Chapter 4 Jaime Salom Moreno; César Fernández-de-las-Peñas Neurological conditions are chronic, progressive, and lifelong diseases accounting for about 6% of the global burden of diseases and resulting in a substantial effect on healthcare use (World Health Organization, 2006). In fact, the burden of neurological diseases is expected to increase as the population ages (Gaskin et al., 2017; Gooch et al., 2017). Raggi and Leonardi (2015) found that the years lived with a disability and costs of neurological conditions were lower in southern European countries, whereas prevalence was lower in northern European countries, However, no statistical differences were observed. Danila and colleagues (2014) reported that neurological conditions with the highest estimated prevalence in various Canadian settings, including home care, nursing homes, complex continuing care, and psychiatric hospitals, were: Alzheimer disease and related dementias (9%–25%); Parkinson disease (2%–8%); epilepsy (3%–8%); and traumatic brain injury (3%–6%). Additionally, cerebral vascular accidents (Feigin et al., 2017) and multiple sclerosis (Adelman et al., 2013) are important neurological causes of related disability and burden with a prevalence of nearly 5% (Favate & Younger, 2016; Howard et al., 2016). Although stroke has dropped from being the third main leading cause of death to the fourth cause in the United States and Europe (Burke et al., 2012), patients with this disease exhibit higher rates of related disability. This chapter will discuss the presence of spasticity and pain as potential causes of disability in subjects with neurological conditions and their management with needling therapies. One of the main causes leading to physical disability in neurological conditions is the presence of spasticity. The prevalence of spasticity is around 65% in patients with multiple sclerosis (Oreja-Guevara et al., 2013), 65% in patients with spinal cord injury (Holtz et al., 2017), and 40% in patients who had suffered a stroke (Wissel et al., 2013). Not surprisingly, there is a significant correlation between increasing severity of spasticity and worsening of symptoms. Patients with spasticity exhibit lower motor activity performance than patients who do not have spasticity (Sommerfeld et al., 2004; Milinis & Young, 2015); the presence of spasticity, in this case in the lower extremity, is associated with a fourfold increase in direct care costs during the first year poststroke (Lundstrom et al., 2010). Spasticity was originally defined by Lance as ‘a motor disorder characterised by a velocity-dependent increase in tonic stretch reflexes (muscle tone) with exaggerated tendon jerks, resulting from hyperexcitability of the stretch reflex, as a component of upper motor neuron syndrome’ (Lance, 1990). The upper motor neuron syndrome is associated with positive and negative symptoms. Negative signs include weakness and loss of dexterity such as during the acute phase after a stroke. Positive signs are characterised mainly by muscle overactivity such as excessive muscle contractions or other inappropriate muscle activity. Lance’s descriptive definition has been questioned because the term spasticity can also include several other clinical syndromes, including spasms, clonus, and hypertonia. In clinical practice, it is not very common to see spasticity as a primary hypertonic, velocity-dependent increase in tonic stretch reflexes. Burridge and colleagues (2005) proposed that spasticity features ‘disordered sensory-motor control, resulting from an upper motor neuron lesion, and presenting as intermittent or sustained involuntary activation of muscles’, which is a broader and clinically more applicable definition. Current hypotheses of the potential mechanisms of spasticity suggest two main mechanisms: a neural hypothesis involving the cortex, brainstem and spinal cord; and a muscular hypothesis. Potential neural mechanisms include hyperexcitability of the stretch reflex due to an imbalance between excitatory and inhibitory cortical mechanisms on spinal cord circuits (Sheean, 2002) and the release of primitive reflexes or the development of pathological new reflexes; however, these hypotheses are controversial (Sheean, 2002). Not all patients with spasticity exhibit signs of hyperreflexia (Sinkjaer & Magnussen, 1994), which suggests that the neural hypothesis is incomplete by itself. Although the primary lesion is probably neural in origin, there is emerging evidence of secondary changes in the soft tissues such as changes in muscles at the protein, single-fibre, and whole-muscle levels (Foran et al., 2005). Spastic musculature features: