dominant RPE for a given exercise mode may explain a greater amount of variance in VO2max (i.e., is a better predictor) than the undifferentiated RPE-O. For resistance exercise, only differentiated RPE for the active muscle mass (RPE-AM) has been used in statistical models to predict 1RM (Eston and Evans 2009; Robertson et al. 2008).
8.1.6 Cross-Validation of RPE-Based Prediction Models
From a research perspective, statistical prediction models can be developed for the field setting that do not require actual measurement of VO2. These statistical models must be validated in one sample of subjects then cross-validated in a separate but similar sample of subjects. For the initial validation study, subjects undergo two separate exercise trials: (1) a criterion VO2max test on a treadmill in a laboratory setting; and (2) a submaximal exercise protocol appropriate for administration in the field setting during which RPE are measured. A statistical regression procedure is used to develop an equation to predict criterion measured VO2max using submaximal RPE responses measured during the field test. This equation is used to calculate predicted VO2max for each subject. Criterion measured VO2max is then compared with predicted VO2max. If predicted VO2max is similar to actual measured VO2max (i.e., exhibiting no statistically significant difference), the field-based prediction model is deemed valid.
The statistical model developed and validated in one subject group is then cross-validated by testing the equation on a separate sample of subjects. For such a cross-validation study, the subjects also undergo two separate exercise trials: (1) a criterion VO2max test on a treadmill in a laboratory setting; and (2) the same submaximal exercise field protocol used for the initial validation study, during which RPE are measured. This second, cross-validation sample should have similar characteristics (i.e., age, sex, PA level) as the initial validation sample used to develop the RPE-based prediction equation. Generally, the more specific the population for which the prediction equation is designed, the less error there will be in the predicted VO2max using criterion variables measured under field conditions. Predicted VO2max, calculated using the previously developed equation, is compared with actual measured VO2max using the cross-validation subject sample. If predicted VO2max is similar to actual measured VO2max, then the field-based prediction equation has been successfully cross-validated using an independently selected subject sample. The equation could then be used to predict VO2max for similar individuals in a health-fitness facility where respiratory-metabolic instrumentation is unavailable.
8.1.7 A Perceptually Regulated Exercise Test to Predict VO2peak
Eston and colleagues (2005) developed an alternative type of submaximal exercise test to predict cycle ergometer VO2peak in healthy adults. This exercise is in contrast to commonly used protocols that employ specific increments in power output to produce a systematic increase in exercise intensity. This exercise test is perceptually regulated using step-wise increments in RPE that are produced sequentially to progressively increase exercise intensity. Using the Borg (6–20) Scale, at each 2-min test stage subjects were asked to self-regulate exercise intensity by producing the following RPE’s: 9, 11, 13, 15, and 17. VO2 was recorded near the end of each stage. Linear regression was used to develop equations for the relation between RPE and the corresponding submaximal VO2 response. To predict VO2peak, RPEmax (i.e., Borg Scale RPE of 20) was entered into the regression equations. The perceptually regulated exercise test and subsequent prediction equation d procedures were repeated two additional times to test the effects of protocol familiarity on VO2peak prediction. The results of the investigation found that equations developed using RPE’s 9 through 15 and RPE’s 9 through 17 both predicted VO2peak with reasonable accuracy. However, the equation developed using RPE’s 9 through 17 was more precise, predicting most subjects’ VO2peak within 5–7 ml·kg−1 min−1. In addition, the accuracy of such prediction was improved with practice, evidenced by a closer agreement of predicted and measured VO2peak between trials two and three than between trials one and two (Eston et al. 2005). Subsequent investigations confirmed the ability of perceptually regulated exercise tests to predict VO2max/peak in both active and sedentary adults performing cycle ergometer exercise (Eston et al. 2006, 2008; Faulkner et al. 2007; Morris et al. 2009) and treadmill exercise (Eston et al. 2012; Morris et al. 2010), as well as in able-bodied individuals and paraplegics performing arm ergometery (Al-Rahamneh and Eston 2011).
8.1.8 Submaximal Tests to Assess Training-Induced Fitness Changes
An advantage of exercise tests used to predict VO2max/peak and 1RM is that they can also use submaximal end-points to assess training induced fitness changes. If the same procedures are performed prior to the initiation of an exercise training program and after a designated amount of time participating in the exercise program, pre- and post-training submaximal values can be compared. This can be accomplished using either load-incremented estimation protocols or perceptually regulated protocols. For an estimation protocol, HR and RPE measured during a given workload (i.e., exercise intensity) can be compared before and after training. For a given exercise intensity, decreases in HR reveal a lowered physiological strain to perform the fixed intensity and an improvement in cardiorespiratory fitness. Pre- to post-training decreases in RPE when measured at a given PO (intensity) indicates that the perception of effort associated with a given level of exercise is comparatively lower following a training program. A lower perception of exertion fatigue at a given workload indicates improved tolerance of that exercise intensity and as such an improvement in cardiorespiratory fitness. In addition, this perceptual training adaptation allows the individual to perform the given exercise intensity for a greater amount of time.