decreased mitochondrial volume fraction; an appearance of intracellular amorphous material; a reduction in muscle fibre length; and a decrease in the number of serial sarcomeres (Lieber et al., 2004; Olsson et al., 2006). This suggests that the increased muscle tone in subjects with spasticity could be caused by structural changes in the musculature (Gracies, 2005). The degree to which each proposed mechanism may be responsible for the development of spasticity is not currently known, partially due to the great variability between individuals. In addition to spasticity, pain is one of the most disabling symptoms experienced by subjects with neurological conditions. The prevalence of pain has been estimated to be: 20% to 70% in stroke (Harrison & Field, 2015); 60% to 70% in spinal cord injury (Siddall et al., 2003); 50% to 60% in multiple sclerosis (Foley et al., 2013a); and 30% to 50% in Parkinson disease (Fil et al., 2013). Patients with spasticity after a stroke, with multiple sclerosis, or with a spinal cord injury may suffer from nociceptive and neuropathic pain. In fact, different types of pain can be present at the same time. For example, patients who have suffered a stroke may suffer central poststroke pain, complex regional pain syndrome, and musculoskeletal or spasticity-related pain, among other types of pain (Paolucci et al., 2016a). Management of pain in individuals with spasticity involves both pharmacological and nonpharmacological interventions. In this chapter, the focus is on nociceptive pain in subjects who have suffered a stroke because this condition is the most studied in the context of indications for dry needling. The most common form of nociceptive pain is hemiplegic shoulder pain, which is present in up to 54% of patients who have suffered a stroke (Coskun Benlidayi & Basaran, 2014). Additionally, musculoskeletal pain is observed in approximately 25% of stroke survivors, and a recent study showed an overall prevalence of 29.5% being 14.1% in the acute phase, 42.7% in the subacute, and 31.9% in the chronic phase (Paolucci et al., 2016b). Hemiplegic shoulder pain has been referred to by different diagnostic terms such as tendinitis, rotator-cuff tear, shoulder subluxation, adhesive capsulitis, or spasticity; however, the contribution of these factors is disputed (Lee et al., 2009). It is interesting to note that, although typically pain is located mainly in the shoulder area, it can also involve additional areas of the upper extremity (Dromerick et al., 2008), suggesting the presence of sensitisation mechanisms. Zeilig and colleagues (2013) confirmed that poststroke shoulder pain is likely related to central sensitisation. Poststroke shoulder pain is currently considered a specific subtype of central poststroke syndrome with both nociceptive and neuropathic influences. It is conceivable that needling therapies can address the nociceptive aspects of hemiplegic (poststroke) shoulder pain. Several therapeutic approaches have been proposed for the management of spasticity in patients with neurological conditions, with intramuscular botulinum toxin A (BTX-A) injections among the most popular (Ghasemi et al., 2013) and recognised in clinical practice guidelines for stroke (European Stroke Organization Executive Committee, 2008). BTX-A works primarily by preventing the release of acetylcholine and blocking cholinergic transmission at the neuromuscular junction for a limited period of time of approximately 3 to 4 months (Brown et al., 2014). Different meta-analyses have found that the application of BTX-A in patients who have experienced a stroke is associated with both moderate improvements in upper extremity performance (Foley et al., 2013b) and function and muscle tone in the lower extremity (Wu et al., 2016). In addition, BTX-A is used for treating spasticity-related pain. Although the underlying mechanisms are still unclear, some hypothesis are proposed, including: blocking nociceptor transduction, reduction of neurogenic inflammation by inhibiting neural substances and neurotransmitters such as substance P, calcitonin gene-related peptide, and glutamate; and prevention of peripheral and central sensitisation (Durham et al., 2004; Bach-Rojecky et al., 2010; Paterson et al., 2014). The review by Baker and Pereira (2013) found that the use of BTX-A was effective for upper and lower extremity spasticity, but its effects on spasticity-related pain were rather limited. A more recent meta-analysis found that BTX-A resulted in small to moderate pain relief and in an increase in range of motion in patients with chronic hemiplegic shoulder pain (Wu et al., 2015). The most common targeted muscles for treatment of hemiplegic shoulder pain included the pectoralis major, teres major, subscapularis, biceps brachii, and infraspinatus muscles (Yelnik et al., 2007; Marciniak et al., 2012). Currently, the dosages of BTX-A vary considerably in clinical practice and are frequently based on the personal experience of the practitioner, expert opinions, the formulation and brand of the type of BTX-A, and the individual patient’s response (Yablon et al., 2011). Yablon and colleagues reported the estimated doses of BTX-A to produce a mean one-point decrease in the Ashworth scale for the flexor carpi radialis (22.5U), the flexor carpi ulnaris (18.4U), the flexor digitorum superficialis (66.3U), and the flexor digitorum profundus (42.5U) muscles. Significant drawbacks of BTX-A therapy are its high cost, the transient nature of the toxin, and the possibility, although very rare, of the development of neurotoxicity (Intiso et al., 2015). Finally, it is important to consider that BTX-A should not be clinically applied as an isolated intervention. Kinnear and associates (2014) found that therapy combined with BTX-A was slightly more effective than BTX-A alone. In fact, several rehabilitation interventions are applied in combination with BTX-A: ergometer cycling, electrical stimulation, stretching exercises, constraint-induced movement therapy, task-specific motor training, and exercise programs (Kinnear et al., 2014). Considering the disadvantages of BTX-A injections, needling therapy with solid filament needles may be a reasonable and worthy alternative. Several studies have investigated the effects of acupuncture on poststroke spasticity; however, the results are conflicting. Some observed that acupuncture was effective for reducing spasticity (Mukherjee et al., 2007; Zhao et al., 2009), but others did not find any significant effect (Fink et al., 2004; Wayne et al., 2005). A recent meta-analysis showed that acupuncture or electroacupuncture was associated with a decrease in wrist, knee, and elbow spasticity in patients after stroke, although a subgroup analysis did not reveal significance (Lim et al., 2015). A review of 24 systematic reviews suggested that acupuncture can be effective for stroke rehabilitation and stroke related disorders (Zhang et al., 2014); another review concluded that acupuncture combined with exercise was effective for shoulder pain after stroke (Lee et al., 2012). Discrepancies between published studies appear to exist because of different methodologies and point selection. Although in most acupuncture studies, classical acupuncture points were the target, in other studies the needle was directed to the region of spastic muscles or entirely outside the affected area. In the latter scenario, the needle placement was therefore remote from the spastic musculature. Dry needling with the needle placed directly into the spastic muscles may offer an alternative intervention for the spastic musculature. Evidence for the effects of dry needling in neurological conditions is slowly emerging (Uttam, 2015). Two recent case reports discussed dry needling for poststroke spasticity of the upper extremity (Ansari et al., 2015) and for quadriparesis of a 4-year-old child (Gallego & del Moral, 2007), respectively. In the first case report, Ansari and associates applied dry needling to the pronator teres, the flexor carpi radialis, and the flexor carpi ulnaris muscles. In the second report, Gallego and del Moral applied dry needling to the opponens pollicis, the flexor carpi radialis, the flexor digitorum superficialis, and the biceps brachii muscles. A recent case series that included 29 patients with poststroke spasticity observed an improvement of spasticity, alpha motor neuron excitability, range of motion, and hand dexterity 1 hour after the application of a single session of dry needling into the wrist flexor muscles (Fakari et al., 2017). Mendigutia-Gómez and colleagues (2016) conducted the only clinical trial to date investigating changes in spasticity in the upper extremity with dry needling, which included a control group. In this study, patients with poststroke spasticity received three sessions of dry needling in the shoulder girdle muscles combined with a multimodal rehabilitation program. The results showed significant improvements in shoulder range of motion, pressure pain thresholds, and spasticity in the external rotator muscles such as the infraspinatus muscle (Mendigutia-Gómez et al., 2016). There is only one study investigating the effects of dry needling in hemiplegic shoulder pain. The study by DiLorenzo and associates (2004) found that inclusion of dry needling