This perceptual training adaptation holds true for resistance exercise training programs as well. That is, for a given weight lifted, the perception of exertion decreases from pre- to post-training. A decrease in RPE for a given resistance load indicates improved muscular strength and the ability to perform a lift of that weight for a higher number of repetitions after training. Likewise, for a perceptually regulated aerobic exercise protocol, if a given RPE is produced by self-regulating exercise at a higher workload following training, this decreased perception of exertion indicates an improved exercise tolerance.
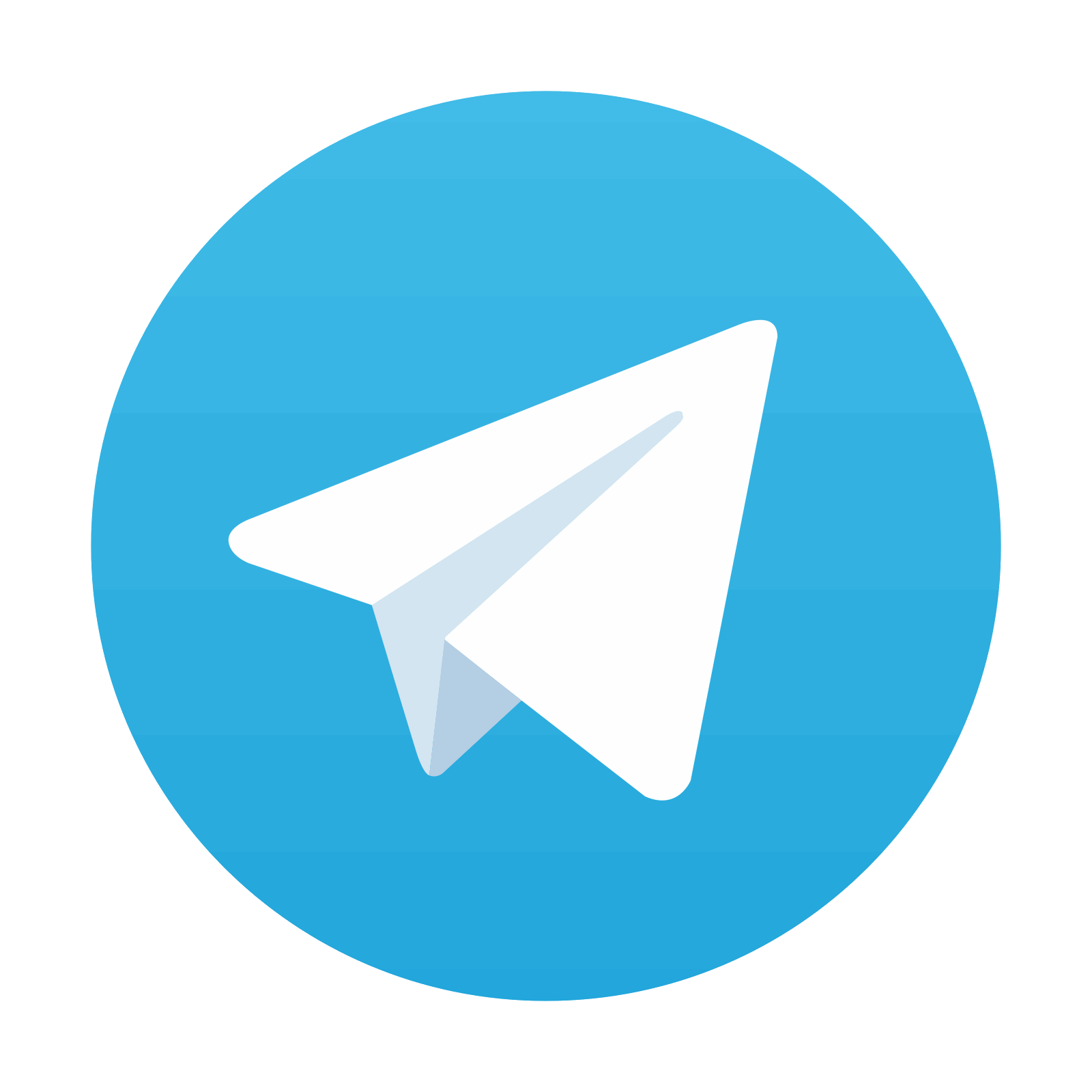
Stay updated, free articles. Join our Telegram channel

Full access? Get Clinical Tree
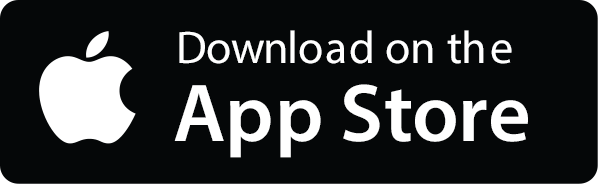
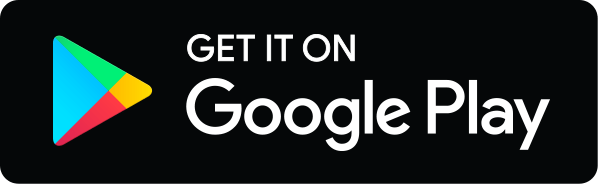