into a rehabilitation program was effective for improving hemiparetic shoulder pain. In this study, patients experienced pain in the anterior and lateral parts of the shoulder area, the deltoid and rotator cuff muscles, and the lateral aspect of the upper extremity. This pain pattern resembles the referred pain from TrPs in the shoulder girdle muscles (Simons et al., 1999). The supraspinatus, infraspinatus, anterior deltoid, middle deltoid, and levator scapulae were the most painful muscles with the dry needling intervention. Dry needling was effective for reducing severe pain during sleep and providing more comfort overall in the wheelchairs, in bed, and during their physiotherapy treatment (DiLorenzo et al., 2004). Subjects receiving dry needling reduced their pain medicine intake (DiLorenzo et al., 2004). Evidence for the application of dry needling in the lower extremity is similar to the upper limb. A single case study reported that dry needling was able to decrease local muscle stiffness assessed by tensiomyography in a patient with chronic poststroke spasticity (Calvo et al., 2016). In this report, the treated muscles included the biceps and triceps brachii, rectus femoris, semitendinous, biceps femoris, and medial and lateral gastrocnemius (Calvo et al., 2016). The only randomised clinical trial investigating the immediate effects of dry needling on spasticity in the lower extremity found a decrease in spasticity in the ankle plantar-flexor muscles and improvements in pressure pain sensitivity in individuals with poststroke spasticity (Salom-Moreno et al., 2014). In this clinical trial, agonist (i.e., gastrocnemius) and antagonist (i.e., tibialis anterior) muscles received a single session of dry needling (Salom-Moreno et al., 2014). There were significant improvements in support surface of the affected and unaffected feet, which suggest that dry needling may improve gait parameters in poststroke subjects (Salom-Moreno et al., 2014). A recent case study report including two poststroke patients reported specific electroencephalographic changes after dry needling of TrPs. The first subject was a 51-year-old male with an ischaemic stroke affecting the left side. The researchers used dry needling for TrPs within the pronator teres, medial and lateral gastrocnemius, soleus, and peroneus longus muscles. The second subject was a 56-year-old male with a haemorrhagic stroke affecting the right side, who received dry needling targeting TrPs in the pectoralis major, biceps brachii, brachialis, latissimus dorsi, teres major, pronator teres, flexor digitorum superficialis and profundus, adductor pollicis, medial and lateral gastrocnemius, soleus, semitendinosus, semimembranosus, and the long head of biceps femoris muscles. The researchers observed an improvement in the regional brain activity, especially in alpha waves, and changes in the cordance of the frontal/prefrontal regions, using quantitative electroencephalographic activity and electroencephalographic cordance. This is the first demonstration that the results of peripherally applied dry needling can be objectively assessed with electroencephalographic outcome measures. In conclusion, dry needling is an emerging therapeutic intervention for the management of spasticity in patients with neurological conditions; however, current evidence has only investigated the short-term effects and has focused mainly on patients who suffered a stroke. Several questions about the use of repetitive treatments, appropriate dosage, the combination with other pharmacological and nonpharmacological agents the combination with other physical therapy approaches, and the longer-term duration of the effects of dry needling remain to be elucidated. The application of dry needling in spastic muscles is based on the similarities between structural changes observed in spastic muscles, changes observed in muscles with myofascial trigger points (TrPs), and the similarities between the neurophysiological mechanisms of dry needling and BTX-A injections. There is clear evidence that muscle contractures secondary to spasticity are due to a reduction in muscle fibre length, an increase in the number of cross bridges, an increase in collagen tissue, and an increase in active muscle stiffness. In fact, the muscle fibres from patients with spasticity are more than twice as stiff as the fibres from patients without spasticity (Fridén & Lieber, 2003). It is interesting to note that current pathophysiological theories explaining the formation of TrPs also involve a reduction of muscle fibres (Bron & Dommerholt, 2012) and an increase in stiffness in the taut band, which involves the TrP (Chen et al., 2016) (see Chapter 1). In addition, TrPs can also contribute to movement alterations observed in patients with neurological conditions because they promote accelerated fatigue, altered patterns of intramuscular activity, an increase antagonist coactivation, and altered muscle activation patterns (Lucas et al., 2004; Ibarra et al., 2011; Ge et al., 2012, 2014; Bohlooli et al., 2016). Both dry needling and BTX-A interventions target dysfunctional motor end-plate zones. In an animal model, Domingo and associates (2013) observed that the application of dry needling induces a destruction of the neuromuscular junction, including damage of the axonal area. On the other hand, BTX-A blocks the release of acetylcholine at the neuromuscular endplate, and BTX-A injections are also used in the management of myofascial pain syndrome (Zhou & Wang, 2014). A recent Cochrane review concluded that BTX-A injections were only effective for patients with epicondylalgia (Soares et al., 2014). The efficacy of BTX-A injections for myofascial pain is not entirely clear, which may be due to a wide variety of factors such as an incomplete treatment of a regional myofascial pain syndrome, inappropriate or confounding control populations or treatments, inappropriate time periods for assessment of outcomes, and a misinterpretation of the timeframe of action of BTX-A (Gerwin, 2012). The presence of pain is another clinical application of dry needling for patients with neurological conditions. De Oliveira and colleagues (2012) reported that burning was the most common descriptor used by 70% of patients for describing postcentral stroke pain. In this sample of patients, active TrPs reproducing their symptoms were found in up to 65% of the sample, especially in the upper trapezius (50%), splenius (30%), supraspinatus (30%), semispinalis (25%), and infraspinatus (20%) muscles (De Oliveira et al., 2012). In this scenario, active TrPs can serve as peripheral generators of nociceptive input that may alter pain perception in a subgroup of patients with stroke. At the same time, spasticity and altered central descending pain modulation observed in poststroke patients (Roosink et al., 2011) may induce overload to the affected muscles and contribute to the development of TrPs. It is conceivable that a vicious cycle may exist between the development of TrPs, spasticity, and sensitisation. It is important to consider whether a clinician aims to reduce spasticity or pain as the main objective of dry needling with patients with neurological conditions. If the objective is to reduce spasticity or muscle tone, patients with spasticity but without pain may not experience the phenomenon of referred pain that is observed commonly in patients suffering from musculoskeletal pain due to sensory disturbances. For these cases, the clinical reasoning process for the application of dry needling should not be based on the same diagnostic criteria as used in patients with musculoskeletal pain. Of interest is whether the term ‘TrP dry needling’ should be used in individuals with spasticity, as many patients do not exhibit any sensory symptom usually associated with myofascial pain. Because spasticity is related to an increase in muscle tone, perhaps the mere presence of a taut band and hypersensitive spots in the spastic muscle would be more relevant clinical criteria. In patients showing sensory disturbances without being able to clearly discriminate the most sensitive spot, the clinician may need to rely on palpation and locate the tightest spot within a spastic contracture, which would be similar to locating active TrPs in individuals without spasticity (Turo et al., 2013). The identification of taut bands in a spastic muscle is usually more difficult than in a nonspastic muscle and requires excellent palpatory skills. Another reason to consider dry needling clinically is to improve the commonly observed resistance to passive movement. To address this clinical issue, clinicians must understand the specific spastic pattern of each individual patient. Once the individual pattern has been determined, therapists must decide which muscles will be included in the actual dry needling treatment. Based on clinical experience, we recommend this cluster of clinical diagnostic criteria: For patients with primary complaints of spasticity-related local and referred pain, who recognise elicited sensory (pain) symptoms, the more standard criteria for myofascial pain would apply. When needling patients with neurological conditions, the clinical criteria and the actual needling procedures differ from needling patients with just musculoskeletal pain.
Dry Needling for Neurological Conditions
Introduction
Spasticity and muscle tone
Pain in neurological conditions
Botulinum toxin a for spasticity and related pain
Dry needling for neurological conditions
Clinical reasoning for dry needling in neurological conditions
Clinical guidelines for dry needling in spasticity
Diagnostic Clinical Decision
Dry Needling Procedure
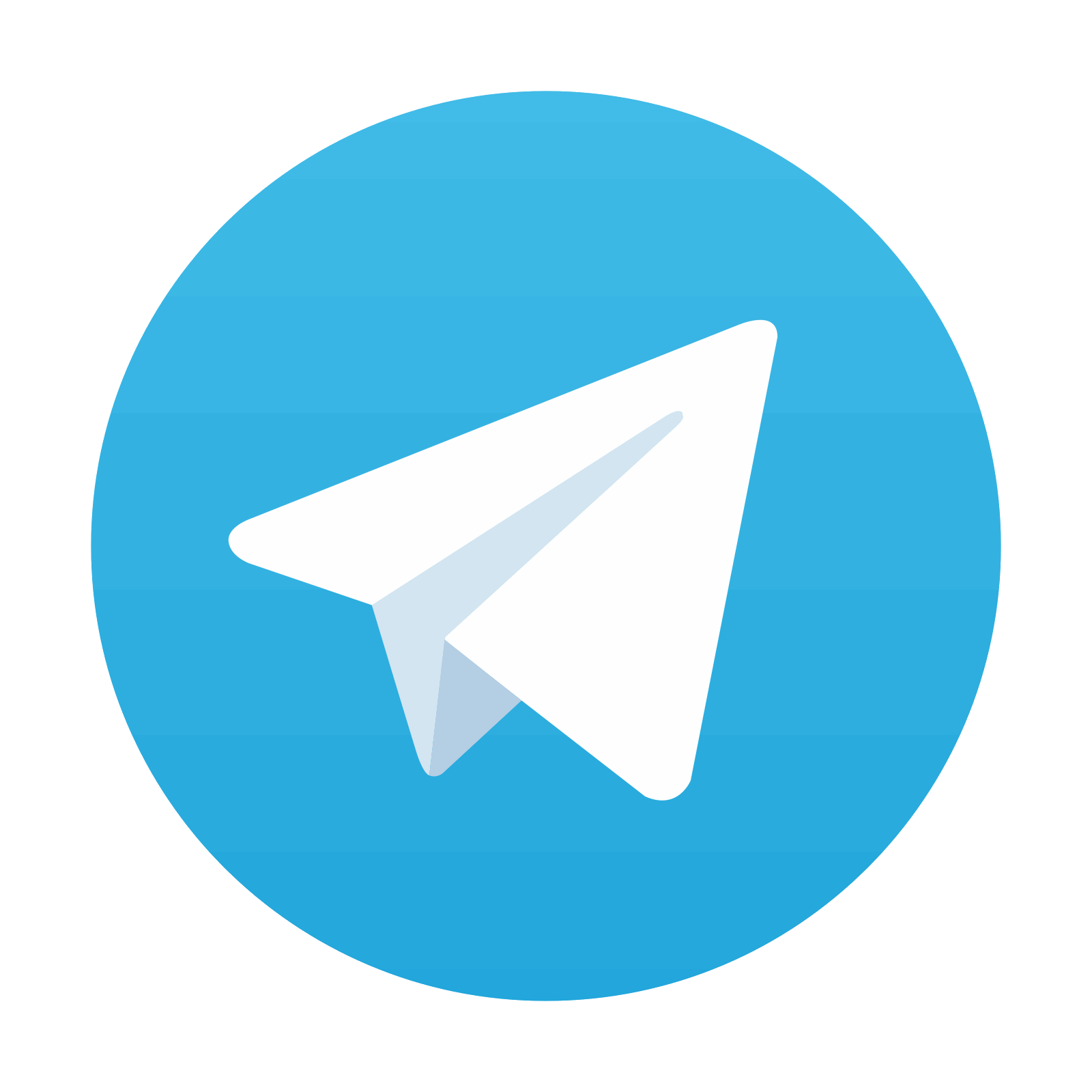
Stay updated, free articles. Join our Telegram channel

Full access? Get Clinical Tree
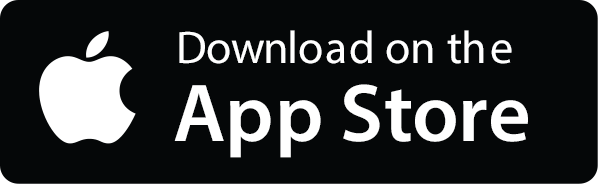
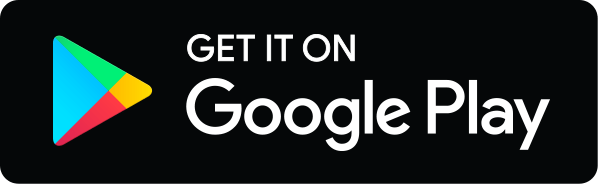